- The family Anatidae, subfamily Anatinae, for example the mallard duck, shoveller, eider ducks and shelducks
- The family Anatidae, subfamily Anserinae, Tribe Anserini (swan and true geese), for example the mute, Whooper’s and Bewick’s swans, barnacle and greylag geese
- The Falconidae family for example the peregrine falcon, the saker, the llanner, the gyrfalcon
- The Accipitridae family such as the buzzards (common, rough-legged and honey), the sparrowhawk, goshawk, golden eagle
The Psittaciformes are among the most colourful of birds kept as pets.
Nervous system
The avian brain is extremely smooth, lacking the many gyri (the ridges in the brain) seen in mammals (Figure 9.1). Sight appears to be the dominant sense in most birds. Two large optic lobes lie between the cerebral hemispheres and the cerebellum, and it is here where the optic nerves communicate and disseminate information. There is no corpus callosum, the cerebral cortex is generally very thin but the corpus striatum is well developed and is thought to be the site of mental association in birds. An important feature of the bird’s brain is the pineal body which sits in the diencephalon, cranial to the cerebellum in midline dorsally. The pineal body has secretory cells similar to photoreceptors and so will respond to light. They are linked also via the cranial cervical ganglia to the optic nerve. The pineal body is responsible for regulating many seasonal effects such as reproduction and migration as well as circadian rhythms. It has a direct effect via hormone secretion onto the hypothalamus.
The avian nervous system is not dissimilar to that seen in its mammalian counterpart. Birds possess 12 cranial nerves (CN), the same number as in cats and dogs. In birds, the optic nerve (CN II) is the largest cranial nerve, being almost half the diameter of the spinal column. It passes through the calvarium via a single hole rather than through multiple smaller ones associated with the cribriform plate of mammals. The other 11 cranial nerves have similar functions as seen in mammals, and the reader is referred to more in-depth anatomy texts for more information (King & McLelland, 1975; Bennett, 1994).
Each of the wings has a nervous supply from a brachial plexus derived from the spinal nerves in the caudal cervical area. There are three nerve plexuses in the lumbosacral region: lumbar, ischiatic and pudendal. The lumbar plexus derives from the last two lumbar and the first one to two sacral spinal nerve roots. Like the other lumbosacral plexuses, it lies in a hollow of the pelvis, dorsal to the cranial kidney area. It supplies the body wall and upper leg muscles and gives rise to the obturator, femoral, cranial gluteal and saphenous nerves. Unlike dogs and cats, birds have an ischiatic plexus which is derived from four to seven spinal nerves in the sacral area and which is situated in a hollow of the pelvis dorsal to the mid-kidney structure. It gives rise to the principal nervous supply for the hindlimbs – the ischiatic nerve which is the largest peripheral nerve in the body and the caudal gluteal nerve. Finally, a pudendal plexus forms in a hollow of the pelvis dorsal to the caudal kidney area from five coccygeal spinal nerves and innervates the tail and cloacal area.
Musculoskeletal system
Most birds have the power of flight. The dense, cumbersome bones of the earthbound mammal would require too much effort to lift into the air. Birds have therefore adapted their skeletal structure, simplifying the number of bones by fusing some together, and generally lightening the whole structure by creating air spaces within many of the bones.
To further lighten the skeleton, several of the larger bones, and even some of the vertebrae in the spine, are connected directly or indirectly to the airways, and are said to be pneumonised. This replaces the thick medullary cavity or bone marrow present in the centre of mammalian bones, and produces a light, trabecular structure. Whilst light, the structure is nevertheless extremely strong.
Figure 9.2 shows a generalised avian skeleton.
Skull
Beak
The beak, or bill, is the principal feature of the avian skull. It has been modified into a bewildering number of shapes and sizes, depending mainly on the diet to which the bird has become adapted. In all cases it is composed of an upper (maxillary) and lower (mandibular) beak which are covered in a layer of keratin, a tough protein compound similar to that which forms the exoskeleton of insects. This keratin layer is known as the rhamphotheca. It is further classified so that the maxillary layer is referred to as the rhinotheca, and the mandibular layer as the gnatotheca. The rhinotheca and gnatotheca grow from a plate at the base of the respective sides of the beak, the rate of replacement depending upon the type of food eaten and the abrasion the beak receives.
In Psittaciformes (Table 9.1), the upper beak is powerfully developed and ends in a sharp point overhanging the broader, stouter lower beak. The tremendous power in a parrot’s beak is due to a synovial joint or hinge mechanism, known as the kinetic joint, which joins the beak to the skull. The parrot’s lower beak has a series of pressure sensors at its tip, which allow it to test the consistency and structure of objects grasped.
In raptors, the upper beak is extremely sharp and pointed, but lacks the kinetic joint attachment so it cannot produce such powerful downward force. Instead, it is used as a ripping instrument.
In Anseriformes (the duck family), the beak is flattened and may have fine serrations at the edges which allow the bird to filter fine particles from the water. Ducks such as mallards and shovellers have this type of beak. These serrations may be further developed to a jagged edge (e.g. in the aptly named sawbill family) which allows the bird to grip slippery food, such as fish. Anseriformes also have nerve endings in a plate at the tips of their beaks (known as the ‘nail’) which allow them to find food hidden in mud.
In all birds there is a series of smaller bones behind the lower and upper beaks which allow them to move the beak independently of the skull. These include the palatine, quadrate and pterygoid bones and the jugal arches. Their exact movements are beyond this text to describe, but many of the references at the end of this chapter give good accounts of their function.
Nostrils
The nostrils, or nares, lie at the base of the upper beak in most birds and are often surrounded by an area of featherless skin known as the cere. This may be highly coloured in some species, such as the budgerigar, where they may be used to identify the sex of the bird. In many Anseriformes the nares lie more towards the tip of the beak. The nares themselves are merely openings into the sinus chambers, which in turn connect with a branching network of bony chambers throughout the bird’s head. These sinuses vary according to the species, but the majority of avian patients have an infraorbital sinus. This sits below the eyes, and is often involved in sinus and ocular infections. It differs from sinuses seen in most mammals in that the lateral wall has no bone, being covered by soft tissue only. This means any infraorbital sinus infection often results in swelling on the face of the bird ventral to the eye. These sinuses also communicate with head and neck air sacs. The function of these air sacs is not clear, but they may help with voice resonance. When a bird suffers from sinus infections, the narrow inlets to these sinuses may become partially blocked and act as one-way valves, allowing air into the sacs but not out. The sacs may then overinflate, and soft swellings are then commonly seen over the back or nape of the bird’s head.
The sinuses and external nares communicate with the oropharynx via the choanal slit. This is a narrow opening in the midline of the hard palate and is sited immediately over the glottis when the beak is closed, allowing the bird to breathe through its nostrils. It is often the area chosen for taking samples when trying to isolate infectious agents for upper airway disease in birds.
The skull of the avian patient connects to the atlas (or first spinal vertebra) via only one occipital condyle at the base of the skull, unlike the mammalian two. There are also a large number of highly mobile cervical vertebrae. These two factors make the avian head extremely agile. However, the atlanto-occipital joint is also a weak point, making dislocation at that site very easy.
Vertebral column
Cervical vertebrae
The cervical vertebrae (Figure 9.2) are independently mobile in the avian patient, as they are in the mammalian patient, and vary in number depending on the species between 11 and 25. They are generally box-like in form.
Thoracic, lumbar and sacral vertebrae
The thoracic vertebrae (Figure 9.2) are fused in raptors, pigeons and many other species to form a single bone known as the notarium. In other species they have some limited mobility. There are then two intervertebral joints between the notarium and the fused lumbar and sacral vertebrae. These fused vertebrae are known as the synsacrum. The synsacrum fuses with the pelvis itself to form a dorsal shield of bone over the caudal aspect of the bird.
Coccygeal vertebrae
The majority of the caudal coccygeal vertebrae (Figure 9.2) are usually fused into a single structure known as the pygostyle – which forms the ‘parsons nose’ part of the chicken!
Pelvis
The roof of the pelvis is formed by the synsacrum (Figure 9.2). The two ‘sides’ of the pelvis are reduced in size compared with mammals but consist of the iliac and ischial bones, with the acetabulum being created where they meet. The acetabulum in birds is not a complete bony socket as it is in mammals, but a fibrous sheet. There is a ridge on the lateral pelvis known as the antitrochanter, which articulates with the greater trochanter of the femur. The function of this ridge is to prevent the limb from being abducted when perching. The pubic bones of the pelvis do not fuse in the ventral midline as in mammals. Instead they form fine long bones which extend caudally towards the vent. They provide support for the skin covering the caudal abdomen and enough space for the passage of eggs in the female bird.
Ribcage
Psittaciformes have eight pairs of ribs (Figure 9.2). Each rib has a dorsal segment known as the thoracic rib, and a ventral segment, or sternal rib. These ribs point backwards and rigidly connect the thoracic vertebrae dorsally and the keel, or sternum, ventrally.
Sternum
The sternal vertebrae are fused in birds to form the keel. The keel has a midline ridge which divides the pectoral muscles into right and left sides. The ridge may be a deep structure, as is seen in pigeons, raptors and Psittaciformes, allowing large pectoral muscles to attach for strong flight. Alternatively, the keel may be flattened, as with Anseriformes, to provide a boat-like structure more suited to floating.
Wings
The shoulder joint is formed by the meeting of three bones: the humerus, the scapula (which is more tubular than the flattened mammalian one) and a third bone known as the coracoid (Figure 9.2). This latter bone forms a strut propping the shoulder joint against the sternum. The supracoracoid muscle attaches to the keel, then passes through the foramen, or opening, formed at the meeting point of these bones, and so reaches the dorsal aspect of the humerus where it attaches. Contraction of this muscle, along with some elastic tissues which are also present, helps to raise the wing. The pectoral muscles attach from the keel onto the humerus to pull the wing downwards. The fused clavicles, or wishbone (often referred to as the furcula), articulate with the coracoid bone and provides a degree of spring to the flapping of the wings. The humerus is pneumonised, which means that it cannot be used for intraosseous fluid therapy. This is also an important point to consider when repairing fractures.
The humerus articulates with the radius and ulna at the elbow joint. The radius is the smaller of these two bones and lies cranially. The ulna provides the source of attachment for the secondary flight feathers, which insert directly into the periosteum of this bone (Figure 9.3). The ulna is often used for intraosseous fluid administration in birds.
Figure 9.3 Ventral aspect of a kestrel’s (Falco tinnunculus) wing with covert feathers removed showing the attachment of the primaries to the manus and the secondaries to the ulna.
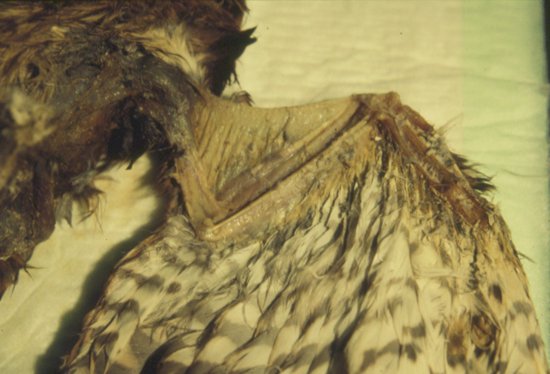
The radius and ulna articulate with one radial carpal bone and one ulnar carpal bone, respectively. These in turn articulate with three metacarpal bones. The first metacarpal bone is the equivalent of the avian ‘thumb’. It is known as the alula, or ‘bastard wing’, and forms a feathery projection from the cranial aspect of the carpometacarpal joint. The remaining two metacarpal bones are known as the major and minor metacarpal bones, and articulate with the first phalanx cranially and the minor digit caudally. The first phalanx then articulates with the second phalanx, forming the wing tip. The primary feathers attach to the periosteum of the phalanges and minor metacarpal bones (Figure 9.3).
The area of the wing is enlarged by thin sheets of elastic tissue which span from one joint surface to another. The largest extends from the shoulder to the carpal joint cranially and is known as the propatagium or ‘wing web’ (Figure 9.4). This can be used in some species, such as pigeons, for vaccine administration.
Figure 9.4 Dorsal aspect of a kestrel’s (Falco tinnunculus) wing with covert feathers removed showing the elastic sheet of the propatagium bridging the elbow joint.
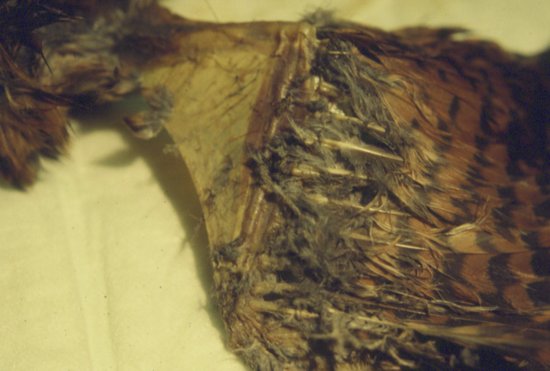
Pelvic limb
The acetabulum of the pelvis holds the femoral head (Figure 9.2). The limb may be locked, and prevented from being abducted, by the greater trochanter of the femur engaging with the antitrochanteric ridge on the pelvis. The femur is pneumonised in many birds. At the stifle joint, the femur articulates with the patella and the tibiotarsal bone. The tibiotarsal bone is so called because it is formed from the fusion of the tibia and the proximal row of tarsal bones, and may also be used for intraosseous fluid administration. On the lateral aspect of the proximal tibiotarsus is the much reduced fibula.
Distally, the tibiotarsal bone articulates with the tarsometatarsal bone. This bone is formed by the fusion of the distal row of tarsal bones with the solitary metatarsal bone. The joint between the tibiotarsus and the tarsometatarsus is known as the intertarsal, or suffrago, joint. The tarsometatarsus then articulates with the phalanges.
In Psittaciformes, two digits point forwards (the second and third) and two backwards (the first and fourth), creating a zygodactyl limb. The first digit has two phalanges, the second digit has three phalanges, the third has four phalanges and the fourth has five phalanges. In perching birds (Passeriformes) and raptors, the second, third and fourth digits point forwards and the first points backwards creating an anisodactyl limb. Some species, such as the osprey (Pandion haliaetus), may move the fourth digit to face forwards or backwards to aid capturing its prey, creating a semi-zygodactyl limb.
Special senses
Eye
The avian eye is unique in that it contains a series of small bones. These are known as the scleral ossicles (Figure 9.2). They form a ring-shaped structure which supports the front of the eye. The avian eye also differs from the mammalian eye in that it is not a globe, but pear-shaped, with the narrower end outermost.
The avian eye is large in proportion to the overall size of the skull, with only a paper-thin bony septum separating the right and left orbits. Birds have a mobile, translucent third eyelid, and upper and lower eyelids, the lower of which is more mobile than the upper. Two tear-producing glands commonly exist: the third eyelid, or Harderian gland, which is located at the base of the third eyelid, and the lacrimal gland situated caudolaterally, as in mammals.
The colour of the iris may change with age in some parrots, for example the African grey parrot has a dark grey iris until 4–5 months of age, when it turns yellow-grey, and then silver as it continues to age. In others the iris may be used as an indicator of the sex of the bird: in large cockatoos, for example, the female has a bright, red-brown iris, whereas the male’s is a dark, brown-black.
The avian retina is thick and possesses no visible surface blood vessels, unlike that of mammals. To provide nutrition to the retina, birds possess a pleated and folded vascular structure called the pecten oculi, which is found at the point where the optic nerve enters the eye. It contracts intermittently, expelling nutrients into the vitreous humour.
Finally, the avian iris has skeletal-muscle fibres within it, unlike mammals which possess only smooth-muscle fibres. This means the avian patient can constrict and dilate its pupil at will, so reducing the value of the pupillary light reflex as a tool in determining ocular function. Because the two optic nerves are completely separated from each other, the consensual light reflex is also a poor indicator of cerebral function.
Ear
There is no pinna in birds, although some species, such as the long- and short-eared owls, have feathers in this area. There is a short, horizontal external canal, covered by feathers, which is located caudolateral to the ocular orbit. The tympanic membrane may be clearly seen. The middle ear connects to the oropharynx via the Eustachian canal. The mammalian aural ossicles are replaced in the bird by a lateral, extra columella cartilage and a medial columella bone which transmit sound waves to the inner ear.
The inner ear contains the cochlea and the semicircular canals, which fulfil the same functions as in mammals.
Respiratory anatomy
Upper respiratory system
The nares open into the nasal passages, which in turn communicate with the glottis of the larynx via a midline aperture in the hard palate which forms the roof of the caudal mouth. This aperture is called the choanal slit. The sinus system and cervicocephalic air sacs have been previously mentioned.
Larynx
Birds have a reduced laryngeal structure, lacking an epiglottis, the thyroid cartilage, and the vocal folds seen in cats and dogs. The main structure is the glottis, which protects the entrance to the trachea. External muscles pull the glottis and trachea forwards so that it communicates directly with the choanal slit, allowing the bird to breathe through its nostrils. The glottis is held closed when at rest, only opening on inspiration and expiration.
Trachea
The trachea of avian species differs from the mammalian trachea in that its cartilage rings are complete, signet-ring-shaped circles, interlocking one on top of the other, rather than the C-shaped rings of the mammalian trachea. In Psittaciformes and diurnal raptors, the shape of these cartilage rings is slightly flattened in a dorsoventral direction, whereas in most Passeriformes they are round.
In some species, such as the Whooper’s swan and the guinea fowl, the trachea forms a series of loops and coils at the thoracic inlet. Other species, such as the emu, have a midline ventral split in the trachea three quarters of the distance between the head and the thoracic inlet. The tracheal lining mucosa projects through this slit to form a tracheal sac. This improves vocal resonance. In male ducks, such as mallards, there is a swelling in the last portion of the trachea, often just inside the thorax, known as the tracheal bulla.
Syrinx
Before the trachea divides into the two main bronchi, there is a structure known as the syrinx (Figure 9.6). This is where the bird produces most of its voice. It is composed of a series of muscles and two membranes which can be vibrated, independently of inspiration or expiration.
Lower respiratory system
Lungs
The lungs of avian species are rigid in structure and do not inflate or deflate significantly. They are flattened in shape and firmly attached to the ventral aspect of the thoracic vertebrae and vertebral ribs. There is no diaphragm in birds, and the common body cavity is referred to as the coelom.
The paired bronchi are supported by C-shaped rings of cartilage, unlike the trachea. The primary bronchi supply each of the two lungs, and rapidly divide into secondary and tertiary bronchi, or parabronchi. There are four main groups of secondary bronchi supplying the lung but their role in gas exchange is minimal. The tertiary bronchi, however, do play a role in gas exchange, as their walls are filled with membranes capable of gaseous exchange. These areas appear as small pits, or atria, to which are connected even finer tubes known as air capillaries. These intertwine with each other to form a three-dimensional mesh interwoven with the blood capillary beds. These air capillaries vary in size but average around 3–5 mm in diameter. This extremely small diameter produces very high forces of attraction between their walls when fluid secretions are present, resulting in rapid blocking of the respiratory surfaces. To stop this from occurring, there are cells within the parabronchi which secrete surfactant, to ensure the airways stay open.
The lung structure may be further classified by the direction of airflow within it into the neopulmonic lung and the paleopulmonic lung. These will be mentioned later on when discussing respiratory physiology.
Air sacs
The final part of the avian lower respiratory system is composed of the air sacs (see Figure 9.5). These are balloon-like sacs which act as bellows, pumping the air into and out of the rigid avian lungs in response to movements of the body wall and sternum. The air-sac walls are very thin and composed of simple squamous epithelium which covers a layer of poorly vascularised elastic connective tissue.
Figure 9.5 Avian air-sac system in a duck: (a) nasal passages; (b) infra-orbital sinus; (c) cervicocephalic air sacs (single); (d) clavicular air sacs; (e) cranial thoracic air sacs; (f) caudal thoracic air sacs; and (g) abdominal air sacs. H, heart; L, liver; Lu, lungs; B, syringeal bulla (male ducks).
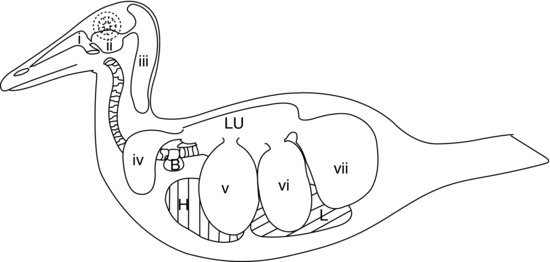
In the majority of birds there are nine air sacs. One of these is the separate air sac already mentioned, the cervicocephalic air sac, which does not communicate with the lungs at all. The other eight all communicate with the lungs via a secondary bronchus (except the abdominal air sacs which connect to the primary bronchus on each side). Figure 9.5 shows the air sac system of a duck.
In addition to the separate cervicocephalic air sac, the other standard eight air sacs are
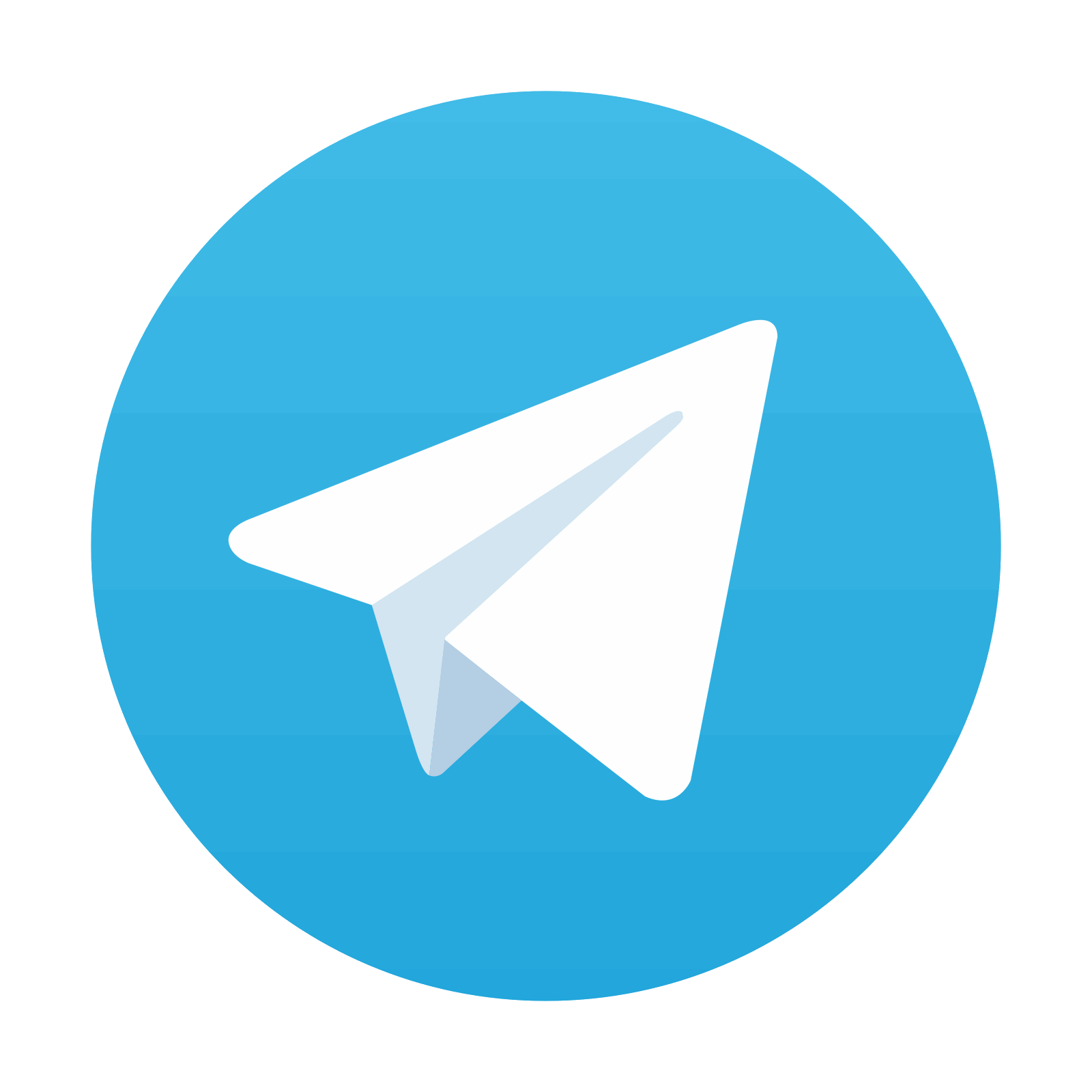
Stay updated, free articles. Join our Telegram channel

Full access? Get Clinical Tree
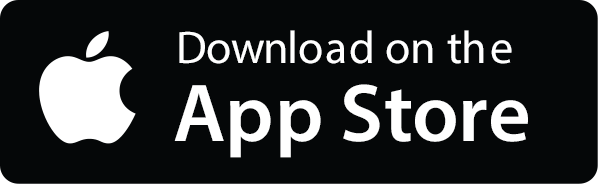
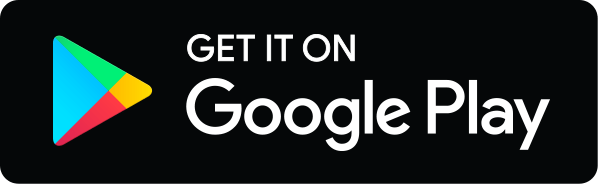