Chapter 67 Diabetic Ketoacidosis
• Diabetic ketoacidosis (DKA) is a severe form of complicated diabetes mellitus that requires emergency care.
• Fluid therapy and correction of electrolyte abnormalities are the two most important components of therapy.
• Concurrent disease increases the risk for DKA and must be addressed as part of the diagnostic and therapeutic plan.
• About 70% of treated dogs and cats are discharged from the hospital after 5 to 6 days of hospitalization.
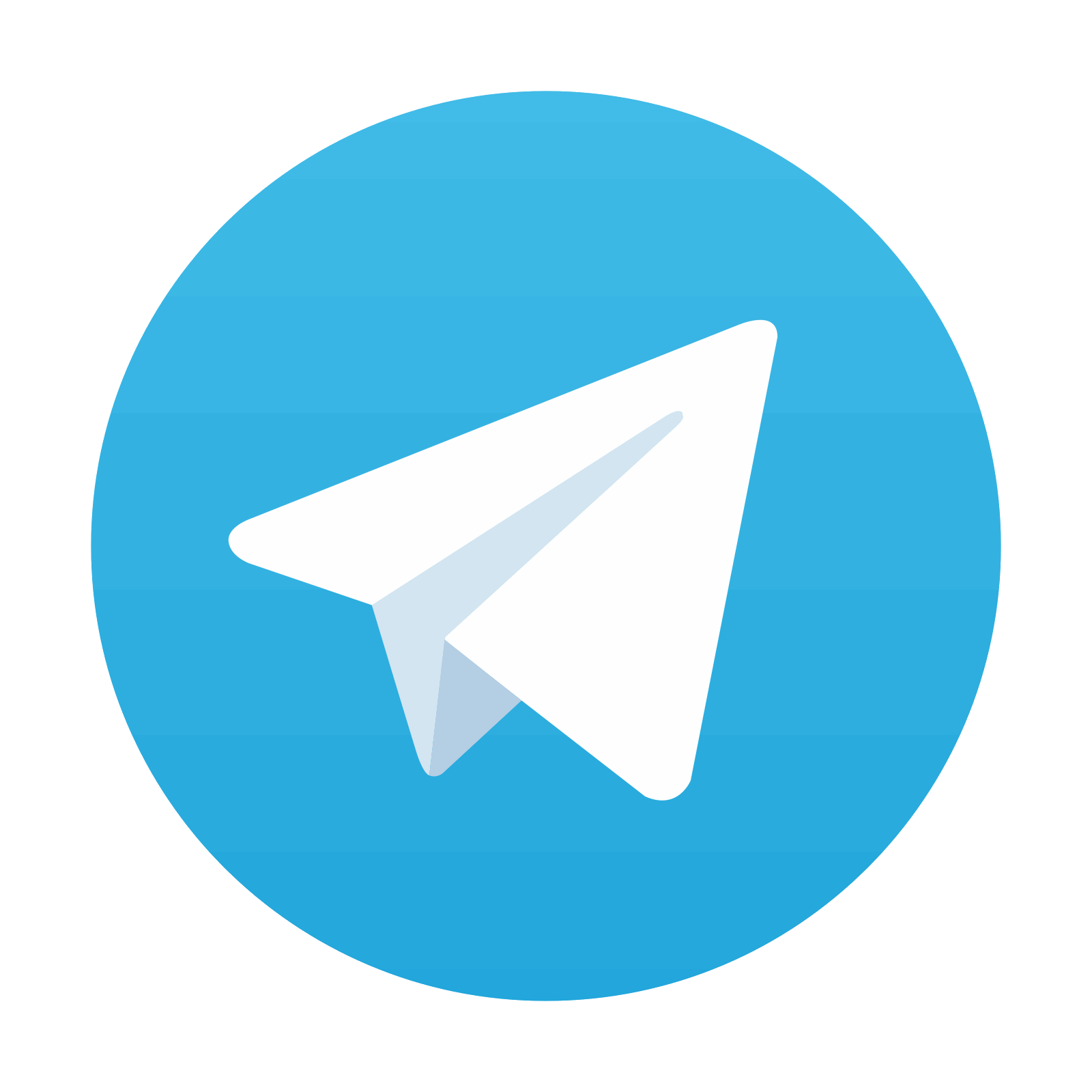
Stay updated, free articles. Join our Telegram channel

Full access? Get Clinical Tree
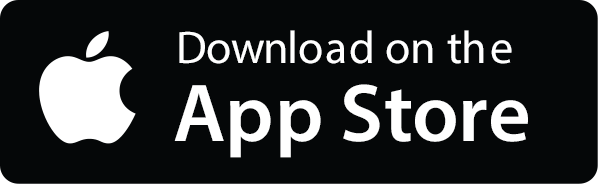
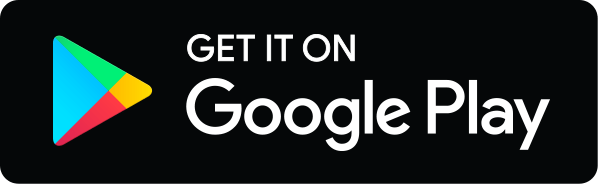