Chapter 8
Clinical Pathology
Clinical Pathology of Reptiles
When evaluating the hematologic and biochemical responses of reptiles, one should consider the various normal physiologic and external factors that may enhance or inhibit these ectotherms’ response to disease. These factors include age, gender, environment, season, and nutritional status.1–4 Differences between captive individuals and free-ranging reptiles of the same species may be too great for use in comparison studies. It has been shown that captive-bred snakes tend to have significantly higher erythrocyte values, lower azurophil, heterophil, and punctate reticulocyte percentages, and higher lymphocyte numbers compared with wild-caught snakes.5 Total leukocyte counts of captive male crocodiles were higher than those of females, whereas no differences between the sexes were observed in wild crocodiles of the same species.6 The total leukocyte counts of foraging sea turtles were shown to be statistically lower than those for the nesting turtles that were likely fasting.7 A marked seasonal variation was noted in all hematologic and blood biochemistry variables in turtles, except mean cell hemoglobin concentration, the relative monocyte and heterophil counts, and creatinine.8 The influence of the normal physiologic and external factors was suspected to be responsible for the lack of significant differences in the packed cell volume, total protein concentration, and total leukocyte count between sick and healthy box turtles.3 That significant hematologic changes in young crocodiles subjected to low temperature treatments occurred without any significant variation in corticosterone concentrations would suggest that the immunosuppressive effect of low temperature was independent of corticosterone concentrations and the hypothalamic–pituitary–adrenocortical axis.9 All of these studies support the importance of knowing the various physiologic and external factors that might influence test results.
The cellular responses in reptilian blood, in particular, are less predictable than those of endothermic mammals and birds whose cellular microenvironments are more stable. The external factors mentioned earlier have a greater influence on the physiology and health of ectothermic vertebrates than they do on endotherms. Because species, age, gender, nutritional status, season, and physiologic status influence blood tests, interpretation of the hemogram and plasma biochemistry profiles becomes challenging. In addition, discrepancies among the results of various analytic methods should also be taken into consideration when one is comparing the results between studies.7 Differences in test results may even occur between blood collection sites.10 All of these factors make the creation of meaningful species-specific reference ranges difficult when compared with those of domestic mammals. An ideal reference interval for each analyte for a given species of reptile would take into consideration gender, age (size), body condition, parasite load, nutritional status, season, quality of habitat (captive versus wild), presence of environmental stressors, blood collection site, and analytic method. All of these things should be taken into consideration when interpreting hematologic and diagnostic chemistry data from a reptilian patient. The American Society for Veterinary Clinical Pathology has provided guidelines for the creation of reference intervals that includes the use of data obtained from a small number of individuals. These guidelines are available from http://www.asvcp.org/pubs/qas/index.cfm.
Hematology
Evaluation of the hemogram and blood film is part of the laboratory evaluation of reptilian patients. Hematology is used to detect conditions such as anemia, inflammatory diseases, parasitemia, hematopoietic disorders, and hemostatic alterations. The hematologic evaluation of a reptilian patient may provide clues to its health status. Continued hematologic monitoring of the patient also provides important information regarding the patient’s response to treatment or the progression of the disease. For example, lymphocytosis, heterophilia, and azurophilia in tortoises have been shown to occur concurrently with a resurgence of clinical signs in patients with upper respiratory disease, providing information regarding the status of the patients.11
The blood of reptiles contains nucleated erythrocytes, nucleated thrombocytes, heterophils, eosinophils, basophils, lymphocytes, and monocytes. The normal hematologic values of reptiles determined by different laboratories can vary significantly. This variation is likely caused by differences in blood sampling, handling, and analytic techniques. Other factors that are likely to contribute to the variation in the normal hematologic values of reptiles include variations in environmental conditions, physiologic status, age, gender, nutrition, and use of anesthetics. Published hematologic reference values for reptiles often fail to include information that may influence the hemogram, especially the environment of the population of reptiles used as normal controls. For these reasons, the published normal reference values of reptiles vary greatly compared with those of domestic mammals.
A properly prepared blood film provides low numbers of smudge cells and an ample amount of monolayer areas for evaluating the cells. The coverslip-to-slide and the bevel-edge slide techniques can provide such quality.12 Whenever possible, examination of blood films obtained from fresh non–anticoagulated blood is preferred. Ethylenediaminetetraacetic acid (EDTA), considered to be the anticoagulant of choice for hematologic studies, may cause the blood to lyse in some species of chelonians; therefore lithium heparin is typically used as an anticoagulant when blood is collected from those reptiles.13,14 However, heparin often imparts a blue tinge to the overall staining of the blood film and causes clumping of leukocytes and thrombocytes, affecting cell counts.15 If heparin is used as an anticoagulant for reptilian hematologic studies, the sample should be processed immediately so that its effects are minimized on the cells. If whole blood samples anticoagulated with lithium heparin or EDTA cannot be evaluated immediately, then the samples should be stored at 4°C and evaluated no longer than 24 hours after collection for best results.16 Citrate used as an anticoagulant often results in significant cell lysis and should be avoided. The addition of albumin does not prevent cell lysis as others have suggested.17
Reptilian Erythrocytes
Mature erythrocytes of reptiles are permanently nucleated, blunt-ended ellipsoids that are larger than erythrocytes of birds and mammals. The erythrocyte size for most reptiles ranges from a length by width of 14 × 8 μm to 23 × 14 μm and the length-to-width ratio of most reptile erythrocytes is 1.7 to 1.8.17 Reptile erythrocytes that are round (length-to-width ratio, 1.5 or greater) rather than oval are rare and likely to occur in chelonians and some snakes.17 The mean cellular volume (MCV) of most reptilian mature erythrocytes ranges between 200 and 1200 fL. The reptilian erythrocyte has a centrally positioned oval to round (especially chelonians) nucleus that is oriented along the cell’s long axis. The nuclei often have irregular margins and contain dense purple chromatin. The cytoplasm appears orange-pink with Romanowsky stains such as Wright stain. Polychromatophilic erythrocytes have nuclear chromatin that is less dense and cytoplasm that is more basophilic than mature erythrocytes.
Reptiles have lower total erythrocyte counts (300,000 to 2,500,000 erythrocytes/μL) compared with mammals and birds.18,19 An inverse relationship appears to exist between the total red blood cell count (TRBC) and the size of the erythrocytes.18 Chelonians have the largest of the reptilian erythrocytes (MCV greater than 500 fL) and, as a result, the lower TRBC values (500,000 erythrocytes/μL or less). Lizards tend to have smaller erythrocytes (MCV less than 300 fL) than other reptiles; therefore they have higher total erythrocyte counts (1,000,000 to 1,500,000 erythrocytes/μL).18,19 Snakes have lower TRBC values (700,000 to 1,600,000 erythrocytes/μL) than lizards but greater numbers than chelonians. The TRBC, hemoglobin concentration (Hb), and PCV values vary with a number of factors, such as environment (TRBC values are highest before hibernation and lowest immediately after hibernation), nutritional status; and gender (males tend to have higher TRBC values than females).17–23
The mean hemoglobin concentration (MCHC) is the red blood cell index denoting the proportion of an average erythrocyte that is composed of hemoglobin in grams per 100 red blood cells (gHb/100 RBC). The average MCHC for reptiles is 30% (range, 22% to 41%).17,24,25 The hemoglobin concentration of reptilian blood generally ranges between 6 and 10 g/dL.26 Most reptiles have multiple hemoglobin types, and considerable variation is seen in oxygen affinity between individual red blood cells.27
Immature erythrocytes are occasionally seen in the peripheral blood of reptiles, especially in young animals or those undergoing ecdysis. Immature erythrocytes are round to irregular cells with large round nuclei and basophilic cytoplasm (Figure 8-1). The nucleus lacks the dense chromatin clumping of the mature cell and has a characteristic checkerboard-like pattern. Immature erythrocytes frequently appear smaller than mature erythrocyte, probably because the final stage of erythrocyte maturation involves changing from a spherical cell, which appears small, to a flattened ellipsoid, which appears larger. In addition, the larger mature cell may contain more hemoglobin. Mitotic activity associated with erythrocytes is common in the peripheral blood of healthy reptiles.
FIGURE 8-1 Immature (mid-polychromatic rubricytes) erythrocytes (arrows) in the blood film of Reeves’ Turtle (Mauremys reevesii); Wright-Giemsa stain × 1000.
Reticulocytes are detected by staining cells with a supravital stain, such as new methylene blue. They are a normal constituent of reptilian blood, representing 1.5% to 2.5% of the red blood cell population.28,29 Reptilian reticulocytes that have a distinct ring of aggregated reticulum that encircles the red cell nucleus are likely the cells recently released from the erythropoietic tissues. Polychromatic erythrocytes account for less that 1% of the red blood cell population of most clinically healthy reptiles (Figure 8-2).
FIGURE 8-2 A polychromatic erythrocyte in the blood film of a Box Turtle (Terrapene carolina triunguis); Wright-Giemsa stain × 1000.
Round to irregular basophilic inclusions are frequently seen in the cytoplasm of erythrocytes in peripheral blood films from many species of reptiles (Figure 8-3). These inclusions most likely represent an artifact of slide preparation because blood films made repeatedly from the same blood sample often reveal varying degrees of these inclusions. Electron microscopy suggests these inclusions are degenerate organelles.30 Other artifacts found in erythrocyte cytoplasm include vacuoles and refractile clear areas. These can be minimized with careful blood film preparation.
Erythrocyte Responses in Disease
The normal PCV of most reptiles is approximately 30% (published ranges are 20% to 40%)20,31,32 Therefore a PCV less than 20% is suggestive of anemia, and values greater than 40% suggest either hemoconcentration or erythrocytosis (polycythemia). Indicators of anemia include a decreased erythrocyte count, PCV, and hemoglobin concentration. The causes of anemia in reptiles are the same as those described for birds and mammals. The anemia can be classified as hemorrhagic (blood loss), hemolytic (increased red cell destruction), or depression anemia (decreased red cell production). Hemorrhagic anemias are usually caused by traumatic injuries or blood-sucking parasites; however, other causes, such as a coagulopathy or an ulcerative lesion, should be considered. Hemolytic anemia can result from septicemia, parasitemia, or toxemia. Depression anemias are usually associated with chronic inflammatory diseases, especially those associated with infectious agents. A low PCV without evidence of red blood cell regeneration supports the presence of a depression anemia—one likely associated with a chronic condition such as poor nutrition, chronic infectious or parasitic disease, immune deficiency related to a debilitated state, or a combination of any of these causes.7 Other causes that should be considered for depression anemia in reptiles include chronic renal or hepatic disease, neoplasia, chemical contact, or possibly hypothyroidism.33,34
The degree of polychromasia or reticulocytosis in blood films of normal reptiles is generally low and represents less than 1% of the erythrocyte population. This may be associated with the long erythrocyte life span (600 to 800 days in some species) and therefore slow turnover rate of reptilian erythrocytes compared with those of birds and mammals.18,20 The relatively low metabolic rate of reptiles may also be a factor. Young reptiles tend to have a greater degree of polychromasia than adults.
Slight anisocytosis and poikilocytosis are considered normal for most reptile erythrocytes. Moderate to marked anisocytosis and poikilocytosis are associated with erythrocytic regenerative responses and, less commonly, erythrocyte disorders. An increase in polychromasia and the number of immature erythrocytes is seen in reptiles responding to anemic conditions. The erythrocyte regenerative response in reptiles is expected to be slower than that of birds and mammals, which respond within 1 week to stimulus. The slow reptilian response (e.g., red cell numbers may return to normal in 4 months after repeated phlebotomy) may be related in part to the long transit time from the rubriblast stage to the mature erythrocyte stage and the long life span of the reptilian red blood cell.35 Young reptiles or those undergoing ecdysis may also exhibit an increase in polychromasia and immature erythrocyte concentration. Erythrocytes exhibiting binucleation, abnormal nuclear shapes (anisokaryosis), or mitotic activity can be associated with marked regenerative responses (Figures 8-4 and 8-5). However, these nuclear findings may also occur in reptiles awakening from brumation or in association with severe inflammatory disease, malnutrition, and starvation.36 Basophilic stippling usually suggests a regenerative response but is also seen in patients with iron deficiency and, possibly, lead toxicosis. Hypochromatic erythrocytes are associated with iron deficiency or chronic inflammatory disease (presumably in association with iron sequestration). Erythroplastids (anucleated erythrocytes) are an incidental finding (less than 0.5% of the erythrocytes) in reptilian blood.37–39 A normocytic, hyperchromic anemia usually signals a regenerative anemia, whereas a normocytic, hypochromic anemia is often associated with infection or chronic inflammatory diseases.40
FIGURE 8-4 A binucleated erythrocyte likely representing accelerated erythropoiesis in the blood film of a Reeves’ Turtle (Mauremys reevesii); Wright-Giemsa stain × 1000.
Reptilian Leukocytes
Special stains (cytochemical staining techniques) are used to examine cellular constituents, such as enzymes, lipids, and carbohydrates. Peroxidase (PER; myeloperoxidase) is used as a marker for mammalian myeloid cells, such as neutrophils, eosinophils, and monocytes. Sudan Black B (SBB) stains lipids within neutrophils, eosinophils, and occasionally monocytes. Chloroacetate esterase (CAE) is a group of enzymes considered to be specific for mammalian neutrophils. Leukocyte alkaline phosphatase (LAP) is present in mammalian granulocytes and some subsets of lymphocytes. Periodic acid Schiff (PAS) staining is positive in a wide variety of mammalian blood cells and is used to differentiate granulocytic or megakaryocytic precursors from lymphoid precursors.41 Nonspecific esterases (NSE) and acid phosphatase (AP) staining of mammalian cells demonstrate unique staining characteristics. Toluidine blue (TB) is helpful in detection of mammalian basophils and mast cells. Some of these have been applied to reptilian blood cells in an effort to detect differences between normal cell types and similarities or differences between the hemic cells of different species (Table 8-1). The variation in cytochemical responses in these studies indicates that it is necessary to study species individually if meaningful clinical decisions are to be made.42 Differences in cytochemical responses among the blood cells of various reptiles and even in studies that have used the same species might also be a reflection of differences in technique. Variations in the reactivity of a cell to the same cytochemical stain can occur with differences in pH, incubation temperatures, staining time, and reagents.41,43 These factors add to the difficulty when one compares the results of different studies, most of which have failed to use human controls to compare positivity and negativity.44
TABLE 8-1
Summary of Selected Cytochemical Staining Reactions for Normal Leukocytes and Thrombocytes in Various Reptiles
Reptilian heterophils are generally round cells with eosinophilic (bright or dull orange), generally fusiform cytoplasmic granules (Figures 8-6 through 8-9). The cytoplasm of normal heterophils is colorless. The mature heterophil nucleus is typically round to oval and eccentrically positioned in the cell, with densely clumped nuclear chromatin.2,32,36,45–49 Some species of lizards have heterophils with lobed nuclei (Figure 8-10).17,23 Heterophils range between 10 and 23 μm in size but vary between species and the individual blood sample.17 Heterophil granules demonstrate heterogeneous electron microscopic morphologic features and cytochemical staining, indicating the presence of different granule types. The cytoplasmic granules of reptilian heterophils are usually PER negative, except for a few species of snakes and lizards.18,30,48,50,51 The heterophil granules from the Green Iguana (Iguana iguana) stain strongly positive with benzidine peroxidase similar to mammalian neutrophils.23 Reptilian heterophils do not stain for alkaline phosphatase.30 These findings indicate that reptilian heterophils are functionally equivalent to mammalian neutrophils but most likely behave like avian heterophils in that they rely more heavily on oxygen-independent mechanisms to destroy phagocytized microorganisms. The PER-positive heterophils of Green Iguanas suggest that these cells may possess bactericidal and oxidative properties similar to mammalian neutrophils.23 The lack of cytochemical consistency in reptilian heterophils makes it difficult to develop guidelines in a related taxonomic group.44
FIGURE 8-6 A heterophil in the blood film of a Wood Turtle (Glyptemys insculpta); Wright-Giemsa stain × 1000.
FIGURE 8-7 A heterophil in the blood film of a common Boa Constrictor (Boa constrictor); Wright-Giemsa stain × 1000.
FIGURE 8-8 A heterophil in the blood film of a Green Sea turtle (Chelonia mydas); Wright-Giemsa stain × 1000.
FIGURE 8-9 A heterophil in the blood film of a Box Turtle (Terrapene carolina triunguis); Wright-Giemsa stain × 1000.
FIGURE 8-10 A heterophil in the blood film of a Green Iguana (Iguana iguana); Wright-Giemsa stain × 1000.
Reptilian eosinophils are large round cells with spherical eosinophilic (typically brightly eosinophilic) cytoplasmic granules (Figures 8-11 and 8-12). The Romanowsky-stained granules of eosinophils found in the peripheral blood of some species of reptiles, such as the Green Iguana, stain blue (Figures 8-13 and 8-14). The cytoplasmic granules of eosinophils stain positive for PER in some species of reptiles, which allows easy differentiation between eosinophils and heterophils in those species with PER-negative heterophils.30,52–54 The blue-staining granules of the Green Iguana eosinophils do not stain with PER or other common cytochemical stains.23 As with heterophils, the size of eosinophils varies with species. For example, snakes tend to have larger eosinophils than do chelonians and lizards.17 The nucleus of the reptilian eosinophil is typically centrally located in the cell and has a variable shape, ranging from slightly elongated to lobed. The variation in the cytochemical staining of reptilian eosinophils may be associated with the variability in cytochemical techniques.44
FIGURE 8-11 An eosinophil in the blood film of a Wood Turtle (Glyptemys insculpta); Wright-Giemsa stain × 1000.
FIGURE 8-12 An eosinophil (arrow) and three thrombocytes in the blood film of a common Boa Constrictor (Boa constrictor); Wright-Giemsa stain × 1000.
FIGURE 8-13 An eosinophil and three thrombocytes in the blood film of a Green Iguana (Iguana iguana); Wright-Giemsa stain × 1000.
FIGURE 8-14 A cell resembling an iguana eosinophil in the blood film of a Reeves’ Turtle (Mauremys reevesii); Wright-Giemsa stain × 1000.
Reptilian basophils are usually small round cells containing basophilic metachromatic cytoplasmic granules, which often obscure the cell nucleus (Figures 8-15 through 8-18). When visible, the cell nucleus is slightly eccentric in position and not lobed. Basophils are easy to identify and stain strongly with TB stain.41,44 Basophil granules are frequently affected by water-based stains, which cause them to partially dissolve. Alcohol fixation and the use of Romanowsky stains are preferred because they provide the best staining for reptilian basophils. Basophils vary in size according to the species of reptile but generally range between 7 and 20 μm.17 Lizards tend to have small basophils, whereas turtles and crocodiles have large basophils.17
FIGURE 8-15 A ruptured basophil revealing its granules in the blood film of a Wood Turtle (Glyptemys insculpta); Wright-Giemsa stain × 1000.
FIGURE 8-16 A basophil in the blood film of a Green Iguana (Iguana iguana); Wright-Giemsa stain × 1000.
FIGURE 8-17 Two basophils in the blood film of a Painted Terrapin (Callagur borneoensis); Wright-Giemsa stain × 1000.
FIGURE 8-18 A basophil in the blood film of a Box Turtle (Terrapene carolina triunguis); Wright-Giemsa stain × 1000.
Reptilian lymphocytes resemble those of mammals and birds (Figures 8-19 through 8-21). They vary in size from small (5 to 10μm) to large (15μm).17,18 Lymphocytes are round cells that exhibit irregularity when they mold around adjacent cells in the blood film or fold at their cytoplasmic margin (Figure 8-22). They have a round or slightly indented nucleus that is centrally or slightly eccentrically positioned in the cell. The nuclear chromatin of mature lymphocytes is heavily clumped. Lymphocytes typically have a large nucleus to cytoplasmic ratio (N:C). The typical small mature lymphocyte has scant slightly basophilic (pale blue) cytoplasm. Large lymphocytes have more cytoplasmic volume compared with small lymphocytes, and the nucleus is often pale staining. The cytoplasm of a normal lymphocyte appears homogeneous and lacks vacuoles and granules. Lymphocytes generally stain negative for nearly all of the cytochemical stains.
FIGURE 8-19 A large lymphocyte (cell on the right) and a small lymphocyte (cell on the left) in the blood film of a Wood Turtle (Glyptemys insculpta); Wright-Giemsa stain × 1000.
FIGURE 8-20 A lymphocyte in the blood film of a Green Iguana (Iguana iguana); Wright-Giemsa stain × 1000.
FIGURE 8-21 A large lymphocyte and thrombocytes (smaller cells) in the blood film of a common Boa Constrictor (Boa constrictor); Wright-Giemsa stain × 1000.
FIGURE 8-22 A small lymphocyte with scalloped cytoplasmic margins in the blood film of a Box Turtle (Terrapene carolina triunguis); Wright-Giemsa stain × 1000.
Lymphocytes in some species of reptiles may be difficult to differentiate from thrombocytes because cell size, appearance, and stain response are similar in both cells (Figure 8-23). The differentiation of these cells is made easier in species whose thrombocytes are characteristically elongated. In general, thrombocytes are slightly smaller than and often have a denser more compact nuclear chromatin pattern than lymphocytes. In addition, thrombocytes often have cytoplasmic vacuoles that are not usually present in inactivated lymphocytes.
FIGURE 8-23 A lymphocyte and two thrombocytes in the blood film of a Green Sea turtle (Chelonia mydas); Wright-Giemsa stain × 1000.
Monocytes are generally the largest leukocyte in the peripheral blood of reptiles and resemble those found in the blood films of mammals and birds (Figures 8-24 through 8-28). They vary in shape from round to ameboid. The nucleus is variable in shape, ranging between round and oval to lobed. The nuclear chromatin of monocytes is less condensed and stains relatively pale compared with the nuclei of lymphocytes. The abundant cytoplasm of monocytes stains blue-gray, may appear slightly opaque, and may contain vacuoles or fine dust like eosinophilic or azurophilic granules. The monocytes of snakes often have a round to oval nucleus and contain abundant dust like azurophilic granules. Cytochemical staining of monocytes can vary among species, which may be related to variation in techniques. The monocytes of the Green Iguana stain positive with AP, alpha-naphthyl butyrate esterase, and PAS and negative with SBB and PER.23 Snake monocytes with distinct azurophilic cytoplasmic granules (azurophils) stain positive for PER, SBB, and PAS.23
FIGURE 8-24 A monocyte in the blood film of a Green Iguana (Iguana iguana); Wright-Giemsa stain × 1000.
FIGURE 8-25 A monocyte in the blood film of a common Boa Constrictor (Boa constrictor); Wright-Giemsa stain × 1000.
FIGURE 8-26 A monocyte in the blood film of a Painted Terrapin (Callagur borneoensis); Wright-Giemsa stain × 1000.
FIGURE 8-27 A monocyte (center cell) and small lymphocyte in the blood film of a Box Turtle (Terrapene carolina triunguis); Wright-Giemsa stain × 1000.
FIGURE 8-28 A monocyte (center cell), a lymphocyte (small cell on the right), and a thrombocyte (small cell on the left) in the blood film of a Green Sea turtle (Chelonia mydas); Wright-Giemsa stain × 1000.
Morphologic differences have been detected in monocytes in the same blood sample of reptiles. This has led some to distinguish monocytes, identified as large cells with clear cytoplasm, from azurophils, identified as smaller cells with basophilic cytoplasm.30,55 Similar morphologic variability has also been found in other cell types, such as thrombocytes, eosinophils, and heterophils.44 Although monocytes that have an azurophilic appearance to the cytoplasm are often referred to as azurophils in the literature, the cytochemical and ultrastructural characteristics are often similar to monocytes and therefore should be reported as monocytes rather than as a separate cell type.18,23,36,44,48 Therefore the term azurophil is not recommended when referring to reptilian monocytes. Instead, the term azurophilic monocyte can be used for monocytes with a basophilic cytoplasm and fine azurophilic granulation, if a distinction is desired.
Leukocyte Responses to Disease
Because extrinsic factors, such as season, temperature, and specific stressors, such as capture, restraint, and confinement, can have a marked effect on the leukogram of reptiles, it has been suggested that a total leukocyte count greater than 30,000 cells/μL would be required to indicate an inflammatory response associated with an infection.4,9 However, regardless of the actual leukocyte count, the presence of toxic heterophils indicates a severe inflammatory response that is likely associated with sepsis.
The percentage of heterophils in the leukocyte differential of normal reptiles varies with species. Heterophils can represent up to 40% of the leukocytes in some healthy reptile species.18,19,31,56–59 The concentration of heterophils in the peripheral blood is also influenced by seasonal factors. For example, heterophil concentration is highest during the summer and lowest during brumation.19 Because the primary function of heterophils is phagocytosis, significant increases in the heterophil count of reptiles are usually associated with inflammatory disease, especially microbial and parasitic infections or tissue injury. Heterophil counts are expected to increase with tissue trauma associated with surgical procedures.40 The lack of PER activity in most reptilian heterophils suggests little, if any, oxidative response to stimuli. Noninflammatory conditions that may result in heterophilia include stress (glucocorticosteroid excess), neoplasia, and heterophilic leukemia.
Heteropenia is indicative of either excessive peripheral utilization of heterophils or inadequate heterophil production. For example, heteropenia has been associated with drought and starvation in tortoises that may be physiologically compromised when entering hibernation.60
Heterophils may appear abnormal in reptiles with a variety of diseases. For example, heterophils may exhibit varying degrees of toxicity with inflammatory diseases, especially those involving infectious agents such as bacteria. Toxic heterophils exhibit an increase in cytoplasmic basophilia, abnormal granulation (i.e., dark blue to purple granules or granules with abnormal shapes and staining), and cytoplasmic vacuolation (Figures 8-29 and 8-30). Degranulated heterophils may be associated with artifacts of blood film preparation or may represent toxic changes. Nuclear lobation in species that normally do not have a lobated heterophil nucleus is also an abnormal finding suggestive of severe inflammation.
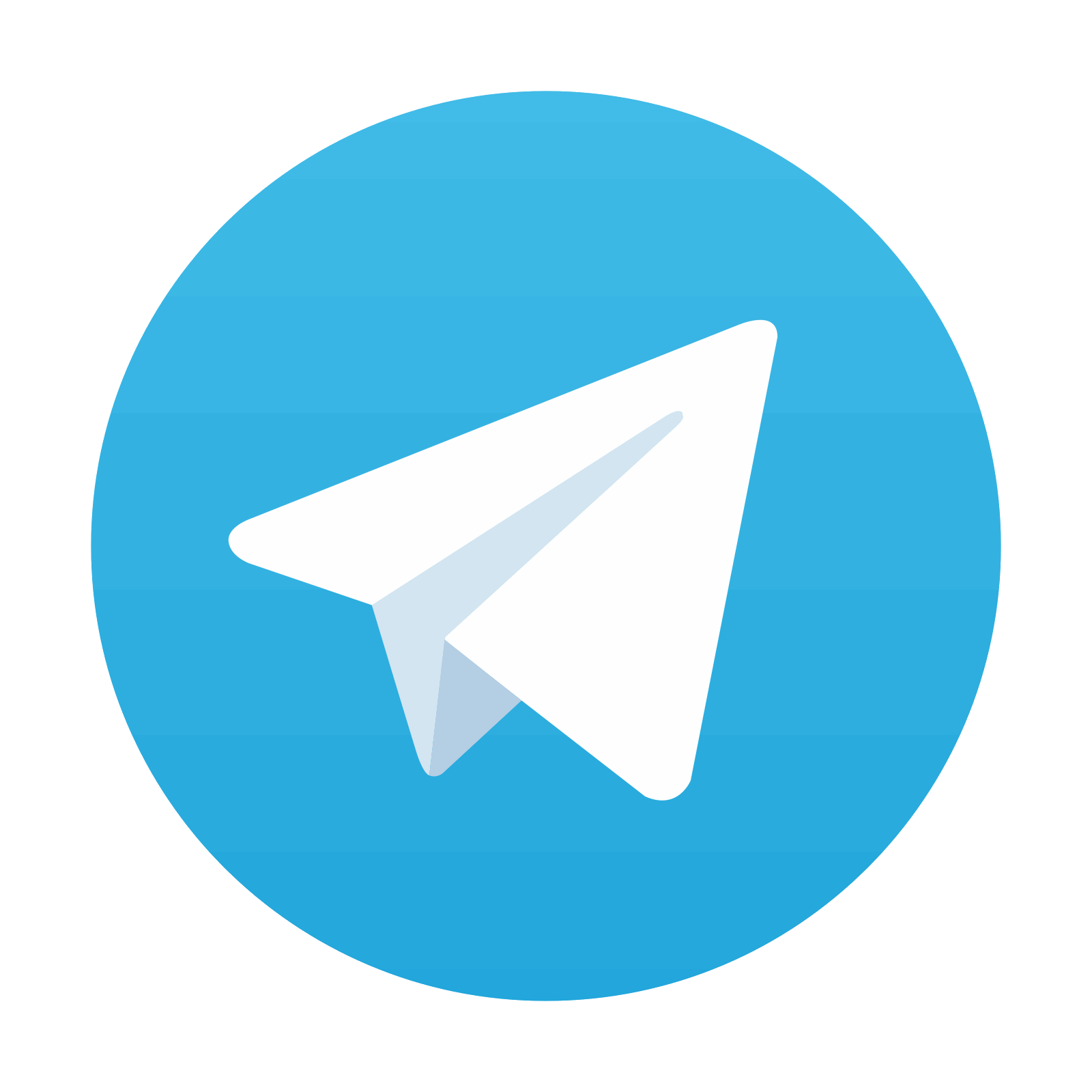
Stay updated, free articles. Join our Telegram channel

Full access? Get Clinical Tree
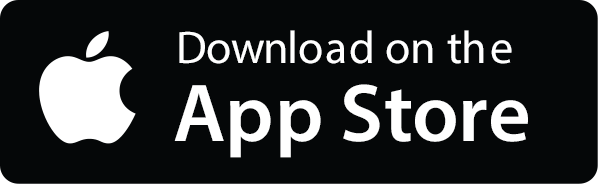
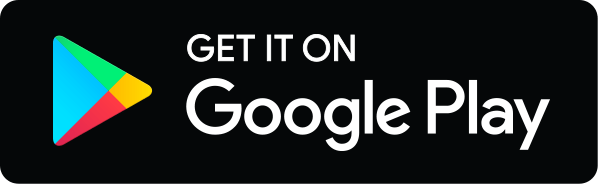