Chapter 12 Considerations in Pregnant or Lactating Patients
Pregnant animals are a unique population with respect to their response to xenobiotic exposures, either therapeutic or accidental. The dynamic physiological changes that occur within the maternal-placental-fetal unit during pregnancy influence the pharmacokinetic processes of xenobiotic absorption, distribution, metabolism, and elimination. In lactating patients, the xenobiotic concentration in milk is directly proportional to the corresponding concentration in maternal plasma. For most xenobiotics, the amount ingested by neonates rarely attains toxic concentrations. However, xenobiotic toxicity can develop in the pregnant or lactating animal, fetus or neonate when sufficient compound is present to exert a damaging effect on cells. There is a scarcity of data on specific pharmacokinetic measurements during pregnancy and lactation in dogs and even less in cats. Most specific information on pharmacokinetics presented in this review is based on comparative data from humans and laboratory animals.
PREGNANT PATIENTS
In dogs, apparent serum concentrations of progesterone and estradiol are similar in pregnant and nonpregnant cycles except for the abrupt decrease in both at parturition. However, if corrections are made for hemodilution that occurs during pregnancy, both steroid hormone concentrations are significantly higher in the last half of gestation.1 This is supported by increased fecal estradiol and progesterone concentrations during the second half of pregnancy.2 Increased hepatic clearance and increased metabolism by the uterus and mammary gland also contribute to the absence of obvious increase in estradiol and progesterone concentrations during pregnancy.3 In addition, thyroxine and adrenocortical hormones are increased during the latter half of gestation. The combined effect of increased hormone secretion during pregnancy results in alterations in dermal, pulmonary, cardiovascular, renal, gastrointestinal, and hepatic function (Table 12-1). Although these changes are necessary for a successful pregnancy, unique absorption, distribution, metabolism, and clearance of xenobiotics must be considered when using drugs to treat or prevent disease or in response to accidental toxin exposures.
Table 12-1 Physiologic Changes During Pregnancy That Alter Pharmacokinetics
Physiological Parameter | Change |
---|---|
Absorption | |
Gastric emptying time | Increased |
Intestinal motility | Decreased |
Pulmonary function | Increased |
Cardiac output | Increased |
Blood flow to the skin | Increased |
Distribution | |
Plasma volume | Increased |
Total body water | Increased |
Plasma proteins | Decreased |
Body fat | Increased |
Metabolism | |
Hepatic metabolism | Increased or Decreased |
Extrahepatic metabolism | Increased or Decreased |
Excretion | |
Renal blood flow | Increased |
Glomerular filtration rate | Increased |
Pulmonary function | Increased |
ABSORPTION
Gastrointestinal
The site of absorption for most xenobiotics is the small intestine due to its large surface area. Xenobiotic absorption across the small intestine is similar between dogs and man, and is often faster than the rate of gastric emptying, such that gastric emptying is a rate-limiting role in xenobiotic absorption.4 In dogs, gastric emptying after a meal is 90 minutes.5 For many therapeutically useful drugs, the biological half-time is long enough to ensure that stomach emptying is not a critical parameter. However, a slower intestinal transit time can significantly increase xenobiotic absorption. Increased progesterone concentrations during pregnancy result in delayed gastric emptying and reduced small intestinal motility, with the net effect of orally administered compounds spending a longer time in both the stomach and small intestine.6 As a result of prolonged intestinal transit time, there is an increase in absorption of poorly water-soluble xenobiotics and an increase in metabolism of xenobiotics by the intestinal wall. Gastric pH is also increased during pregnancy as a result of reduced gastric acid secretion and increased gastric mucus secretion. The increase in gastric pH increases the ionization of weak acids within the stomach, which reduces their absorption.
Pulmonary
Respiratory rate is unchanged during pregnancy but tidal volume (the amount of air per breath) and pulmonary blood flow are increased, which alters the kinetics of inhaled xenobiotics in favor of alveolar uptake and elimination by exhalation.7,8 Highly lipid-soluble anesthetic agents would be absorbed more rapidly and cleared more rapidly during pregnancy. While the rate of anesthetic induction with volatile agents is not faster, the dose requirements for volatile anesthetic drugs (e.g., halothane, isoflurane, methoxyflurane) are reduced.9 The absorption of drugs that are administered as aerosols (bronchodilator compounds) is also increased.
Skin
In humans, substantial changes in blood flow to the skin occur during pregnancy, such that circulation to the hand increases by sixfold.10 Alterations in dermal blood flow may have a significant impact on the pharmacokinetics of transdermal xenobiotic exposure. Topical administration of insecticides or compounded pharmaceuticals may result in toxicity during pregnancy. Xenobiotic absorption from intramuscular delivery is also enhanced during pregnancy because of increased tissue perfusion secondary to vasodilation.
DISTRIBUTION
During pregnancy, increases in body weight, total body fat, total body water, extracellular water, intravascular volume, and cardiac output can influence xenobiotic distribution (Fig. 12-1). The increase in cardiac output during pregnancy in cats and dogs has not been published. However, cardiac output increases by greater than 20% in sheep, guinea pigs, goats, and rabbits and about 40% in humans during pregnancy. Increases in body fat allow for a larger volume of distribution for lipophilic xenobiotics. It has been suggested that the pregnancy-associated increase in progesterone concentration leads to increased aldosterone secretion and results in increased renal fluid retention. Increased total body water results in an increased hydrophilic xenobiotic distribution. For example, pharmacokinetic parameters calculated from the results of the intravenous administration of lidocaine in pregnant ewes showed that the volume of distribution was increased, resulting in an increase in half-life.11 Beginning in midgestation and lasting 1 to 2 months after parturition, hemodilution occurs as reflected in a decrease in hematocrit and plasma albumin concentration.1 In humans, the size of the fetus and number of fetuses influence the increase in plasma volume, but this relationship has not been studied in domestic animals.12 As a result of this pregnancy-associated dilutional hypoalbuminemia, total plasma concentration of a protein-bound xenobiotic decreases. In addition, steroid and placental hormones and serum lipids (from increased body fat) will displace xenobiotics from protein-binding sites, resulting in a rise in free (active) xenobiotic concentration of agents that would normally be protein-bound and potentially result in an increased physiological effect. This is most noticeable for acidic xenobiotics that are highly protein-bound.
Almost all xenobiotics cross the placenta and reach pharmacological concentrations in the fetus after exposure of the mother. Drugs administered to the mother may cross the placenta by passive diffusion, facilitated transport, and active transport. Lipophilic, nonionized molecules less than 600 Da can cross the placenta by passive diffusion.13 In women undergoing elective C-sections, rapid placental transfer of ketamine,14 propofol,15 diazepam,16,17 and atropine18 occur such that fetal cord vein concentrations are several times higher than maternal. Maternal and fetal blood pH and plasma protein binding also influence the rate of passive diffusion across the placenta. The fetal plasma pH is slightly more acidic than the maternal. Consequently, xenobiotics that are weak bases are nonionized and able to easily penetrate the placental barrier. However, after crossing the placenta and making contact with the relatively acidic fetal blood, these molecules will become more ionized, leading to “ion trapping.” Protein-bound xenobiotics do not cross the placenta. In general, fetal plasma proteins bind xenobiotics with less affinity compared with those of the dam (e.g., ampicillin), with the exception of a few xenobiotics (e.g., salicylates) that have a greater affinity for fetal plasma proteins than maternal. Compounds that become bound to fetal proteins represent a depot of xenobiotic in the fetus that would prolong fetal exposure after cessation of maternal exposure. While hydrophilic compounds cannot cross the placenta by passive diffusion, they cross via aqueous diffusion through the water-filled pores between the cells (paracellular pathway).13
METABOLISM
Alterations in the hormonal milieu of pregnancy are associated with changes in xenobiotic metabolism. In most cases, xenobiotic metabolism occurs primarily in the liver. Decreased protein binding during pregnancy results in greater xenobiotic availability for hepatic biotransformation, a process that renders a xenobiotic more water-soluble and thus readily excreted by the kidneys. Hepatic metabolism during pregnancy has been well investigated in rats and to a much lesser extent in other species. Changes in xenobiotic metabolism may have an impact on first-pass effect of drugs given orally during pregnancy. Some microsomal enzymes of the hepatic cytochrome P450 system are induced by progesterone, resulting in a higher rate of xenobiotic metabolism.19 For example, it has been found clinically that the phenytoin dosage needs to be increased during pregnancy to maintain plasma concentrations that are adequate to control epileptic seizures in women.20 However, the capacity for hepatic biotransformation is six times greater in dogs compared with man.21 Some microsomal enzymes are competitively inhibited by progesterone and estradiol, resulting in impaired xenobiotic metabolism.22 Because of hormonal inhibition of hepatic microsomal oxidases, theophylline degradation is delayed during pregnancy.23 Elevated progesterone during pregnancy also inhibits hepatic glucoronidation and extrahepatic cholinesterase activity.24
Most biotransformation reactions present in the liver have also been described within the placenta, although placental biotransformation capacity is many times less than that of the liver.25 The placenta contains several enzymes that are capable of metabolizing xenobiotics via oxidation, reduction, hydrolysis, and conjugation pathways. For example, the placental cytochrome P450 enzyme 1A1 is induced following exposure to aromatic hydrocarbons found in tobacco smoke.26 Alternatively, toxic intermediate formation may result from the placental oxidative biotransformation system. Xenobiotics that are not metabolized by the placenta enter the fetal hepatic circulation via the umbilical vein. However, approximately 50% of umbilical venous blood flow will bypass the liver via the ductus venosus, contributing to a possible accumulation of xenobiotics within the fetus. The near-term dog fetus shows evidence of a functioning enterohepatic circulation of bile salt, and xenobiotics can be found in meconium. Because of the small bile salt pool, apparent limited capacity of the gallbladder to concentrate bile, and evidence of a functional hepatic bypass, fetal hepatic metabolism is immature compared with that of the adult.27
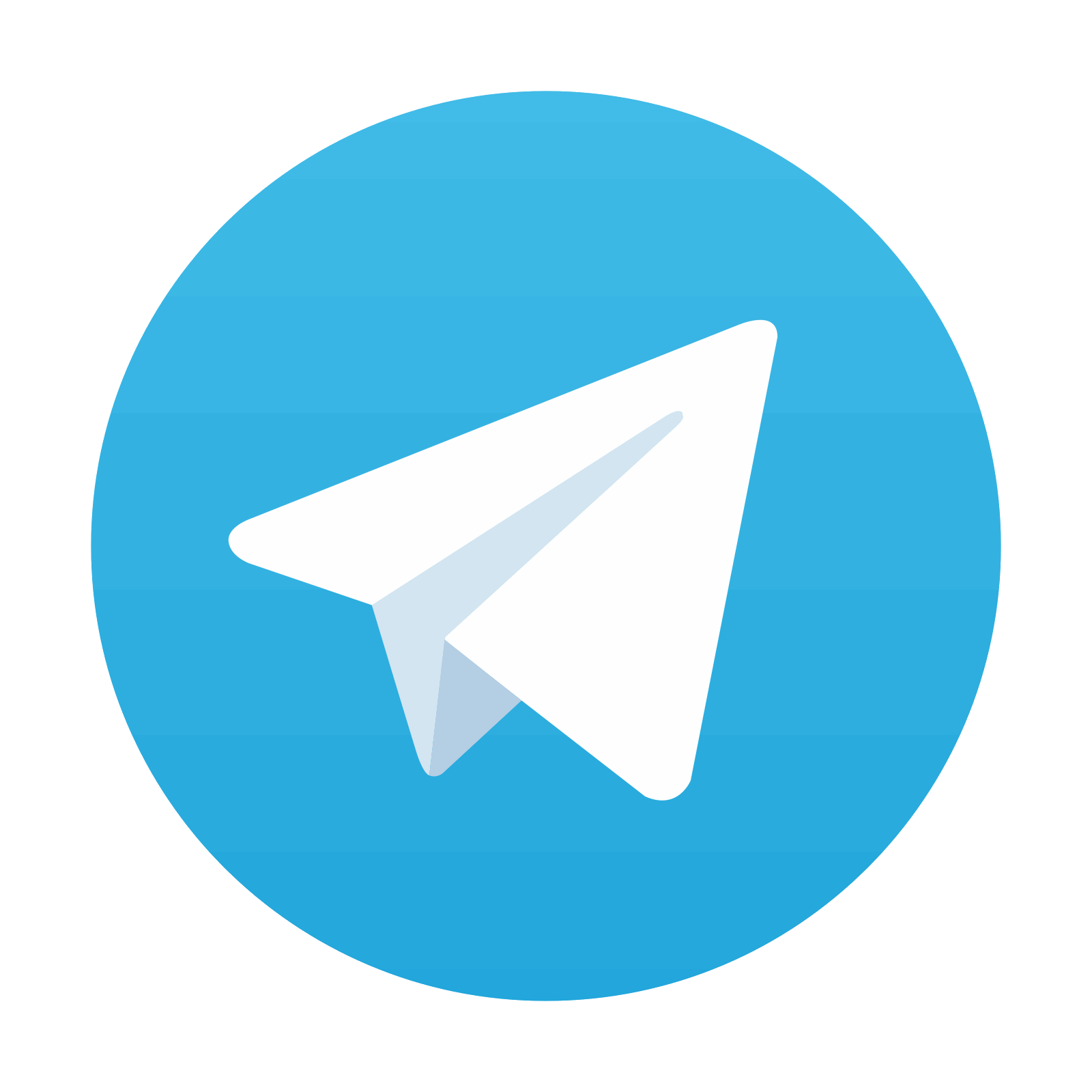
Stay updated, free articles. Join our Telegram channel

Full access? Get Clinical Tree
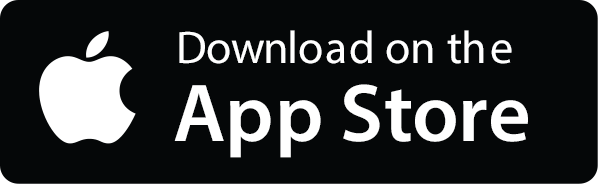
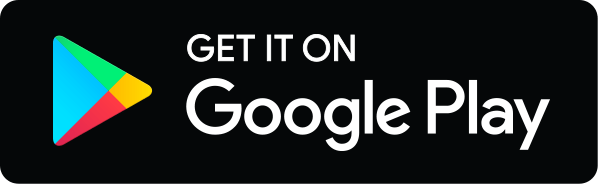