Chapter 56 Hypomagnesemia refers to low circulating concentrations of magnesium in the serum and occurs when total magnesium (Mg) concentrations are below 1.89 mg/dl in dogs and below 1.75 mg/dl in cats; ionized hypomagnesemia occurs when serum ionized Mg concentrations are below 0.43 mmol/L in both species. These values may vary slightly depending on the reference laboratory used. In veterinary medicine, hypomagnesemia is one of the most common electrolyte abnormalities detected in critically ill patients. In 48 dogs admitted to a veterinary teaching hospital intensive care unit, the incidence of hypomagnesemia at admission was 54%, and hypomagnesemic dogs had a higher incidence of concurrent hypokalemia and hyponatremia, as well as a longer length of hospitalization, compared with normomagnesemic counterparts (Martin et al, 1994). A prospective study of 57 cats admitted to an intensive care unit reported that hypomagnesemia developed in 28% during hospitalization (Toll et al, 2002); another study reported an incidence of hypomagnesemia of 62% and 57% in cats with diabetes mellitus and diabetic ketoacidosis, respectively (Norris, Nelson, and Christopher, 1999). Its high frequency of occurrence and impact on morbidity in veterinary patients have established hypomagnesemia as one of the most clinically significant electrolyte disorders in critically ill animals. The three causes of Mg deficiency are (1) decreased intake, (2) alterations in compartmental and cellular distribution, and (3) increased loss (Box 56-1). Realistically, hypomagnesemia results not from any one of these mechanisms but rather from a combination of all three. Poor dietary Mg intake does not usually cause clinically significant hypomagnesemia; however, chronic dietary Mg deficiency may lead to a significant depletion of body stores. Administration of Mg-deficient intravenous fluids or parenteral nutrition may also result in hypomagnesemia in hospitalized animals.
Approach to Hypomagnesemia and Hypokalemia
Hypomagnesemia
Causes
< div class='tao-gold-member'>
Stay updated, free articles. Join our Telegram channel

Full access? Get Clinical Tree
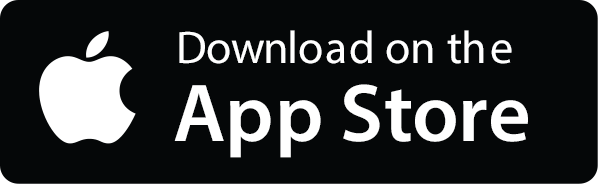
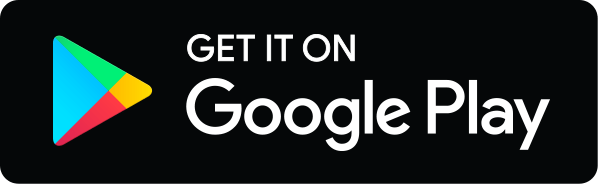