Chapter 12 Application of Laser Energy with Endoscopic Visualization
Laser–Tissue Interaction
Most laser wavelengths in the visible and infrared light spectrum can be transmitted with endoscopic devices (Figure 12-1). Diode lasers producing wavelengths in the 810- to 980-nm range and neodymium:yttrium aluminum garnet (Nd:YAG) lasers producing wavelengths in the 1064-nm range are primarily used with fiberoptic, rigid endoscopic, and laparoscopically directed laser energy transmission to target tissues.
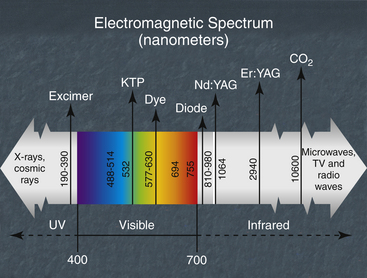
Figure 12-1 Diode laser wavelengths in the electromagnetic spectrum.
(Modified from an illustration provided by Lumenis, Santa Clara, Calif.)
Laser energy is electromagnetic radiation derived from the production of photons via stimulation of specific materials that are capable of high-intensity photon emission. Visible light photons are limited to the part of the electromagnetic spectrum between 400 nm and 700 nm. Infrared light photons (not visible to the human eye, a key safety factor) represent the electromagnetic regions from 700 nm to 12,000 nm. Laser energy specifically transmits as an intense, coherent, monochromatic, collimated, photon beam.
Specifically germane to this discussion is a primary understanding of the specific wavelength absorption coefficient of diode, Nd:YAG, and CO2 laser wavelengths related to water, melanin, hemoglobin, and oxyhemoglobin. A full understanding of these absorption interactions is critical for producing the desired tissue effect with the selected laser wavelength. In most cases clinicians are attempting vaporization, ablation, dissection, resection, or hemostasis at the particular tissue location. Figure 12-2 gives a basic overview of varying absorption events with respect to the laser wavelengths discussed.
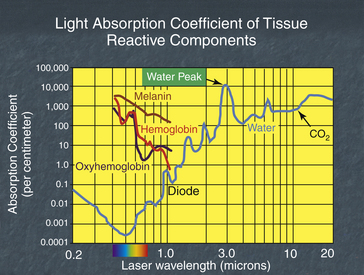
Figure 12-2 Laser light absorption coefficient for diode and CO2 wavelengths.
(Modified from an illustration provided by Lumenis, Santa Clara, Calif.)
Visible and diode laser energy is most readily transmitted through water because its coefficient to water is exceedingly low. This is very advantageous when laser delivery devices are passed through operating channels of scopes such as cystoscopes, which are used during fluid infusion in aqueous or semiaqueous areas. These laser wavelengths work well in environments such as the abdominal cavity, urogenital region, and intraarticular and intrathecal regions of the body. Diode wavelength laser energy is carried via a quartz crystal fiber. This fiber is very flexible and easy to manipulate in the working channel of an endoscopic device (Figure 12-3). CO2 laser wavelength energy has an exceedingly high water coefficient. This renders the CO2 laser much less advantageous in aqueous areas of the patient’s body. The fluid environment would have to be suctioned for lasing to be accomplished. CO2 laser wavelength energy can be considered for endoscopic otoscopy, rhinoscopy, and some abdominal procedures in which limited fluid content is observed. CO2 laser wavelength energy is advantageous because a very limited peripheral tissue effect, usually less than 0.1 mm, occurs. Thus, in areas in which specific tissue is to be vaporized without any peripheral tissue damage, the CO2 laser wavelength energy is preferred.
Diode Wavelength Energy
Used in scope applications, diode laser wavelength energy can be applied to the target tissue in one of two modes. These modes are described as noncontact or contact. Diode laser wavelength energy being applied in a noncontact mode (true laser photon transmission into the target tissue) provides a tissue interaction effect that optimizes the absorption of the photons within hemoglobin, oxyhemoglobin, melanin, and, to a variable degree, water. In noncontact mode (also known as free-beam mode) tissues that are not highly vascular or pigmented (e.g., fascia, tendons, ligaments, adipose, and cartilage) will allow transmission of the diode photons into deeper, more vascular tissue. This can produce unexpected and unwanted distant tissue site effects. Therefore it is critical that the surgeon match the diode wavelength with the tissue to be treated. The surgeon also needs to be aware of what structures or organs are lying deep to the targeted area.
Diode lasers in fiberoptic, endoscopic, and laparoscopic procedures are most commonly applied in the contact mode of energy transmission to the tissue through a rigid endoscopic viewing device (Figure 12-4). Contact mode provides a high heat event at the fiber tip–tissue interface. The surgeon accomplishes this by exposing the diode fiber tip to a piece of wood and firing the laser. This creates a carbonized layer (char) at the cleaved fiber tip surface. This char causes the diode photons traveling down the quartz crystal fiber to impact the carbon molecules and release their energy into the carbon in the form of heat. The more photons that strike the carbonized surface per unit time (watts), the greater the heat effect at the tip. This creates a direct tissue contact ablation and cauterization effect. It allows for maximal tissue resection and cauterization of the target tissue vasculature with minimal or no transfer of diode wavelength photons to distant tissue sites.
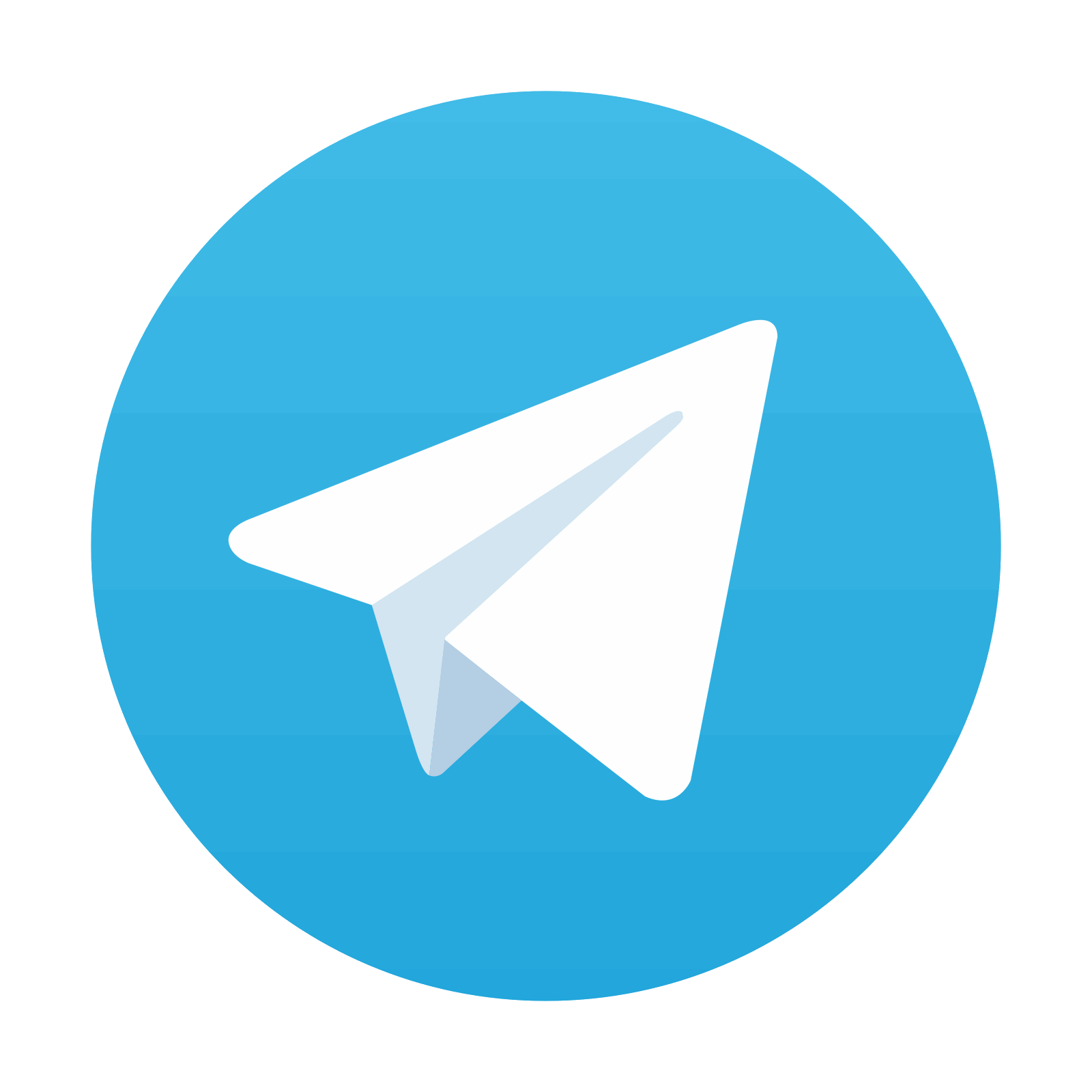
Stay updated, free articles. Join our Telegram channel

Full access? Get Clinical Tree
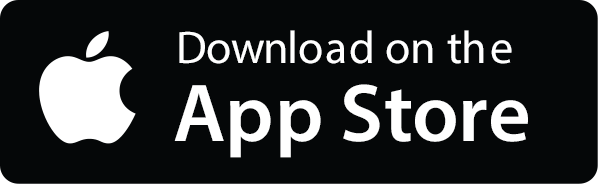
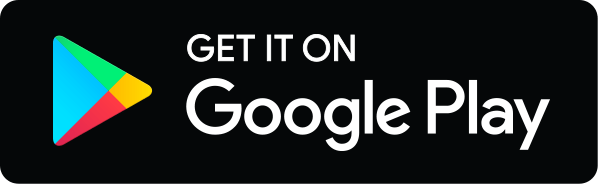