Joachim Frey Bacillus anthracis, a spore‐forming Gram‐positive pathogenic bacterial species, is the etiological agent of anthrax. Anthrax has a long record as a life‐threatening infectious disease of animals and humans worldwide (World Health Organization 2008). It primarily infects herbivores, since they are most frequently exposed to the pathogen in the environment, but it can affect most other mammals, as well as certain avian species. B. anthracis still causes severe losses in cattle breeding in certain parts of the world. However, vigorous control measures, particularly strict prohibition of burial of carcasses and slaughter waste, as well as large animal vaccination programs during the first part of the twentieth century, have significantly reduced the incidence of anthrax in large parts of the world. B. anthracis belongs to the phylum Firmicutes, the family Bacillaceae, the genus Bacillus and the Bacillus cereus group. The latter consists of eight spore‐forming, aerobic, facultative anaerobic, rod‐shaped species: B. anthracis, B. cereus, Bacillus mycoides, Bacillus pseudomycoides, Bacillus thuringiensis, Bacillus weihenstephanensis, Bacillus cytotoxicus, and Bacillus toyonensis (Guinebretière et al. 2013; Okinaka and Keim 2016; Ehling‐Schulz et al. 2019). Phylogenetically, they are closely interrelated but show distinct differences in pathogenicity, clinical symptoms, host preference, and ecological niches. Most of these species are of economic, environmental, and medical importance and are of concern in biodefence. The chromosomes of the different members of the B. cereus group show a high level of synteny and protein similarity with little difference in gene content. The different species of the B. cereus sensu lato group, in particular their housekeeping genes, are genetically closely related considering this group previously as a phylogenetic “single species” (Helgason et al. 2000). However, host species predilection, clinical manifestation and ecological niches distinguish the different species that are included in this group (Okinaka and Keim 2016). In particular, analyses of the gene flow across the B. cereus sensu lato group revealed that B. anthracis is clonal and discerning from the other species (Didelot et al. 2009). Furthermore, B. anthracis infections show distinct clinical symptoms that differ from infections with other species of the B. cereus sensu lato group, with the exception of B. cereus biovar anthracis (Klee et al. 2006, 2010; Antonation et al. 2016). The main virulence attributes of the different species of the B. cereus sensu lato group are host specific toxins and capsular carbohydrates that allow bacteria to avoid clearance by the host’s immune defense. Most of them are encoded on plasmids, which define host specificity and consequently delineate the bacterial species. The identification of individual species of the B. cereus sensu lato group is therefore complex, and some isolates within a species display unusual biochemical and/or physiological properties that confound their accurate differentiation. Phylogenetically, the B. cereus sensu lato group of bacteria divides into five distinct clades that show partial barriers to interclade gene flow. Strains of B. anthracis are highly clonal and restricted to clade I together with the highly virulent B. cereus biovar anthracis, which produces anthrax toxins. Clade II contains other virulent B. cereus strains including emetic toxin‐producing B. cereus strains specified by the cereulide synthetase and the insect pathogen B. thuringiensis. Clade III contains environmental B. weihenstephanensis, mostly non‐pathogenic and psychrotolerant, B. cereus, B. mycoides and some strains of B. thuringiensis. Clade IV contains a distinct cluster of B. cytotoxicus as well as a second cluster with strains of B. pseudomycoides, B. mycoides, B. cereus, and B. thuringiensis. Clade V includes B. toyonensis and strains of B. cereus and B. thuringiensis (Helgason et al. 2000; Priest et al. 2004; Didelot et al. 2009; Ehling‐Schulz et al. 2019). B. toyonensis was characterized for probiotic properties and is used as such in animal feed (Jiménez et al. 2013). In its vegetative form, B. anthracis is rod shaped of approximately 4 × 1 µm, often appearing in short chains from the blood of affected animals, or in long chains from blood agar cultures. On blood agar B. anthracis presents as non‐hemolytic, dull, gray‐white colonies with curly edges. It is sensitive to penicillin and to phage gamma (γ‐phage). These characteristics were used formerly as species confirmation. Surprisingly, B. anthracis contains two bla genes encoding β‐lactamases that normally cause penicillin and ampicillin resistance. However, in B. anthracis, both bla genes are silenced by the repressor RsiP repressing sigma factor SigP expression, which otherwise would enable transcription of the two bla genes (Ross et al. 2009). The rare strains of B. anthracis or anthrax‐like B. cereus biovar anthracis in contrast are highly resistant to penicillin and moderately resistant to oxacillin and cephalothin. They emerged most likely by de‐repression of the sigma factor SigP that transcribes in these strains one or both of the otherwise silent β‐lactamases in B. anthracis (Ross et al. 2009; Pilo et al. 2011). The most characteristic features of B. anthracis are the two binary anthrax toxins, the lethal factor (LEF) encoded by gene lef and the edema factor (EF), an adenylate cyclase, encoded by cya. They share the common adhesin subunit termed the protective antigen (PAG, encoded by pag). Genes lef, cya and pag are specified by virulence plasmid pXO1. A further major virulence factor, the capsule (CAP) is encoded by the capsular biosynthesis genes capABCDE on a separate virulence plasmid pXO2. These factors mainly determine the disease anthrax. They are genetically highly conserved which makes them a prominent target set for identification by polymerase chain reaction (PCR; Ramisse et al. 1996; Zincke et al. 2020). Currently, specific PCR tests targeting the genes of the two toxins and the capsule located on the virulence plasmids and occasionally a chromosomal marker are used for rapid confirmation of the species B. anthracis and distinction from other Bacillus species, which is essential for medical and epidemiological purpose (Ramisse et al. 1996). Although anthrax is generally considered as a monoetiologic disease, anthrax‐like diseases in humans and animals caused by non‐B. anthracis or atypical B. anthracis strains have been reported since 2004. The strains exhibited a combination of phenotypic traits of B. anthracis and genetic background of B. cereus and were named B. cereus biovar anthracis (Hoffmaster et al. 2004, 2006; Klee et al. 2006; Antonation et al. 2016). Strains from the United States were isolated from humans, while the strains isolated in Africa were mostly from wild and occasionally domesticated animals (Hoffmaster et al. 2004, 2006; Leendertz et al. 2004, 2006; Klee et al. 2010; Pilo et al. 2011; Antonation et al. 2016). B. cereus biovar anthracis from Africa were shown to harbor virulence plasmids that are very similar to pXO1 and pXO2 that were detectable by PCR for the respective virulence gene markers pag, lef, cya, and cap (Klee et al. 2010; Antonation et al. 2016). Figure 27.1 Schematic genetic tree summarizing the phylogeography of worldwide Bacillus anthracis strains. The three major clades A, B, and C are shown in different colors. (Note that the clades A, B, and C from B. anthracis are different from the clades I–V of B. cereus sensu lato.) The first capital letter of the different canonical single‐nucleotide polymorphism groups indicate the clade followed by the indicators of the lineages. Lineage A.Br.TEA.011 is indicated by an oval line to highlight the close relation between European, African and Northwestern American sublineages. Subbranches of the B.Br.CNEVA lineage with their geographic locations are given to illustrate the close geographic clustering. Branch lengths are not scaled and bootstraps are not given in this figure. Source: Data compiled from Keim et al. (2004), Kenefic et al. (2009), Derzelle et al. (2011, 2015a, 2015b, 2016a, b), Girault et al. (2014a), Vergnaud et al. (2016). In contrast, American strains seem to have a pXO1‐like plasmid named pBCX01 but not a pXO2‐like plasmid (Hoffmaster et al. 2004, 2006; Marston et al. 2006). Instead, they possess a second plasmid, pBC218, which carries the bpsX‐H operon encoding for a specific tetrasaccharide capsule (Oh et al. 2011; Scarff et al. 2018). In addition, these strains contain the genes hasABC encoding for the biosynthesis of a hyaluronic acid capsule on plasmid pBCXO1, together with the variants of the toxin genes, pagA1, lef1, cya1 (Oh et al. 2011). Interestingly, an inactive hasACB operon is also present in the pXO1 plasmid of B. anthracis. During infection with North American B. cereus biovar anthracis strains, both hasACB and bpsX‐H capsules are essential for the establishment of anthrax‐like disease and resistance to phagocytosis. In contrast, African B. cereus biovar anthracis strains produce a poly‐γ‐D‐glutamic acid (PGA) capsule specified by the pXO2‐like plasmid in addition to the hyaluronic acid capsule (Klee et al. 2006; Antonation et al. 2016). Remarkably, curing of the pXO2‐like plasmid in these strains did not significantly alter their virulence in mice and guinea pigs since supposedly the hyaluronic acid capsule is sufficient for virulence (Brézillon et al. 2015). Anthrax‐causing B. cereus biovar anthracis in Africa is widely present and persistent in tropical rainforests where it is responsible for causing death to a wide range of mammalian hosts and constitutes a particular threat to local chimpanzee populations (Hoffmann et al. 2017), in contrast to B. anthracis that is largely limited to arid ecosystems affecting mostly bovine species. B. anthracis spread worldwide, generally by two different ways: (i) a relatively slow process by migration of human populations with their domesticated cattle; and (ii) a rapid way by trade of animal products, mostly wool from contaminated rural areas to industrialized countries that possessed wool‐processing factories. Both ways could be documented by analyzing hundreds of historic as well as new strains of B. anthracis isolated from infected humans, perished cattle or occasionally from environment and wool samples (Pilo et al. 2008). Bacterial genomes are dynamic. Various genetic processes shape their evolution during DNA replication including accumulation of point mutations, loss or acquisition of genes, or larger genomic segments and genome rearrangements. Whole‐genome sequencing using high throughput sequencing methods allowed the detection and analysis of large numbers of single nucleotide polymorphisms (SNPs), revealed to be evolutionary stable. This allowed representative non‐homoplastic SNPs, called canonical SNPs (canSNPs) to be selected for the definition of branch points, which accurately define main clades and lineages of B. anthracis (Pearson et al. 2004; Van Ert et al. 2007; Figure 27.1). Thus, genome sequencing of a worldwide collection of more than a thousand strains enabled allocation of the strains into three clades: A, B, and C, using a set of 13 canSNP markers (Van Ert et al. 2007). Subsequently the clades have been subdivided into lineages (Figure 27.1). Clade A, the worldwide most spread clade, is subdivided in nine lineages: A.Br.005/006 (containing the former basal A‐strains mostly from Africa), A.Br.Vollum (Middle East, Caucasus, Europe and Northern America, Australia, China), A.Br.H9401, A.Br.004.054 (Americas Africa, Europe), A.Br.Ames (Northern America), A.Br.Sterne, A.Br.Aust94, A.Br.008/011, A.Br.TEA.011. This latter lineage is further subdivided in A.Br.WNA (United States, Canada), A.Br.TEA.011a (Senegal, Gambia) and A.Br.TEA.011b (Northern France) (Saile and Koehler 2006; Van Ert et al. 2007; Derzelle et al. 2011, 2015a, 2016a; Figure 27.1). Clade B, a geographically more limited clade, representing about 15% of the strains sequenced, is subdivided in B.Br.001.002 Kruger (South Africa) and B.Br.CNEVA, which contains exclusively European strains from France, Southern Germany, Switzerland, Northern Italy, Croatia, Slovenia, Slovakia, and Poland (Derzelle et al. 2011, 2015b, 2016b; Girault et al. 2014a). Clade C occurs very infrequently and currently only a few strains have been analyzed thereof (Saile and Koehler 2006; Figure 27.1). The analysis of the population structure allowed studying the dissemination and phylogeography of B. anthracis over many centuries. However, the significance of clustering genomic data are strongly dependent on good metadata of the strains to avoid wrong conclusions (Pilo et al. 2008; Pilo and Frey 2018). Clade A is widely dispersed throughout the world, most likely through the rapid way via trade of animal products, in particular unprocessed goat and sheep hair for wool production. Strains of lineage A.Br.Vollum are worldwide the most dispersed. They are dominant in the central Caucasus but are found also in Western China as well as in Northern America, Australia, and Europe, where they were frequently (and still are occasionally) isolated in wool factories that are processing raw wool from the Cashmere area, Pakistan, Afghanistan, or Kazakhstan (Pilo et al. 2008; Aikembayev et al. 2010; Khmaladze et al. 2014; Derzelle et al. 2015a, 2015b; Figure 27.1). These strains jumped in the past to the cattle population, causing concurrently overlapping epidemiological situations in certain European countries with strains from two different roots (Derzelle et al. 2011). A particular lineage of B. anthracis clade A, which lacks the expression of the spore surface‐associated anthrose‐containing oligosaccharide that is generally specific to the species, was identified in western Africa (Chad, Cameroon, Mali). This lineage that is estimated to have emerged recently shows a geographic correlation to massive vaccination with the live vaccine strain Sterne, which produces a strong antigenic response to anthrose (Tamborrini et al. 2011). An interesting lineage is A.Br.TEA.011, which is highly branching with only a few SNPs between branches and includes the North American sublineage A.Br.WNA. A large number of strains with solid metadata allowed development of an ungulate transmission model. The estimated infection/death/year rate of 0.28 generations/year is based on a study of 13 consecutive anthrax outbreaks of cattle in Northern Canada between 1962 and 1991 using strains with precise data on time and location of isolation (Kenefic et al. 2008, 2009). From these data, the authors estimate the dispersion of clade A from Eurasia to the Northern American continent in the late Pleistocene (about 13 000 years ago) via the former Bering Land Bridge, progressing from Northern Canada southwards to Southern and South‐Western USA. An alternative route of dispersion was estimated from the common origin of sublineage A.Br.WNA of Northern America with the two sublineages A.Br.TEA.011a (Senegal, Gambia) and A.Br.TEA.011b (Northern France) that split about 500 years ago when Europeans started to settle in Northern America and Africa (Figure 27.1; Vergnaud et al. 2016). This would hypothesize the spread of A.Br.WNA to Northern America via Breton explorers and emigrants from Northern France, such as Jacques Cartier in 1541 and Samuel de Champlain in 1608, as the emigrants often brought their animals, which might have transmitted B. anthracis. However, the lack of documented established anthrax in Northeastern Canada (Québec), the entering point of the settlers, and the fact that the most basal A.Br.WNA subclade originates from Northwestern Canada requires further verification of this hypothesis. Clade B seems to have diverged from clade A about the time of the emergence of agriculture and farming in the fertile areas in Europe. Specimens of clade B are mostly related to bovine anthrax in Europe. The various sublineages of B.Br.CNEVA can be strongly attributed to the different cattle breeds that traditionally were breed very stably and geographically isolated from each other for hundreds of years in the various regions of France and Switzerland (Girault et al. 2014a, 2014b; Derzelle et al. 2016b). The oldest currently known specimen of anthrax is a blood sample on a glass slide with originates from a diseased bovine in 1878 in Germany and was recently sequenced showing its belonging to clade B (Braun et al. 2020). The exceptional lineage B.Br.001/002/Kruger (Figure 27.1) contains strains from South Africa indicating that European settlers might have transmitted this lineage of cluster B to Southern Africa. Infection of animals and humans occurs by spores that are able to enter via skin in particular skin lesions, or by the intestinal route or by inhalation. Exceptionally B. anthracis infections occur by abusive injection of contaminated drugs. Figure 27.2 Overview of the molecular mechanisms of virulence of Bacillus anthracis. B. anthracis with the two virulence plasmids pXO1 and pXO2 and the chromosome is illustrated in the upper part. Variable number tandem repeats (VNTRs) on the chromosome are indicated by black blocks. They played a central role in the molecular phylogenetic analysis of B. anthracis strains prior to whole genome sequencing. Susceptible host cells are represented the lower part. Favorable conditions as found upon infecting a mammalian host (e.g. temperature around 37°C) trigger the master virulence regulator AtxA located on plasmid pXO1 of B. anthracis (upper left). This induces the synthesis of the four major virulence factors: the lethal toxin zinc metalloprotease (LEF), the edema factor calmodulin‐dependent adenylate cyclase (EF) and the adhesive subunit of the two toxins named “protective antigen” (PAG) all of which are encoded on plasmid pXO1. Concomitantly the poly‐γ‐D‐glutamic acid polymer capsule (CAP) (green) specified by plasmid pXO2 is induced by the acpAB gene products. The capsule allows survival of the vegetative bacterium in macrophages. PAG (blue) binds to the receptors CMG2 and TEM8 on the host cell and is subsequently cleaved by furin, allowing multimerization and binding of LEF (yellow) or EF (red). The PAG:EF and PAG:LEF complexes are then internalized by endocytosis in a dynamine‐clathrin dependent way. In the acid environment of the endosomes, the PAG:EF and PAG:LEF complexes change conformation in order to dissolve the complexes, transit to late endosomes and release the toxic subunits, which exert their activities. The potent adenyl cyclase EF leads overproduction of cAMP causing a decrease of Cl‐ ions and loss of water resulting in oedema. LEF induces apoptosis and subsequent shock by release of pro‐inflammatory cytokines by proteolytic cleavage of MAPKK. Part of the toxins can endure in intraluminal vesicles that are able to transfer as exosomes and to be incorporated to non‐infected cells, where the toxins can be stored for long term, be released and exert their activity long after the infection has taken place. CAP, capsule; PAG, protective antigen; EF, oedema factor; LEF, lethal factor; VNTR, variable number tandem repeat; MAPKK mitogen‐activated protein kinase kinase; CaM, calmodulin; cAMP, cyclic adenosine 3′ 5′ monophosphate; CMG2, capillary morphogenesis gene 2. Source: Adapted from Pilo and Frey (2018). In cutaneous anthrax, low‐level germination leads to growth at the site of infection, which is suspected to be facilitated by cutaneous microlesions. Growth and action of toxins leads to localized edema and necrotic lesions of the skin resulting in a typical coal‐black appearance of the affected tissue, which gave the name of the disease “anthrax” (Greek άνθρακος, coal, carbon). Cutaneous anthrax in humans was often reported in the past in workers at wool‐processing factories where it represented to most frequent form of anthrax and was called “wool sorters disease.” It generally remains localized and can be treated with penicillin or quinolone antibiotics. It rarely results in septicemia and generalized toxemia by spreading to further parts of the body. Cutaneous anthrax is only rarely described in animals, mostly in pigs that occasionally show signs of cutaneous anthrax. Pigs seem to be much less affected by anthrax than ruminants, which may be due to both genetic and physiologic differences as well as by their different habitats and a generally more humid environment (Mock and Mignot 2003). Intestinal anthrax starts with low‐level germination at the site of infection, subsequently leading to massive effusion, mucosal edema and necrotic lesions and subsequent local multiplication within macrophages. Regional hemorrhagic lymphadenitis may lead to septicemia and toxemia, and finally to shock and death. In certain cases, lymphatic or hematogenous spread to the brain results in meningitis and rapid death. Gastrointestinal anthrax is considered to be largely underreported in both humans and animals. However, it seems to be dispersed in humans in rural disease‐endemic areas of the world, mostly caused by ingestion of meat from animals affected by anthrax. The spectrum of responses ranges from subclinical infection to death. Certain studies report cases of gastrointestinal anthrax to outnumber those of cutaneous anthrax. However, differential diagnosis of gastrointestinal anthrax is difficult. In particular, the oropharyngeal variant causing fever and toxemia, inflammatory lesions in the oral cavity or oropharynx, enlargement of cervical lymph nodes together with edema of the soft tissue of the cervical area seems to be unknown to most physicians in spite of a relatively high case‐fatality rate (Sirisanthana and Brown 2002). Pulmonary anthrax, the most severe form, is caused by a rapid spread of germinated B. anthracis to the regional lymph node followed by hemorrhagic lymphadenitis, septicemia, toxemia, and shock. In addition, it is suspected that hemorrhagic lymphadenitis leads to pulmonary lymphatic blockage and oedema as cause of death (Mock and Fouet 2001; Guidi‐Rontani and Mock 2002; Mock and Mignot 2003). Pulmonary anthrax is assumed the most frequent cause of anthrax in ruminants due to inhalation of spores by animals grazing on dry prairies with short grass. In humans, pulmonary anthrax is the most dreaded form of anthrax as it develops very rapidly leaving hardly time for efficient therapy. Virulence of B. anthracis is mainly determined by the two main anthrax toxins, the lethal factor LEF and the edema factor EF encoded on the virulence plasmid pXO1 of 182 kbp and a polycarbonate capsule CAP specified by the capsular biosynthesis genes capABCDE on plasmid pXO2 of 95 kbp (Figure 27.2). Both plasmids pXO1 and pXO2 also contain the regulatory genes for the expression of the toxin and capsule biosynthesis components. The two main anthrax toxins LEF of 90 kDa and EF of 89 kDa are binary protein toxins containing a common cell‐adhesive component called protective antigen PAG of 83 kDa that is binding to and transporting the two toxic enzymes LEF and EF to the cytosol of the host cell (Okinaka et al. 1999; Fouet and Mock 2006; Figure 27.2). The lethal factor LEF is a zinc dependent metalloprotease encoded by gene lef on pXO1 (Figure 27.2). It inactivates members of the mitogen‐activated protein kinase kinase family (MEK) in the host cell cytoplasm by proteolytic cleavage leading to excessive release of cytokines, induction of apoptosis, and finally to necrosis and hypoxia (Duesbery et al. 1998; Abrami et al. 2005; Depeille et al. 2007). The edema factor EF is a highly efficient calcium and calmodulin‐dependent adenylyl cyclase encoded by gene cya on pXO1 (Figure 27.2). It reaches the same compartments as LEF using the adhesin subunit PAG. This adenylyl cyclase is about 1000 times more active to convert ATP to cyclic adenosine monophosphate (cAMP) than the mammalian adenylyl cyclase. At high concentrations, c‐AMP results in the decrease of chloride ions and a strongly increased water efflux from the affected cells (Figure 27.2). This leads to massive edema that is typically seen in cutaneous anthrax. EF was also shown to inactivate phagocytes thus protecting infecting B. anthracis by allowing escape from the innate immune response (Drum et al. 2000). The capsule is based on an anionic polypeptide consisting of linear chains of PGA that is covalently attached to the peptidoglycan of the bacterial envelope. It represents the second major virulence factor of B. anthracis. The capsule is synthetized by the capsule biosynthesis genes capABCDE on plasmid pXO2. CapB is member of the tetrahydrofolate synthase family and acts as the glutamic acid polymerase together with CapE to form the polymer, while CapD is a γ‐glutamyl‐transpeptidase anchoring the PGA to the peptidoglycan layer. The capA and capD
27
Bacillus anthracis
Introduction
Characteristics of the Organism: Taxonomy and Phylogeny
Pathogenic B. cereus Biovar anthracis
Source and Spread of the Infection: Ecology, Evolution, and Epidemiology
Types of Disease and Pathologic Changes
Virulence Factors and Pathogenomics
Stay updated, free articles. Join our Telegram channel

Full access? Get Clinical Tree
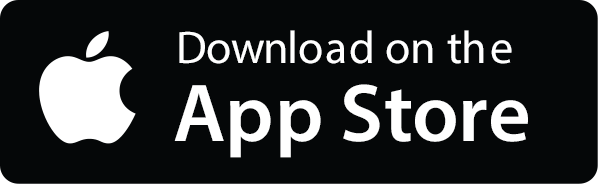
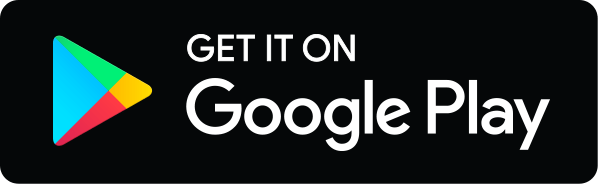