61 Jim E. Riviere Most of this textbook has focused on describing the pharmacodynamics and pharmacokinetics of drugs in animals. In both human medical and companion-animal veterinary practice, the primary concern in drug selection and use is the therapeutic end point, whether or not the drug is efficacious against the disease being treated. Doses are usually administered at label recommendations, and, if greater than label doses are administered, only potential toxicity is of concern. While this line of reasoning is also true to a large degree in food-animal production, veterinarians and producers involved in the treatment of disease in food animals bear the additional concern of the persistence of drug residues in the edible tissues after the disease process has been treated. Adulteration of the food supply with antimicrobial agents, pesticides, environmental contaminants, and other chemicals has been a growing source of concern to the general public and special-interest groups in recent years. The importance of chemical residues in the edible tissues of food-producing animals has been thoroughly reviewed elsewhere (Bevill, 1989; Sundlof, 1989; Van Dresser and Wilcke, 1989; Mercer, 1990; Riviere, 1991, 1992a; Kindred and Hubbert, 1993; Baynes et al., 2015) and has been the topic of a recent text by the author (Baynes and Riviere, 2014). The purpose of this chapter is to acquaint the veterinarian with the legal and regulatory issues concerning the control of drug and other chemical residues in the United States, and to review some of the pharmacokinetic parameters used to determine withdrawal times for drugs and other chemicals in food animals. Drug use in food animal practice is fully discussed in Chapter 52 and the legal regulations are also extensively covered in Chapter 55 of this text. All of these references should be consulted for an in depth analysis of this complex and ever changing topic. The primary parameter used by veterinarians to prevent violative tissue residues is the length of the withdrawal time, or the time required for a drug after dosing to be depleted from the animal before the animal’s meat can be marketed for human consumption. In dairy practice, this is the milk discard time. Recently, government regulation and control as well as adoption of more sensitive multiresidue analytical tools have necessitated more stringent adherence to withdrawal times, and on-site monitoring for many drugs has been instituted. This may have an economic impact on production costs. Unlike in previous editions of this text, withdrawal times will not be tabulated, because they are subject to constant regulatory revision. Extensive tables of tissue depletion pharmacokinetic data are published elsewhere (Craigmill et al., 2006). Current withdrawal times are provided on the Food Animal Residue Avoidance Databank (FARAD) website, available at: www.farad.org. A great deal of concern has been demonstrated over the last 50 years about the presence of chemical adulterants or residues, mainly antimicrobials and pesticides, in the meat, poultry, and milk supplies of the United States. By definition, a chemical residue is either the parent compound or metabolite of that parent compound that may accumulate, deposit, or otherwise be stored within the cells, tissues, organs, or edible products (e.g., milk, eggs) of an animal following its use to prevent, control, or treat animal disease, or to enhance production. Residues can also result from unintentional administration of drugs, or food additives. Finally, accidental exposure to chemicals in the environment can also result in tissue residues. Concerns over food residues are economic as well as public health related. For example, the contamination of milk with antibiotics, most commonly penicillin, can affect starter cultures used to make fermented milk products such as cheeses, buttermilk, sour cream, etc., which can result in economic losses to those processors. From a public-health viewpoint, both the US government and producer associations have taken active roles in minimizing antibiotic residues in meats and milk. Penicillin, for example, is known to induce allergic reactions in some sensitive people, and therefore, penicillin-tainted milk poses a health risk for these individuals. Similarly, chloramphenicol has been reported to induce blood dyscrasias that may lead to death; hence, its use in food-producing animals has been prohibited by the Food and Drug Administration (FDA). The FDA has also prohibited the use of nitrofurans in food-producing animals (excluding the topical routes for administration) because studies have shown them to be carcinogenic. Not only therapeutic drugs but pesticides create residue problems. Most pesticides are administered topically, allowing some amount of percutaneous absorption and sequestration in edible tissues. Lindane has been detected in the fat deposits of sheep dipped in a 0.0125% lindane emulsion 12 weeks after topical exposure (Collett and Harrison, 1963). Other studies have shown lindane residues in sheep, goats (Jackson et al., 1959), and lactating cows (Oehler et al., 1969). In addition to lindane, many common pesticides (organochlorines, organophosphates, botanicals, pyrethrins, etc.) and herbicides used in agriculture today that are applied topically have been shown to produce residues in food-producing animals. Environmental contaminants (e.g., heavy metals, PCB, dioxins, mycotoxins) are also of major concern today. Tissue residue violations detected by governmental monitoring programs have been summarized by Sundlof (1989) as well as Baynes et al. (2015). The USDA Food Safety and Inspection Service (FSIS) manages the National Residue Program (NRP) and yearly publishes sampling plans for the next year (Blue Book) and sampling results for the prior year (Red Book). Both public-health and economic concerns have been the major driving forces in the United States and in other countries behind the search for ways to minimize the threat of residue contamination of the public food supply. The latest editions of these reports (2014, 2015) have served as the source of some NRP details in the present chapter. Contamination of the food supply with chemical residues is rarely an intentional act and usually results either from failure to observe the correct meat withdrawal or milk discard time for a drug after it has been used to treat a disease process in food animals or from accidental contamination of feed by chemicals or drugs. An early study by Van Dresser and Wilcke (1989) provides some interesting insight on drug residue problems in food-producing animals. In that study, streptomycin, penicillin, sulfamethazine, and oxytetracycline were the four most common antibiotics found in tissues, with sulfamethazine being the most commonly found sulfonamide in animal tissues. Long-acting formulations of these drugs (i.e., penicillin and oxytetracycline) had the highest association with violative residues in the animals involved in the study. Injectable drugs were more likely to be associated with residue problems than were feed additives and boluses. Most of these residues were found in veal calves, cows, and market barrows and gilts. The most frequently cited reason for violative residues was failure to observe the correct withdrawal time for the drug. Failure to observe the correct withdrawal time was cited as the most common reason for violative drug residue levels in a study performed by the FDA in the 1970s (Bevill, 1984) and continued to be the most common cause of residue violations in the 1990s. Interestingly, in this study the producer was found to be the responsible party in 80% of the cases investigated for violative levels of drug found in edible tissues (when the responsible party could be identified); unapproved drug use (extralabel drugs) is not considered a major cause of drug residues in animals. Ways to prevent drug residues will be discussed in Section Residue Prevention. The FDA and Environmental Protection Agency (EPA) establish tolerances for a drug, pesticide, or other chemical in the relevant tissues of the food-producing animals. The tolerance is the tissue concentration below which a marker residue for the drug or chemical must fall in the target tissue before that animal’s edible tissues(s) (meat, milk, or eggs) are considered safe for human consumption (Riviere, 1991). The marker residue may be the parent compound, or a metabolite, and reflects a known relationship to the total residues of the drug or chemical (parent and all metabolites), although this has recently been suggested to not be constant but instead sensitive to disease and other pathophysiological conditions seen in target animals ( Lin et al., 2016). The target tissue is an edible tissue, frequently liver or kidney which, when the compound has depleted below the tolerance, assures that all edible tissues are safe for human consumption. Tolerances for different tissues are considered legal end points for which drug withdrawal times are established. Tolerances are established based on assessment of potential hazard of consumption to humans, a topic extensively reviewed elsewhere (Gehring et al., 2006; Baynes and Riviere, 2014). Oral toxicity studies are conducted in animals leading to the determination of an acceptable daily intake (ADI) for the compound in the human diet. These studies consider the compound’s carcinogenic potential, systemic, reproductive, and developmental toxicity, and incorporate various safety factors. Recently, the potential for an antimicrobial compound to induce resistance in bacteria is also factored in. A safe concentration for human consumption is calculated using an equation that accounts for the amount of a specific food consumed by a person representing a high-consuming population (e.g., 19-year-old males) so that a safe concentration of the drug in this food (e.g., meat, milk, eggs) can be established. Various safety factors and statistical considerations are built into these determinations, and a tolerance for this drug in the specific tissue is established where appropriate and published. For example, safety factors reflect the duration of exposure and the nature of the toxic effects associated with the chemical. A teratogen requires a larger safety factor than a nonteratogen, and ADIs based on a short-term subchronic study use a larger safety factor than ADIs based on chronic studies. The tolerance is for a specific drug or chemical entity and reflects both the inherent toxicity of the chemical as well as assumptions about the human consumption of the tissue for which the tolerance is established. Tolerances are determined for the active ingredient (the drug substance or its metabolite) and are not established for the specific formulation of the commercial drug product. A full discussion of this process is presented in the latest FDA Guidance General Principles for Evaluating the Human Food Safety of New Animal Drugs Used in Food-Producing Animals – July, 2016; it has also been reviewed (Baynes et al., 1999; Baynes and Riviere, 2014) and can be found in toxicology or risk assessment texts. It is important for the veterinarian to realize that the end point for determining withdrawal times, the tolerance, is a combined scientific and legal concept and therefore is ultimately controlled by regulatory and not purely scientific and medical practices. The actual withdrawal time appearing on a drug label is also a function of the experimental design that the manufacturer used in the research studies submitted to the FDA for approval. Thus, although the science governing the withdrawal time is based on the pharmacokinetic principles discussed below, the withdrawal time is actually determined based on experimental data. Generally, a drug is administered to healthy animals, groups of the animals are slaughtered at sequential time intervals, and their edible tissues are analyzed for drug concentrations. The withdrawal time is the time from cessation of treatment to the time it takes for the residues of the drug to deplete below the safe concentration. A statistical method (statistical tolerance limit procedure) is used to determine the time at which the marker residue depletes to the tolerance in the target tissue. The method determines the time, rounded up to the next whole day, at which the upper bound of the marker residue tissue concentration is below the established tolerance in the target tissue (where the upper bound is statistically determined to represent with 95% confidence the 99th percentile of the population). Withdrawal times for the FDA-approved drugs for use in food animals are only valid for the specified species, dose, route, and frequency of administration. They are also specific to the manufacturer’s product and formulation; thus, a drug substance (the active ingredient) may have different withdrawal times when present in differently formulated drug products. An analogous process occurs in establishing milk discard times and in determining the withdrawal times for drugs administered to egg-laying poultry (although presently, all drugs approved in the USA for use in laying hens have a 0-day withdrawal). Producers, veterinarians, and other persons involved with chemicals and food-animal production should be acquainted with a few terms and the agencies involved in drug residue control in order to better understand the drug residue problem and how withdrawal times are determined. The use of drugs in veterinary medicine, especially in food-producing animals, is closely regulated in the United States by the FDA under the Department of Health and Human Services. The FDA is charged, through the Federal Food, Drug and Cosmetic Act, with regulating the use of drugs in humans and in animals as well as requiring that the safety and efficacy of a drug be established before the product can be approved for use in animals, including those drugs added to animal feeds. The FDA also regulates human biologics, medical devices, drugs, and food safety. The FDA’s Center for Veterinary Medicine (FDA-CVM) is responsible for the regulation of drugs, medical devices, and feeds intended for animals. The FDA is charged with the responsibility for establishing withdrawal times for drugs and the tolerances of drugs. The FDA and the EPA share responsibility for establishing tolerances for pesticide residues in animal-derived foods. Whereas the FDA establishes safety guidelines for drug use in food animals, it is the responsibility of the USDA to enforce the standards established by the FDA and the EPA. The USDA, through authorization by the Federal Meat Inspection Act and the Poultry Inspection Act, inspects meat and poultry for sale through interstate commerce. The USDA is also authorized to test the tissues of food-producing animals through provisions in the Federal Insecticide, Fungicide and Rodenticide Act to determine whether violative levels of residues of chemicals and drugs are present. The USDA FSIS monitors these tissues through the NRP and identifies problems with drug and chemical residues. The NRP has been in existence for over 30 years and has concentrated on individual as well as population sampling for monitoring and surveying possible residue problems in slaughter animals. In 1992, the NRP estimated they would collect some 350,000 specimens that year to analyze for antimicrobial and pesticide residues (Kindred and Hubbert, 1993). The FSIS is the largest safety inspection force in the federal government (Norcross and Post, 1990). A few years ago, FSIS introduced a new three-tiered monitoring plan and more sensitive multiresidue analytical methods. Sampling occurs in a random commodity-specific national plan, as well as at the production and herd level. The number of samples and selection criteria have changed in 2014–2015 and thus comparisons to previous data may be problematic. There are a number of rapid screening test, once used by FSIS and in producer food safety programs. The FSIS has used several rapid tests for determining contamination of animal products. Among these are the Swab Test on Premises (STOP) for antibiotic and sulfonamide residues, the Overnight Rapid Beef Identification Test (ORBIT) for species identification of meat, the Calf Antibiotic and Sulfa Test (CAST), the Sulfa-on-Site (SOS), and a variety of enzyme-linked immunosorbant assays (ELISAs) (Norcross and Post, 1990). Milk in bulk tanks and from individual animals is assayed for antibiotic residues using various testing strategies: Penzyme III (SmithKline Beecham Animal Health), Spot Test (Cambridge Biotech Corp.), Charm Tests (Charm Sciences, Inc.), and Delvo tests (Gist-Brocades Food Ingredients, Inc.), to name only a few. This area is difficult to adequately summarize because rapid advances in analytical screening methodologies have led to the rapid development of new tests. Other federal government agencies have defined roles in regulating the sale and use of drugs in animals, including the Drug Enforcement Agency (a division of the Department of Justice), the Animal and Plant Health Inspection Service (a part of the USDA), the EPA (mainly for pesticides), and the Department of Transportation. On a state level, the Department of Public Safety, Department of Health, the Animal Health Commission, Boards of Veterinary Medical Licensing, and Pharmacy Boards all have some regulatory influence over the use of drugs in food-producing as well as companion animals. Other legal jurisdictions, including the European Union, have established protocols that should be consulted for details in these countries. As in the USA, these regulations are fluid, end points change based on residue sampling results and new drug submissions. One should always consult the relevant government websites for the latest information. In the USA, the FDA approves new animal drugs for specific indications in a particular species or subclass of animals (e.g., dairy cattle, weanling pigs, etc.). Occasionally, veterinarians may encounter diseases or conditions in animals for which there are no FDA-approved drugs. Under such circumstances, US veterinarians often administer drugs that are not approved for use in food animals or they administer approved drugs in nonapproved ways, practices commonly referred to as “extralabel” usage. Extralabel drug use is defined as the use of a drug in a manner that is inconsistent with its FDA-approved labeling, a practice that, until 1994, was technically illegal. Recognizing that the Food, Drug and Cosmetic Act (FD&C) placed veterinarians in an untenable position of having to choose between providing for relief of animal suffering or complying with the law, the US Congress passed the Animal Medicinal Drug Use Clarification Act (AMDUCA) in 1994. AMDUCA amended the FD&C Act to allow veterinarians under specific conditions to prescribe and administer drugs in an extralabel manner. Table 61.1 lists drugs specifically prohibited from extralabel use in food animals. Under AMDUCA, veterinarians may resort to the extralabel use of approved animal and human pharmaceuticals provided that the following conditions are met: Table 61.1 Drugs prohibited from extralabel use (ELDU) in food animals. Source: Adapted from Food Animal Residue Avoidance Databank, 2016. The following additional conditions must be met for a permitted extralabel use in food-producing animals of an approved human drug, or of an animal drug approved only for use in animals not intended for human consumption: Implicit in these regulations are that the veterinarian makes every effort to first use an approved drug at an approved dosage, that an individual veterinarian–client–patient relationship exists between the producer and the veterinarian, that proper animal identification and documentation be maintained, and that the veterinarian makes every effort to determine an extended withdrawal time for this usage (e.g., consult with a resource such as the Food Animal Residue Avoidance Databank, FARAD), which the producer must adhere to. The regulation prohibits extralabel use for routine production purposes, routine disease prevention, or as feed additives. Pharmacokinetics is the science of quantitating the change in drug concentration in the body over time as a function of the administered dose. Although this discipline has been covered in Chapters 2 and 3 of this text and elsewhere (Riviere, 1992b, 1999, 2011; Baynes and Riviere, 2014), it is important to review some principles here since it serves as the basis for understanding withdrawal times. How a drug or combination of drugs behaves in the body after administration not only is important from a therapeutic point of view but is of paramount importance to the producer and veterinarian in order to prevent residues in the edible tissues after the disease process has been resolved and the animal is slaughtered. For therapeutic usefulness and drug residue determinations, a known amount of drug is administered to a healthy animal. Serum concentration data are collected and mathematical models are created so that the overall disposition of the drug in the body can be evaluated in relation to absorption, distribution, metabolism, and elimination. Parameters for these models are estimated by fitting regression lines to the observed serum concentration versus time profiles, an example of which is shown in Chapter 3, Figure 3.15. The slopes of the three lines shown in Figure 3.15, when plotted on a semilogarithmic plot, represent distribution (λ1), short-term elimination (λ2), and long-term elimination (λ3) from the body. The λ1 (or α) and λ2 (or β) phases of the serum concentration versus time profile are the only phases that are usually present when serum concentrations are monitored over a short period of time after dosing and are typically used to predict therapeutic drug concentrations. When serum concentrations are monitored for longer periods of time after drug administration using more sensitive analytical assay methods, however, the λ3 (or γ) phase of elimination appears for certain compounds. This can be present for several days or several months after the last administered dose depending on the drug’s physicochemical properties, the amount of drug administered, and the species in which it was administered. This λ3 phase reflects drug disposition in the so-called “deep compartments.” Alternatively, for prolonged-action depot preparations (e.g., benzathine penicillin), this terminal phase may actually reflect the rate-limiting absorption phase (“flip–flop” phase). For purposes of determining withdrawal times of drugs that may be used in food-producing animals, we will focus our discussion on the terminal phase of drug elimination to determine the half-life (t1/2) of the drug in the body, since this is the measurement that is relevant in determining drug withdrawal times. In the actual research studies conducted to determine a withdrawal time, it is the half-life of drug in the specific tissue that is of paramount importance. This information is not available to the veterinarian. Additionally, tissue pharmacokinetics are exceedingly complex, and a discussion here of terminal half-lives will be sufficient to illustrate the concepts involved. For more in-depth information on the clinical application of pharmacokinetics and how pharmacokinetic parameters can affect clinical parameters, readers should consult Chapters 2 and 3 of this textbook. The half-life of the drug or chemical in the body is the primary biological measurement used to determine withdrawal times of drugs and chemicals in food-producing animals; however, this parameter can be influenced by many biological factors (Baynes and Riviere, 2014; Baynes et al., 2015). The t1/2 of a drug by definition is the time is takes for 50% of the drug in the animal to be eliminated from the body and is based on the terminal slope of the elimination curve used to determine the withdrawal time of a drug. The t1/2 is calculated using the equation
Chemical Residues In Tissues Of Food Animals
The Concern Over Residues in Food
Regulation of Drug Residues in Animals
The Veterinarian and Extralabel Drug Use
Drugs with no allowable extralabel uses in any food-producing animal species
Drugs with restricted extralabel uses in food-producing animal species
Pharmacokinetics and Residues
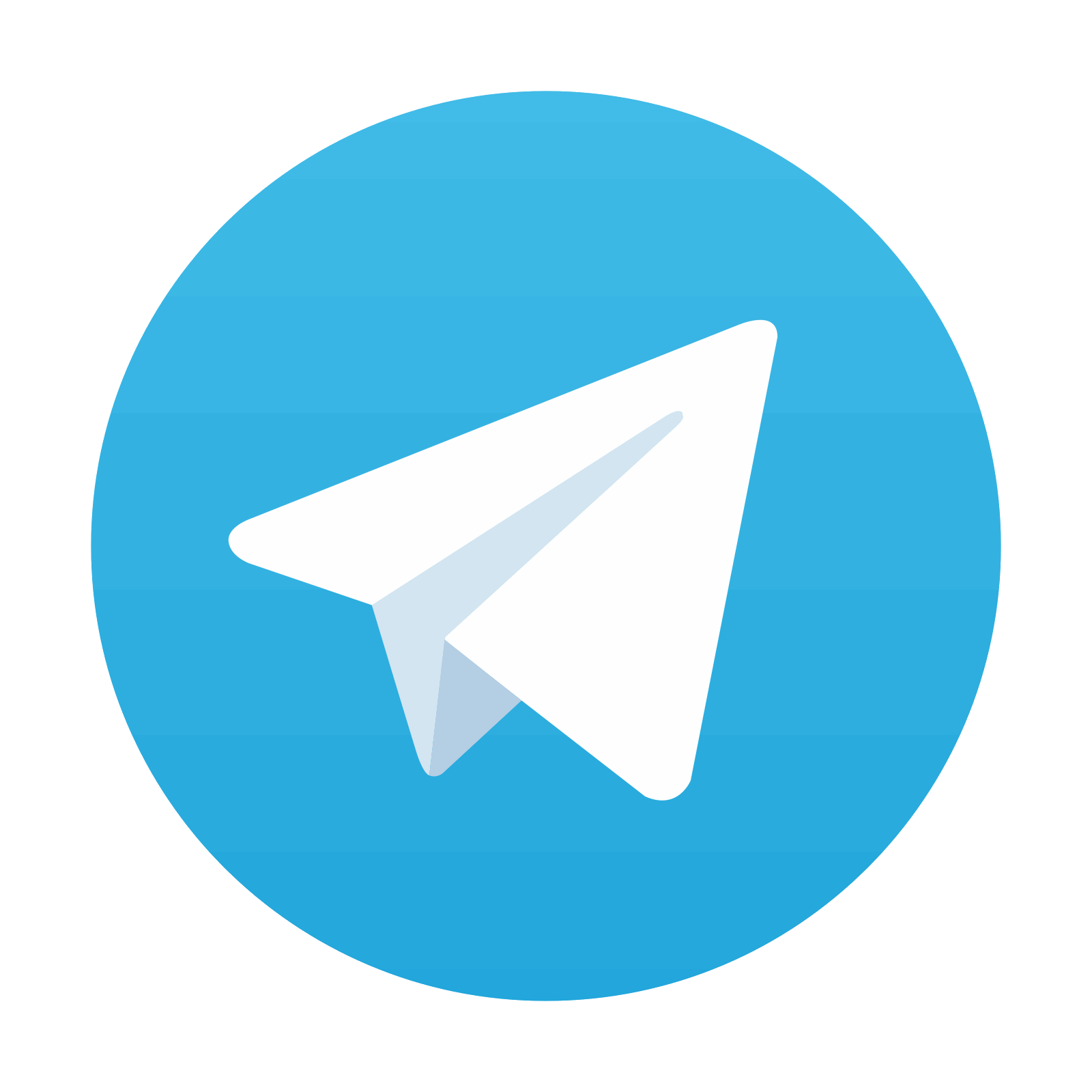
Stay updated, free articles. Join our Telegram channel

Full access? Get Clinical Tree
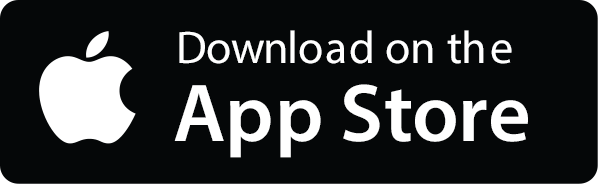
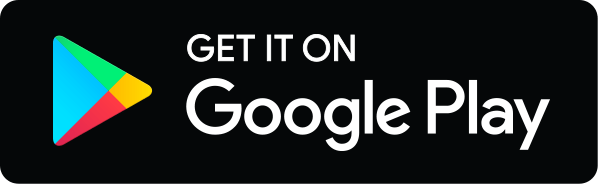