Chapter 3 Antimicrobial Agents, Mechanisms of Resistance, and Susceptibility Testing
The veterinarian attending to a domestic animal with a bacterial infection must identify and eliminate predisposing factors (e.g., foreign bodies or stress), reduce the numbers of the etiological agent and its toxic products from the site of infection when possible (usually by drainage or some other form of surgical intervention), and reduce proliferation of the agent to allow host defenses to gain control at the infected site (most often by use of antimicrobial chemo-therapeutic agents). Administration must be in a manner that exploits a drug’s pharmacodynamic parameters relative to the target pathogen; successful outcomes are most common when an effective drug concentration is maintained for an appropriate time at the site of infection, allowing specific and nonspecific defenses to eliminate the offending pathogen. Knowledge of natural resistance traits of common pathogens is a key component of antimicrobial susceptibility testing and the practice of veterinary therapeutics.
ANTIMICROBIAL AGENTS
Antimicrobial agents may be based on fungal or higher bacterial metabolites or may be wholly synthetic. They may have a broad or narrow spectrum of activity, as in the case of cephalexin (afirst-generation cephalosporin, which has a narrow spectrum of activity, directed primarily against gram-positive bacteria) and tetracyclines (which are active against a broad range of bacteria, including some mycoplasmas, rickettsiae, and chlamydiae) (Tables 3-1 and 3-2). Antimicrobials may also be classified by their ability to kill the target organism or simply inhibit growth. Bactericidal agents kill at or near the same concentration that inhibits bacterial growth (the minimal inhibitory concentration, MIC). Bacteriostatic drugs inhibit multiplication at the MIC, but a higher concentration, the minimal bactericidal concentration (MBC), is necessary to kill bacteria.
TABLE 3-2 Mechanism of Action of Various Antimicrobial Agents
Agent | Mechanism of Action |
---|---|
Aminoglycosides/aminocyclitols | Inhibit protein synthesis via irreversible binding to 30S ribosomal subunit |
β-lactams | Inhibit enzymes essential for peptidoglycan synthesis |
Chloramphenicol/florfenicol | Inhibits protein synthesis via irreversible binding to 50S ribosomal subunit |
Fluoroquinolones | Inhibit DNA gyrase (involved in synthesis and maintenance of DNA) |
Lincosamides | Inhibit protein synthesis via binding to 50S ribosomal subunit |
Macrolides | Inhibit protein synthesis via reversible binding to 23S rRNA of 50S ribosomal subunit |
Pleuromutilins | Inhibit protein synthesis by effects on peptidyl transferase center of 50S ribosomal subunit |
Potentiated sulfonamides | Inhibit folate metabolism, interfering with DNA synthesis |
Sulfonamides | Inhibit folic acid synthesis via competition with para-aminobenzoic acid for dihydropteroate synthetase |
Tetracyclines | Inhibit protein synthesis via reversible binding to 30S ribosomal subunit |
DNA, Deoxyribonucleic acid; rRNA, ribosomal ribonucleic acid.
Classes of Antimicrobials
Aminoglycosides include amikacin, gentamicin, kanamycin, neomycin, streptomycin, tobramycin, and apramycin and are derived from either Streptomyces spp. or Micromonospora spp. These agents inhibit protein synthesis by binding tothe 30S subunit of the ribosome, rendering it unavailable for translocation of mRNA duringprotein synthesis, and can cause mistranslationof genes and production of defective proteins. Their activity is directed mainly against aerobic, gram-negative bacilli, Staphylococcus aureus, and mycoplasmas. Aminoglycosides may be both nephrotoxic and ototoxic, but these toxic effects are mitigated with appropriate dosing regimens.
Chloramphenicol, a bacteriostatic agent originally isolated from Streptomyces venezuela, is now produced as a derivative of dichloroacetic acid. The drug binds irreversibly to the 50S ribosomal subunit and prevents peptide chain elongation. It has a broad spectrum of activity against gram-positive and gram-negative aerobes, anaerobes, chlamydiae, rickettsiae, and many mycoplasmas. Use of this drug in food animals is prohibited in the United States. Eukaryotic mitochondrial ribosomes are also subject to the effects of chloramphenicol, and bone marrow suppression with irreversible aplastic anemia and neutro-penia may be a consequence of chloramphenicol therapy.
Lincosamides have a moderate spectrum of activity. Clindamycin, a semisynthetic derivativeof lincomycin, is used primarily in small animal medicine for treatment of anaerobic or staphlylococcal soft tissue infections. Lincomycin is used to control dysentery, erysipelas, and mycoplasmosis in swine. Pirlimycin is veterinary specific and has activity against gram-positive organisms, especially staphylococci and streptococci. It is used as an intramammary infusion for treatment of mastitis due to infection with S. aureus, Streptococcus agalactiae, Streptococcus uberis, and Streptococcus dysgalactiae ssp. dysgalactiae infection in lactating dairy cattle. Lincosamides are toxic for sheep, horses, rabbits, and laboratory rodents, and may induce pseudomembranous colitis as a result of overgrowth of Clostridium difficile in laboratory animals.
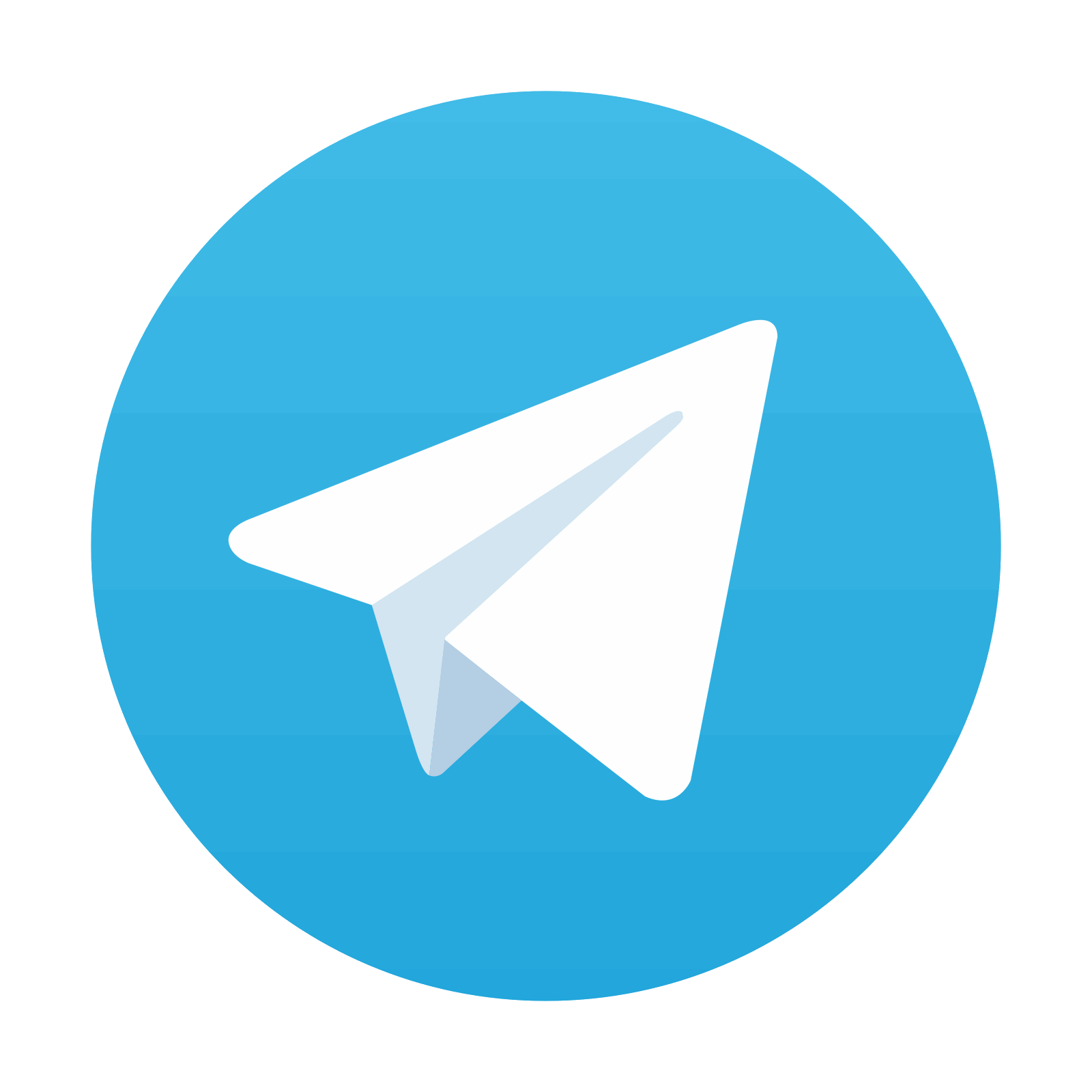
Stay updated, free articles. Join our Telegram channel

Full access? Get Clinical Tree
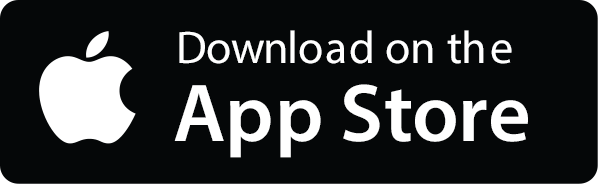
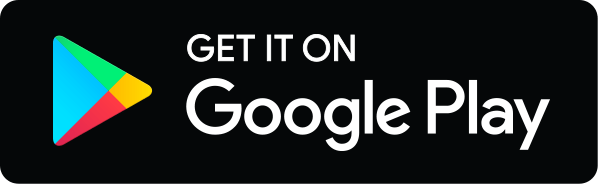