Chapter 2 The goals of resuscitation and maintenance fluid therapy in the critically ill animal are to restore and maintain perfusion and hydration without causing volume overload and complications caused by pulmonary, peripheral, or brain edema. By using colloid fluids in conjunction with crystalloid fluids (see Chapter 1), goal-driven resuscitation (also known as end-point resuscitation) can be achieved more rapidly and with less fluid volume compared with crystalloid fluids alone. Maintaining an effective circulating volume can be challenging when there is vascular leakage, vasodilation, excessive vasoconstriction, inadequate cardiac function, hypoalbuminemia, or ongoing fluid loss. Whether a fluid administered intravenously remains in the intravascular compartment or moves into the interstitial or intracellular spaces depends on the dynamic forces that affect fluid movement between body fluid compartments. where V = filtered volume, k = filtration coefficient, HP = hydrostatic pressure, c = capillary, i = interstitial fluid, gc = subendothelial glycocalyx, σ = membrane pore size, COP = colloid osmotic pressure, and Q = lymph flow. The main components that control intravascular fluid volume include intravascular colloid osmotic pressure (COP) and hydrostatic pressure (HP) (Figure 2-1). Eighty percent of the COP is produced by albumin, which is the most abundant extracellular protein. The pressure generated by albumin is augmented by its negative charge, which attracts cations (e.g., sodium) and water around its core structure. This unique dynamic is termed the Gibbs–Donnan effect. Vascular permeability to ion species, ionic concentration gradients, and electrochemical charges influence the movement of ions such as sodium, potassium, and chloride. Capillary membrane pore size and the filtration coefficient control the ease with which larger molecules such as albumin leave the intravascular space. Pore size varies from tissue to tissue (e.g., continuous capillaries in the brain and fenestrated capillaries in the liver). The filtration coefficient is variable and partly dependent on the amount of albumin in the intravascular space and within the interendothelial cleft. Figure 2-1 The main components of the Starling-Landis equation that affect intravascular water content include intravascular hydrostatic pressure (HP) and colloid osmotic pressure (COP). The intravascular HP is a result of intravascular volume, cardiac output (CO), and systemic vascular resistance (SVR). Under normal conditions the HP favors the movement of fluid from the vessel into the interstitium. The COP is the force that opposes intravascular HP, supporting intravascular water retention. The COP is generated by the presence of large molecules (primarily proteins) that do not readily pass the capillary membrane and create an osmotic effect. The dynamics of normal fluid movement across the capillary membrane can change with certain diseases. Fluid moves from the intravascular to the interstitial or third-space compartment under certain conditions (Figure 2-2 and Table 2-1). Plasma COP can increase with water loss (hemoconcentration), remain the same when there is acute hemorrhage, or decrease with protein loss. In addition to capillary dynamics, the composition of intravenously administered fluids determines how these fluids are distributed across fluid compartments. Figure 2-2 Fluid can pass out of the intravascular space under several conditions listed in Table 2-1: increased intravascular hydrostatic pressure (HP), increased capillary permeability, decreased filtration coefficient, and decreased intravascular colloid osmotic pressure (COP). These conditions lead to the consequences of peripheral edema and hypovolemia. The two major categories of intravenous fluids are crystalloids (see Chapter 1) and colloids. A crystalloid fluid is a water-based solution with small molecules permeable to the capillary membrane. A colloid fluid is a crystalloid-based solution that contains large molecules that do not easily cross normal capillary membranes. When large volumes are needed for intravascular fluid resuscitation, crystalloids alone may fail to provide effective intravascular volume support without causing interstitial volume overload and edema. Ultimately the selection of a colloid or a combination of a colloid with a crystalloid for intravenous resuscitation and maintenance is based on the pharmacology of the fluid and the disorder that requires treatment. Each colloid solution is unique, and knowledge of the composition and pharmacology of the fluid is needed to make an appropriate colloid selection for an individual patient’s needs (Table 2-2). Differences in macromolecular structure and weight dictate the colloid osmotic effect, method of excretion, and half-life of the colloid solution. The larger the number of small molecules per unit volume of the colloid, the greater will be the initial colloid osmotic effect and plasma volume expansion. When the number of large molecules per unit volume of colloid is high, the colloid is retained longer within the vascular space. A 5-g lyophilized canine albumin (www.ABRInt.net) recently has been developed for use as a replacement colloid in the treatment of hypoalbuminemia in dogs. It is stored in a dehydrated powder form and reconstituted with isotonic saline to a desired concentration. Information on its clinical use is limited to albumin replacement in dogs with hypoalbuminemia and septic peritonitis, but not for volume replacement.
Colloid Fluid Therapy
Fluid Dynamics
Basic Colloid Fluid Pharmacology
Albumin
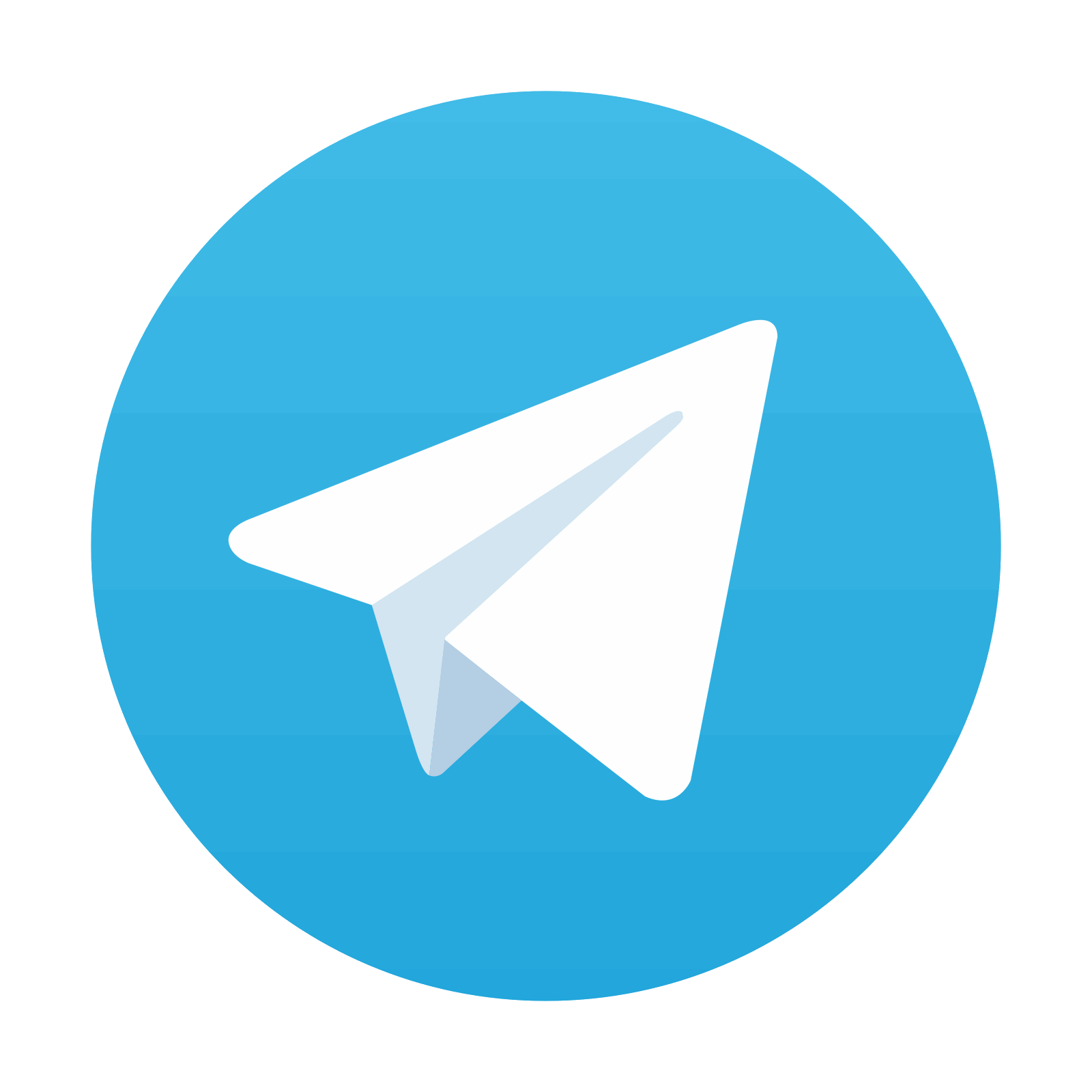