47 Jim E. Riviere and Rosanna Marsella A large number of cases seen in the everyday practice of small- and large-animal veterinary medicine involve lesions of the skin or its appendages. This chapter reviews the salient features of the general anatomy, histology, and biochemistry of skin relevant to the treatment of dermatological disease; acquaints the practitioner with the features of percutaneous drug absorption relevant to the treatment of skin disease; and discusses the categories of pharmacological preparations available on the veterinary market to treat skin diseases in domestic animals. The specific drugs used to treat skin diseases are well covered in other chapters of this text. Chapters that should be consulted for application to skin disease include those on antimicrobials, analgesics, and antiinflammatory drugs; antipruretics and antihistamines; glucocorticoids; immunosuppressives (cyclosporine); and ectoparasiticides. Only in the last few decades have scientists begun to understand how the skin functions in normal and diseased states, to understand the skin’s barrier function in terms of water loss and drug delivery, and that attempts have been made to improve the delivery of pharmaceutical agents through the skin by temporarily adjusting that barrier to deliver the drug. In order to successfully minimize the skin’s ability to block the absorption of drugs to treat dermatological disease in domestic animals, it is imperative to understand the normal functional anatomy and biochemistry of the skin. In veterinary medicine, transdermal delivery is widely employed when pesticides are applied monthly to a single area of skin for the control of fleas and ticks over the entire body. Transdermal fentanyl patches are also widely used for postsurgical analgesia. This topical port of drug delivery will see increased use for other therapeutic indications. However, most drugs applied to the skin are not intended to be absorbed systemically but are used to elicit a local therapeutic effect in the treated skin. The characteristic that separates dermatological therapy from other components of veterinary pharmacology is this use of topical dosage formulations to treat surface conditions or to target the underlying skin. The drugs are not different from those used in other diseases, but they are different in the vehicles employed and mode of administration. A full understanding of the biological factors that modulate absorption and of the pharmaceutical composition of dermatological formulations (e.g., vehicles) is essential to a proper understanding of dermatopharmacology. The same principles also apply to the absorption of topical transdermal preparations such as pour-on ectoparasiticides and analgesics such as transdermal fentanyl gels or patches. It must be stressed that the drugs incorporated into these formulations are the same as those used to treat diseases of other systems and thus, their descriptions will not be repeated in this chapter. The skin is the largest organ of the body, with the integument accounting for 24% of the overall body weight in the puppy and 12% in the adult dog (Pavletic, 1991). The skin has the function of protecting the internal organs of the body from extremes in temperature fluctuations, allergens, pollutants, toxic chemicals, and organisms such as bacteria, fungi, parasites, and viruses found ubiquitously in the environment. The skin of domestic animals is quite similar in gross and histological morphology across species lines and is usually thickest over the head, dorsum of the neck, back, and sacrum and on the plantar and palmar surfaces of the feet. It is thinner on the ventral abdomen, the medial surfaces of the limbs, and the inner pinnae, and thinnest over the scrotum of male animals and the earlobe of the human. Perforating the skin are several types of appendages (depending on the species), such as hair follicles, sebaceous and sweat glands, spines, quills, scales, spurs, horns, claws, nails, and hooves (Montagna, 1967). The specific anatomy of skin and hair has been reviewed extensively elsewhere (Monteiro-Riviere, 2006; Blackburn, 1965; Lloyd et al., 1979a, 1979b; Sar and Calhoun, 1966; Kozlowski and Calhoun, 1969; Strickland and Calhoun, 1963; Talukdar et al., 1972; Pavletic, 1991; Montagna, 1967; Amakiri, 1973). On the histological level, the skin can be divided into two distinct units: the epidermis and the dermis. The epidermis consists of stratified squamous keratinized epithelium that undergoes a programmed proliferation and differentiation that will eventually result in the formation of the major barrier to drug penetration: the stratum corneum. Two primary cell types exist in the epidermis: those of keratinocyte origin and those of nonkeratinocyte origin. Five distinct layers of keratinocytes can be present in the epidermis, as shown in Figure 47.1. Listed from the deepest layer of the epidermis to the most superficial, they are (i) stratum basale (basal layer), (ii) stratum spinosum (prickle layer), (iii) stratum granulosum (granular layer), (iv) stratum lucidum (clear layer), and (v) the stratum corneum (horny layer). Each cell layer has its point of origin at the stratum basale. The stratum basale is a single layer of cuboidal or columnar cells that rest on the basal lamina. These cells are attached to the basal lamina by hemidesmasomes, and to each other and to the cells of the stratum spinosum by desmasomes. The stratum basale cells continuously divide, with some remaining as basal cells and others beginning to move more superficially and mature by changing their intracellular content through the process called keratinization. This viable and dividing epidermal cell layer is the target for transformation into cancerous cells. Figure 47.1 A schematic view of the epidermis and dermis of the skin. Source: Monteiro-Riviere, 1991. Reproduced with permission of Taylor & Francis Group LLC. The next more superficial layer next to the stratum basale, the stratum spinosum, is composed of irregularly shaped polyhedral cells that make up much of the bulk thickness of the epidermis. The next layer composes the stratum granulosum, which consists of several layers of cells that begin to flatten horizontally. Of primary interest are the lamellated granules within these cells, which contain polar phospholipids, such as glycosphingolipids and free steroids, and numerous hydrolytic enzymes, including acid phosphatase, proteases, lipases, and glycosidases. As these intracellular products accumulate, these cells will exocytose their intracellular products and fill in the intercellular spaces, eventually forming the extracellular lipid matrix of the stratum corneum that forms the penetration barrier of the stratum corneum. As these epidermal cells continue their migration, they form the stratum lucidum, a translucent line of cells found only in areas having very thick skin, such as plantar and palmar surfaces (footpads) and the planum nasale. These cells are translucent because both nuclei and cytoplasmic organelles are missing (Monteiro-Riviere, 1991, 2006; Idsen, 1975; Montagna, 1967). The stratum corneum is the final and most superficial layer of the epidermis and is its most important when considering the feasibility of topical drug therapy since it is the primary barrier to percutaneous absorption. In addition to the barrier function for xenobiotics trying to enter the body from the environment, the stratum corneum also provides a barrier to insensible water loss, an evolutionary adaptation that allows terrestrial animals to exist in a nonaquatic environment. In fact, most veterinary dermatological vehicles are targeted at this action. This layer consists of several dead layers of cells, organized into vertical columns in a tetrakaidecahedral (14-sided) configuration, the thickness of which varies depending on location (Monteiro-Riviere, 1991). This particular cell shape provides a minimal surface-to-volume ratio and also minimizes systemic water loss through the skin (transepidermal water loss). Each cell is ingrained in the lipid matrix produced by the lamellated granules when the cells were still in the stratum granulosum layer. These dead cells are also surrounded by a thick plasma membrane with a submembranous layer of involucrin, also produced earlier in development. With intracellular and intercellular barriers firmly in place, the stratum corneum has the ability to constrain the passage of unwanted chemicals and toxins from the environment. Unfortunately, the stratum corneum does not discriminate between these unwanted substances and the pharmaceuticals the veterinarian may wish to penetrate the skin for topical drug therapy for the treatment of dermatological disease. Melanocytes are cells located in the basal layer of the epidermis and contain dark cytoplasmic granules called melanosomes. These cells impart color to the skin, the color and intensity determined by the number, size, distribution, and degree of melanization of the melanosomes. Merkel cells are also located in the basal region of the epidermis and are thought to function as slow-adapting mechanoreceptors for touch. Langerhans cells are located in the stratum spinosum but can also be present in dermal lymph vessels, lymph nodes, and dermis. The Langerhans cells’ primary function is to present antigen to lymphocytes; they may also be the initial receptors for cutaneous immune responses. The dermis is composed of connective tissue, consisting of collagen, elastin, and reticular fibers dispersed in an amorphous ground substance, and can be divided into two rather poorly demarcated areas. The papillary layer consists of loose connective tissue and connects the epidermis (stratum basale/basal lamina) to the deeper reticular layer of the dermis. The reticular layer consists of dense connective tissue connected to the hypodermis, which is composed mostly of fat. Dispersed throughout both layers of the dermis is a network of arterial and venous blood vessels needed to nourish the cells of the epidermis and dermis as well as take part in the last stages of the percutaneous absorption of compounds. Lymph vessels, nerves, apocrine and eccrine sweat glands, sebaceous glands, Pacinian (pressoreceptor), Meissner’s (touch receptor), and Ruffini (mechanical receptor) corpuscles, hair follicles, and smooth muscles (arrector pili) are the other major structures found in the dermis. Two types of arteries, musculocutaneous and direct cutaneous, supply the needed nutrients to the epidermis. Direct cutaneous arteries run parallel to the skin, directly supplying the skin with blood, while musculocutaneous arteries supply both the skin and underlying musculature and run perpendicular to the skin. Cutaneous blood supply by each type of artery varies with species and location. Cutaneous blood flow rates may be one of the factors affecting the passive percutaneous absorption of chemicals. Table 47.1 clearly demonstrates this by comparing laser Doppler cutaneous blood flow parameters in nine species of domestic animals (Monteiro-Riviere et al., 1990). Table 47.1 Cutaneous blood flow measurements in nine species of domestic animals. Source: Adapted from Monteiro-Riviere et al., 1990. Values are ml/min/100 g tissue, ± standard error of the mean. NA, data not available. As a general rule, skin structure and function are similar across species lines. However, some minor differences are apparent. Avian integument possesses the most profound differences in skin morphology from other domestic species. The four layers of the epidermis are, from the deepest to the most superficial, the stratum basale, the stratum intermedium (stratum spinosum), the stratum transitivum (stratum granulosum), and the stratum corneum (stratum germinativum). Unlike mammals, avians possess no skin glands (Monteiro-Riviere, 2006). The skin of aquatic mammals has a very thick stratum corneum resembling parakeratosis and there is no stratum granulosum (Montagna, 1967). Pig skin is similar histologically to human skin (Monteiro-Riviere and Stromberg, 1985) and has been used experimentally to reliably predict the percutaneous absorption of chemicals in humans (Riviere, 2006). With respect to cutaneous circulation, musculocutaneous arteries are the primary vascular supply to the skin of humans, apes, and swine. Loose-skinned animals (canines and felines) lack musculocutaneous arteries; all vessels involved in cutaneous circulation travel parallel to the skin (Pavletic, 1991). Studies of piroxicam (a nonsteroidal antiinflammatory drug) in pigs suggest that the type of cutaneous circulation may affect the local tissue concentrations of topically applied drug (Monteiro-Riviere et al., 1993). Little work has been done directly comparing the absorption of drugs and topical pesticides across veterinary species (Riviere and Papich, 2001). The skin, specifically the epidermis, is mostly an anaerobic organ. The absence of capillaries directly feeding oxygen to the epidermal cells makes the epidermal cells relatively oxygen poor in the normal state when compared to other tissues with a more direct blood supply. Due to this low oxygen tension, the epidermis produces 70–80% of its total energy requirements (adenosine triphosphate) through the anaerobic metabolic pathway (glycolysis), with lactic acid being the end product of glucose utilization. The epidermal cells, the most active of which are those in the single layer of the stratum basale, are the primary cells involved in this energy production, with the lactic acid end product passively diffusing into the dermis and then into the blood vasculature, eventually recycled by the liver back into glucose. Although the glycolytic pathway produces most of the energy requirements of the epidermis, other energy pathways (tricarboxylic acid cycle and pentose phosphate shunt) are also utilized to lesser degrees in some phases of epidermal growth (Freinkel, 1983). Some topically applied drugs may have an effect on energy production pathways within the epidermis. One study (Spoo et al., 1993) showed that in vitro weanling porcine skin flaps had large increases in epidermal cell glucose utilization when the skin was dosed with benzoyl peroxide. In addition to drugs, vehicles (discussed in more detail in Section Topical Vehicles) may also have similar effects. The stratum corneum is the primary line of defense to prevent percutaneous absorption of drugs. However, any drug passing through the stratum corneum may face a metabolic, rather than a physical, barrier. The skin has a remarkable ability to metabolize xenobiotics. These metabolic reactions consist mainly of oxidation, reduction, hydrolysis, and the phase I and II conjugation reactions. Enzymes present within the extracellular lipid matrix include acid lipase, phospholipase A, sphingomyelinase, glycosidases, acid phosphatases, cathepsins, and carboxypeptidases (Elias, 1992). Some of these reactions are major pathways for the metabolism of topical steroids as well as other drugs such as norepinephrine, benzoyl peroxide, and benzo(a)pyrene. Skin has been shown to metabolize organophosphate parasiticides. When parathion is topically applied to the skin and penetration through the stratum corneum occurs, it undergoes significant metabolism within the epidermis to the bioactive metabolite paraoxon and/or p-nitro phenol, both of which will enter the systemic circulation to possibly affect other organ systems (Riviere and Chang, 1992). Additional studies have demonstrated the metabolic capabilities of skin when topically exposed to caffeine, testosterone, butylated hydroxytoluene, salicylic acid, norepinephrine, benzo(a)pyrene, and benzoyl peroxide – to name only a few – in many species of laboratory animals, as well as in human skin. Benzoyl peroxide, a popular veterinary drug used as a keratolytic and degreasing agent, is metabolized almost 100% to benzoic acid within the epidermis. In addition, the cytochrome P450 enzyme system, most commonly associated with the liver, is present and is inducible in skin (depending on the compound that was applied topically) and is the pathway that is responsible for the conversion of parathion into paraoxon in porcine skin (Riviere and Chang, 1992; Mukhtar, 1992; Bashir and Maibach, 2005). Glutathione-S-transferase, aryl hydrocarbon hydroxylase, and 7-ethoxycoumarin have also been demonstrated to exist in rat and mice epidermal cell homogenates (Raza et al., 1992). These studies all show that the skin has formidable metabolic functions as well as the traditional barrier functions. In addition to its metabolic activities, skin also has a marked ability to synthesize lipid, which is used to construct the extracellular epidermal barrier. Epidermal cells manufacture a variety of neutral lipids, ceramides, glycosylceramides, gangliosides, sterol esters, fatty acids, alkanes, and phospholipids, which are largely found in the extracellular barrier of the stratum corneum. Some of these lipids tend to be site specific, with phospholipids and sterols residing mostly in the basal cell layers and some sterols and neutral lipids occupying the upper regions of the epidermis, mainly the stratum corneum. The effects on percutaneous absorption by the intercellular lipid structure have been discussed in greater detail elsewhere (Wertz, 1992; Potts and Francoeur, 1992; Hadgraft et al., 1992; Swartzendruber, 1992; Elias and Feingold, 1992; Monteiro-Riviere et al., 2001). It is now accepted that this complex intercellular lipid matrix provides the primary barrier to drug penetration. It is important to realize that in some skin disease states, the overall production of lipids by the epidermal cells may be altered due to altered intracellular metabolism, subsequently followed by some alterations in the percutaneous absorption patterns of many drugs. Alterations in the epidermal cells’ plasma membrane (primarily composed of lipid) – whether due directly to a drug(s), by the vehicle a drug was delivered in, or due to a disease process – may incite inflammatory reactions due to the release of inflammatory mediators (eicosanoids) from the epidermal cells or from the underlying cutaneous vasculature. Eicosanoids are a group of biologically active compounds derived enzymatically from eicosatetraenoic acid (arachidonic acid). Prostaglandins, leukotrienes, thromboxanes, and hydroxyeicosatetraenoic acids can be produced in minute quantities from arachidonic acid and have powerful proinflammatory effects on skin and the surrounding tissues. Eicosanoids are found in nearly every tissue in the mammalian body (Spannhake et al., 1981; Dunn and Hood, 1977; Goldyne, 1986), with some cells specializing in a certain type of eicosanoid. Skin produces several prostaglandins, including PGE2, PGF2α, and PGI2, as well as the lipoxygenase product leukotriene B4. PGE2 induces vasodilation by elevating cyclic adenosine monophosphate in vascular smooth muscle cells, while PGF2α causes vasoconstriction of the cutaneous vasculature by elevating cyclic guanosine monophosphate levels in vascular smooth muscle cells. In contrast to PGE2, increased PGF2α concentrations in skin enhance the leukocytes’ response to chemotactic stimuli. PGF2α has many effects on organs other than the skin, most notably in the reproductive tract of both humans and domestic animals. PGI2 is a potent vasodilator produced mainly by vascular endothelial cells; it also inhibits vascular platelet aggregation. PGI2 can be released from skin in response to some vehicles – namely, alcohols such as methanol, ethanol, and 2-propanol (Landolfi and Steiner, 1984; Karanian et al., 1985). Cutaneous vasodilation has been observed in humans orally consuming alcohol (ethanol), presumably due to release of PGI2 from vascular endothelial cells in contact with the alcohol-containing blood (Landolfi and Steiner, 1984). Leukotriene B4 (LTB4) is an inflammatory mediator produced from arachidonic acid via 5-lipoxygenase enzyme activity (Ford-Hutchinson, 1985). As with PGF2α, LTB4 is a potent chemotactic agent (Paulissen et al., 1990; Van de Kerkhof et al., 1991) and may also be a potent vasodilator and cause significant increases in vascular permeability when coadministered with PGE2 (Ford-Hutchinson, 1985). Although LTB4 is most commonly produced by leukocytes, skin contains some inherent 5-lipoxygenase activity as determined from studies using stimulated human keratinocytes in culture as well as human and murine epidermal homogenates (Ruzicka, 1990). These inflammatory mediator-induced changes in cutaneous blood flow may impact on the absorption and cutaneous distribution of topically applied drugs. The primary protein product of the skin is keratin, which is the main intracellular component of the stratum corneum. In tandem with extracellular lipid, keratin forms the major component of the epidermal barrier. Keratin primarily consists of cystine, serine, glutamic acid, arginine, aspartic acid, and glycine amino acid residues. Of significance within the keratin molecule structure is the intrachain cystine disulfide bridging that further strengthens the overall keratin macrostructure. Keratin is the major protein foundation of hair (Monteiro-Riviere, 1991, 2006). Prior to the 20th century, many scientists believed that skin was an impermeable barrier to all substances, except possibly gases. The science of dermatology has since advanced to reveal that many substances can enter the systemic circulation via the skin. Four pathways by which substances can gain this access to the rest of the body have been postulated and are schematically shown in Figure 47.2. Figure 47.2 A schematic view of the skin and the four routes of drug penetration. Drugs can penetrate through the stratum corneocytes (A), between the stratum corneocytes (B), transfollicularly via any hair follicle (C), or via an eccrine sweat gland or sebaceous gland (D). The percutaneous absorption of drugs is important from three perspectives. Veterinary clinicians should be concerned about how much of a drug actually penetrates the skin to achieve a pharmacological effect in treating diseases of the skin. This can be a function of several factors including vehicle selection, hydration state of the stratum corneum, partition coefficient of the drug from vehicle into the stratum corneum lipids, the integrity of the stratum corneum, and cutaneous blood flow. The same endpoints, but in an opposite direction, also impact the efficacy and duration of effect for topical transdermal products. The final need for knowing the extent of percutaneous absorption lies in the field of public health and food consumption. Many compounds used in food animals are applied to and absorbed through the skin and enter the systemic circulation. Many of these compounds are stored in edible tissues (fat, muscle) for long periods of time, causing concern about residues in these tissues (Baynes et al., 1997; Riviere, 1992). The stratum corneum is accepted to be the major barrier, but not the only barrier, to percutaneous penetration in most species of animals. Passive diffusion is defined here as the movement of matter from one space to another space by random molecular motion. No known active transport processes are used by stratum corneum to modulate percutaneous absorption. To better understand how substances diffuse through the stratum corneum, it is convenient to think of the stratum corneum as a wall in a “brick and mortar” configuration (Elias, 1983), with the bricks representing the stratum corneocytes and the mortar representing the lipid matrix around them, as shown in Figure 47.3. In this context, permeation through the stratum corneum might occur via two routes. The first involves the drug traversing through the stratum corneocytes (“bricks”) and extracellular lipid matrix (“mortar”), through the cells of the deeper epidermal cells, and then into the systemic circulation. The second route involves the chemical maneuvering its way through the stratum corneum via the intercellular lipid matrix only. It is generally accepted that the primary route of penetration is via the intercellular pathway. Because of the structure of the stratum corneum and the metabolic capabilities of the lower epidermal cells, the veterinarian must be aware that only a small percentage of the drug that is topically applied is going to actually penetrate the stratum corneum. This small fraction of the total dose will need to be able to affect the disease process occurring in the underlying layers of the epidermis. Figure 47.3 Simplistic “brick and mortar” model of the skin. Drugs can penetrate by traveling around the stratum corneocytes through the lipid matrix, or drugs can pass through each corneocyte via the lipid matrix. The molecular structure of the intercellular lipid matrix throughout the epidermis is in a liquid crystalline configuration, consisting of fatty acids, ceramides, triglycerides, sterols, sterol esters, cholesterol sulfate, and miscellaneous alkanes. As epidermal cells migrate superficially and transform into the cells of the stratum corneum, the lipid content surrounding these cells changes from polar to more neutral in nature. Specifically, phospholipids and triglycerides tend to decrease while fatty acids, cholesterol, cholesterol sulfate, ceramides, and sphingolipids increase as the epidermal cells differentiate (Elias, 1992). Enzymes are also present within this matrix of lipid. These lipids organize themselves into a lipid bilayer structure, with the hydrophobic ends of the molecules orienting themselves with other hydrophobic ends, and the hydrophilic ends orienting themselves in a similar fashion. More than one lipid bilayer can be formed within the intercellular matrix, leading to the formation of hydrophilic and hydrophobic channels, as illustrated in Figure 47.4. One might assume that this complex lipid barrier would result in very low absorption profiles for hydrophilic (water soluble) compounds since these molecules would have difficulty crossing a hydrophobic barrier, but some studies have shown that many hydrophilic compounds penetrate the epidermis in much higher quantities than predicted, implying that there are other mechanisms whereby hydrophilic compounds permeate the stratum corneum. The primary mechanism by which this is accomplished is for hydrophilic molecules to penetrate through fluctuations, or “kinks,” in the lipid alkyl chains (Potts and Francoeur, 1992; Potts et al., 1992). Further, the permeation of hydrophilic molecules (such as water, methanol, ethanol, etc.) through the lipid matrix is due to their small size and molecular weight, which aid them in traveling through the lipid matrix via passages established by the lipid alkyl chains. This view is supported by the observation that larger hydrophilic molecules have lower percutaneous absorptions than their smaller molecular weight counterparts. Alternatively, penetration may also occur through the aqueous channels formed between the polar head groups of the lipid layers. Figure 47.4 The lipid bilayer model of the stratum corneum. More recently, the role of tight junction in the barrier function of the skin and permeability to substances has been discovered. Tight junctions are present in the SG and are composed of a number of proteins that selectively and dynamically regulate the penetration of substances through epithelia, including the epidermis. Tight junctions are actively remodeled to allow the migration of keratinocytes toward the surface without compromising the barrier function of the skin (Matsui and Amagai, 2015). Chemicals may also permeate the skin using the skin appendageal route, mostly by way of hair follicles and sweat ducts. The importance of the transappendageal route on percutaneous absorption is controversial, but some studies indicate that the importance of this route tends to be species specific (Pitman and Rostas, 1981). Generally, those animals possessing a sparse number of hair follicles per area of skin (humans, pigs) are considered to have little, if any, absorption of topically applied compounds via this route when compared to those animals having a high density of hair follicles (cattle, sheep). In these latter animals, the stratum corneum barrier is just as impermeable to drugs as that of their less-haired counterparts, but the drug permeates the skin barrier via the hair follicles, sweat ducts, or other openings in the stratum corneum, increasing the percutaneous absorption of the chemical. It has been demonstrated that transappendageal absorption is high initially, then becomes insignificant, due to the small surface area of follicles and glands in relation to the total surface area of the stratum corneum. Topical delivery of pesticides to domestic livestock has been used for many years for the control of external as well as internal parasites of these animals, indicating that enough of the pesticide is absorbed systemically to kill some internal parasites while not harming the host animal (Riviere, 1992). Two events must occur for a drug to be successfully delivered to the epidermis under the stratum corneum: (i) the drug must diffuse out of the vehicle and onto the surface of the stratum corneum; and (ii) the drug must be able to penetrate the stratum corneum (Idson, 1983). Restated, the vehicle in which the topical drug is being delivered should have significantly less affinity for that drug than for the stratum corneum. In actuality, many factors enter into the release of drug from the vehicle and its penetration through the stratum corneum. These factors have been mathematically described for steady-state kinetics in Fick’s first law of diffusion (Idson, 1983): where dQ/dt is a steady rate of penetration, PC is the drug’s partition coefficient between the vehicle and the stratum corneum, Cv is the concentration of the drug dissolved in the vehicle, D is the diffusion coefficient, A is the area of skin to which the drug is applied, and h is the diffusion path length through the stratum corneum. In short, Fick’s law states that the driving force causing the transfer of a drug from regions of high concentration to areas of low concentration is proportional to the concentration gradient (Idsen, 1975). The diffusion coefficient is primarily correlated to the partition coefficient of the penetrant, and can also be predicted from a knowledge of the drug’s molecular volume and hydrogen bond activity (Potts and Guy, 1995). Only the nonionized moiety of a weak acid or base is available for diffusion across the stratum corneum. The pH of skin is variable and sensitive to hydration state. The typical pH of skin ranges from 4.2 to 7.3. For drugs with pKa values within this range, the ionized fraction may vary according to the principles governed by the Hendersen–Hasselbalch equation, which would alter the amount of drug available for absorption. This is usually controlled for in the dermatological formulation by use of buffering systems. Theoretically, vehicles with different pH may have species-specific absorption for drugs with pKa values in this range. A vehicle is defined as the medium in which a medicinally active agent is topically administered. It is a gross misconception that the vehicle in which topical drugs are dissolved is inert and has little effect on the skin; in fact, the physical and chemical properties of the vehicle will largely determine how successfully the drug will penetrate the skin. This has been clearly demonstrated with the pesticide carbaryl in pigs (Baynes and Riviere, 1998). The vehicle may penetrate the stratum corneum to some extent and change the solubility of the intercellular lipid matrix. A simplistic view of the fate of topically applied drugs has been outlined in Figure 47.5. Figure 47.5 Schematic representation of the fate of topically applied drugs. The partition coefficient is a unitless measure of the relative affinity of a compound between a highly hydrophobic phase (usually octanol) and a hydrophilic phase (water), and is a physicochemical factor that has a major role in determining the percutaneous absorption of a chemical. As the partition coefficient increases, the compound has a greater affinity for the lipid phase and less affinity for the water phase. As discussed earlier, the intercellular matrix of the skin consists of several different types of lipid, the ratios of which may vary from site to site, and furthermore be modulated by vehicle penetrating the skin. Skin penetration of many chemicals has often been correlated to the partition coefficient of the penetrant. Generally, as the log of the partition coefficient increases, lipid solubility increases and there is an overall increase in the percutaneous absorption of the compound through the skin. This scenario is not always the case, however; some poorly lipid soluble compounds (i.e., low partition coefficients) sometimes penetrate the skin much more easily than compounds with higher partition coefficients. Once penetration into the skin occurs, the molecule must eventually leave the lipid phase to be able to reach the systemic circulation. Compounds with high partition coefficients tend to remain within the lipid of the skin and form a reservoir rather than pass through; this is a well-documented phenomenon in skin (Muhammad et al., 2005). It has been shown that a log partition coefficient of 1–4 is desirable for skin penetration of most compounds. Absorption of the vehicle into the stratum corneum is another factor to consider, since the vehicle may cause some disruptions in the composition and orientation of the intercellular lipid matrix. Partition coefficients for use in predicting the percutaneous absorption of topically applied drugs should ideally measure the index of affinity of a drug between the vehicle the drug is being delivered in and the lipid/vehicle composition of the stratum corneum. Unfortunately, few measurements of this kind are in existence because interaction of the vehicle with the stratum corneum lipids invalidates its determination. The role of partition coefficients in skin has been extensively reviewed elsewhere (Surber et al., 1990a, 1990b; Sloan et al., 1986; Aungst et al., 1990). The optimal vehicle is one in which the drug is soluble enough to enter into solution, but has less affinity for the vehicle than the stratum corneum lipids, thereby favoring partitioning from the vehicle into the epidermis. Optimal delivery for a polar drug may thus be a relatively hydrophobic vehicle that has sufficient solubility to dissolve the drug. However, if a drug is too soluble in a vehicle relative to the stratum corneum, the drug may persist in the vehicle and only slowly release drug into the skin for as long as the vehicle stays on the skin. The effect of the vehicle on chemical absorption has been quantitated based on competing physical chemical interactions of the drug and the vehicle versus the skin, using quantitative structure activity relationship principles (Riviere and Brooks, 2005, 2007; Karadzovska et al., 2013a, 2013b; Riviere et al., 2014). These studies also compared topical ectoparasiticides vehicle modulation between canine and porcine skin and has the potential for rationally designing topical drug formulations based on physical chemical principles. The ability of the vehicle to stay on the skin surface long enough to allow passive absorption of the drug to occur is an important factor in passive drug absorption. The vehicle can be removed by three mechanisms: (i) absorption of the vehicle through the stratum corneum, (ii) evaporation from the skin surface into the surrounding air, or (iii) physical removal (rubbing, licking, scratching, etc.). If the vehicle penetrates the stratum corneum more quickly than the drug, the concentration of the drug in the vehicle left on the surface of the stratum corneum will increase, which would increase the concentration of drug driving absorption, but would also have the potential of inducing precipitation of the drug onto the skin’s surface. The vehicle may also change the permeability of the lipid matrix (e.g., function as an enhancer). Evaporation of the vehicle will result in a similar scenario.
Dermatopharmacology: Drugs Acting Locally on the Skin
Anatomy and Histology
The Epidermis
The Dermis
Buttocks
Pinnae
Humeroscapular Joint
Thoracolumbar Joint
Ventral Species Abdomen
Feline
1.82 ± 0.59
6.46 ± 2.30
1.86 ± 0.70
2.39 ± 0.35
6.19 ± 0.94
Bovine
6.03 ± 1.84
6.98 ± 2.19
5.51 ± 2.32
5.49 ± 1.49
10.5 ± 2.13
Canine
2.21 ± 0.67
5.21 ± 1.53
5.52 ± 1.31
1.94 ± 0.27
8.78 ± 1.40
Equine
3.16 ± 1.22
NA
6.76 ± 1.49
2.99 ± 0.86
8.90 ± 1.46
Primate
3.12 ± 0.58
20.9 ± 5.37
8.49 ± 3.28
2.40 ± 0.82
3.58 ± 0.41
Mouse
3.88 ± 0.92
1.41 ± 0.48
10.1 ± 3.51
20.6 ± 4.69
36.9 ± 8.14
Porcine
3.08 ± 0.48
11.7 ± 3.02
6.75 ± 2.09
2.97 ± 0.56
10.7 ± 2.14
Rabbit
3.55 ± 0.93
8.38 ± 1.53
5.38 ± 1.06
5.46 ± 0.94
17.3 ± 6.31
Rat
4.20 ± 1.05
9.13 ± 4.97
6.22 ± 1.47
9.56 ± 2.17
11.4 ± 5.53
Species Differences
Biochemistry
Energy Production and Utilization
Drug Biotransformation
Lipid Metabolism
Protein Metabolism
Principles of Percutaneous Absorption: Skin Permeability
Topical Vehicles
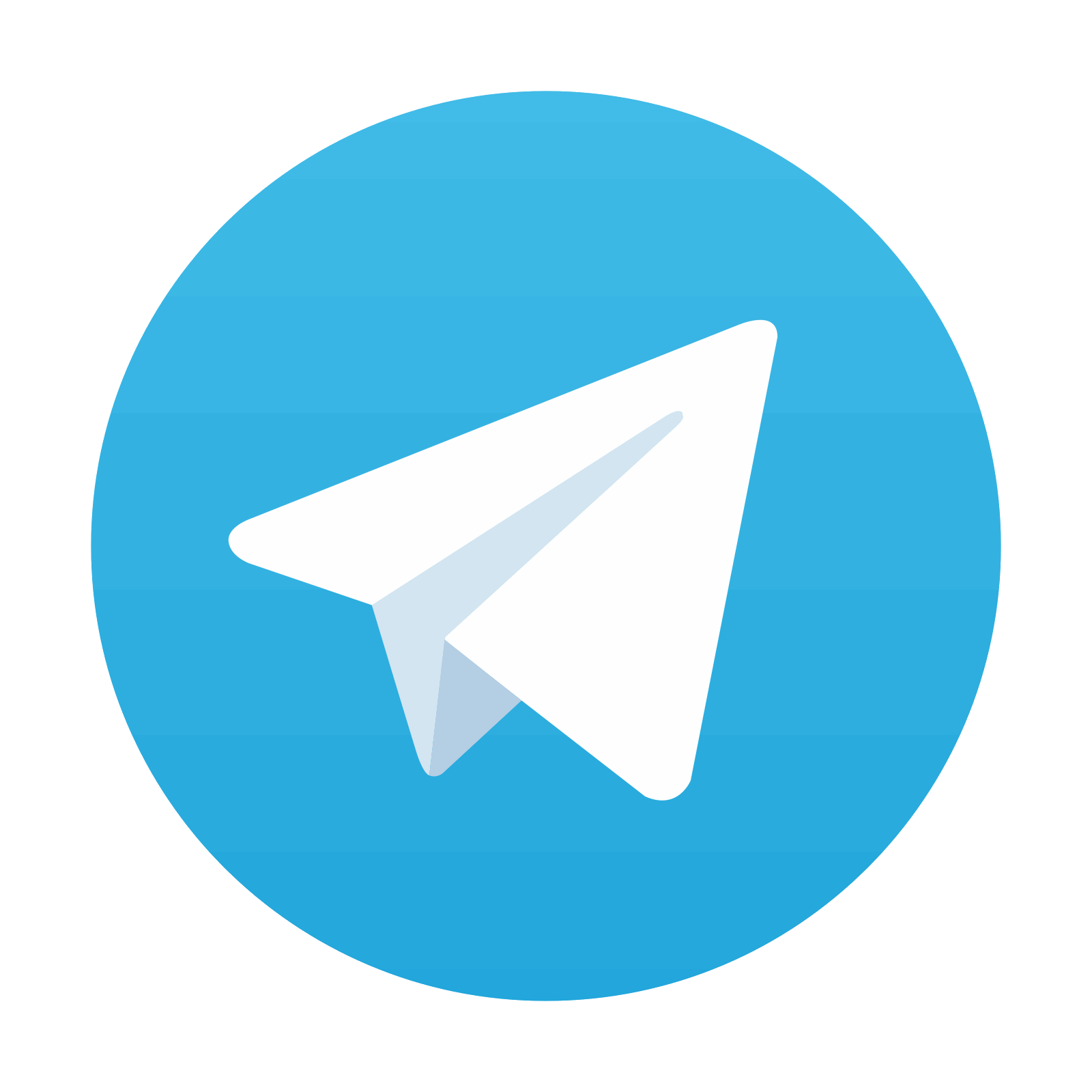
Stay updated, free articles. Join our Telegram channel

Full access? Get Clinical Tree
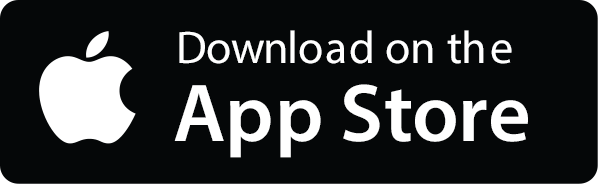
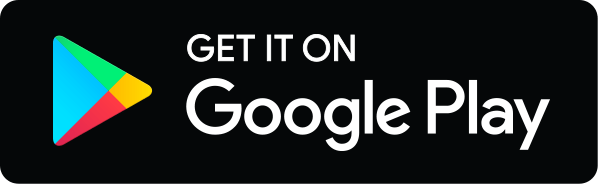