40 Carlos E. Lanusse, Luis I. Alvarez, and Guillermo L. Virkel Anticestodal drugs used to treat tapeworm infections may act as either taeniafuges or taeniacides. The former compounds facilitate tapeworm expulsion from the host, whilst the later cause the death of the tapeworm in situ. Because the cestode’s scolex is capable of regenerating an entire organism, drugs that merely remove the proglottids or the whole tapeworm body but leave the scolex intact are unsatisfactory. Insufficient drug diffusion into the scolex and consecutive two or three proglottids, due to the inflammatory reaction of the intestinal mucosa, may account for such a limited cestocidal activity. The usual interval from destrobilization to patency is approximately 3 weeks, so the recommended interval for evaluating tapeworm therapy is ∼3 weeks after initial drug treatment. Tapeworm infection in farm animals may be a minor problem and usually does not require treatment with a specific cestocidal drug. Some of the broad-spectrum benzimidazole compounds used to control nematode infections (see Chapter 39) are also effective against tapeworms. However, special attention is given to the treatment of Anoplocephala perfoliata infections, the commonest tapeworm parasite in horses. Increasing evidence is available suggesting an association between this tapeworm and erosions around the ileocecal valve, intussusceptions, ileal impactions, and other conditions leading to recurrent episodes of intestinal colic (Gasser et al., 2005). It has been shown that oral administration of a pyrantel pamoate paste (see Chapter 39) at 13.2 mg/kg resulted in effective (96%) control of this tapeworm in naturally infected horses (Reinemeyer et al., 2006). Treatment of tapeworm infections is also necessary in companion animals. It is medically significant that dogs and cats are definitive hosts of tapeworms whose larval stages cause zoonosis. For example, effective treatment of Echinococcus granulosus in dogs is extremely important since the larval stage of this tapeworm may cause hydatidosis in the intermediate hosts, primarily sheep and humans. Environmental control of intermediate hosts is also essential to prevent reinfection after treatment. The control of fleas and lice that vector Dipylidium caninum, and restricted access of carnivores to mammalian intermediate hosts to preclude Taenia infections, are essential (Reinemeyer and Courtney, 2001). Taeniafuge compounds interfere with the ability of tapeworms to maintain their site of location in the gut. They paralyze tapeworms at least temporarily, but if tapeworms recover prior to expulsion, reattachment to the gut may occur. Thus, treatment with taeniafuges has been routinely accompanied by purgation. Arecoline, a muscarinic agonist, induces parasite’s spastic paralysis and enhances host gut motility, which facilitates tapeworm expulsion. The search for enhanced efficacy motivated the development of synthetic taeniacidal drugs that are now widely used. Many of the older antitapeworm drugs are natural organic compounds (taeniafuges). Readers interested on specific information on those older antitapeworms are referred to a previous edition of this textbook (Roberson and Courtney, 1995). Bunamidine (N,N-dibutyl-4-(hexyloxy)-1-naphthami-dine) (Figure 40.1) is a taeniacidal drug that disrupts the tapeworm’s tegument and reduces glucose uptake. Consequently, subtegumental tissues are exposed and destroyed by the host digestive enzymes. It is formulated as tablets (hydrochloride salt) or suspensions (hydroxynaftoate salt) for oral administration in companion animals and ruminants, respectively. The hydrochloride salt is widely used for treatment of cestode infections in dogs and cats. Bunamidine hydrochloride exhibits >90% efficacy against Taenia spp., Dipylidium spp., Mesocestoides spp., and Diphyllobothrium spp. (oral treatment at 25–50 mg/kg). However, efficacy against D. caninum may be erratic. Effectiveness ranging from 85.9 to 98.8% (immature stages) and 100% (mature stages) of E. granulosus have been reported (Andersen et al., 1975). Bunamidine prepared as a hydroxynaftoate salt is effective against Moniezia spp. infections in small ruminants. Figure 40.1 Bunamidine chemical structure. Dissolution of tablets in fasted animals is improved and, therefore, subsequent contact of the drug with the parasite in the gut is enhanced, resulting in advantageous efficacy. The drug is safe because is not extensively absorbed in the duodenum and low amounts of the absorbed compound are inactivated in the liver. Administration of dissolved tablets enhances drug absorption and, consequently, the risk of systemic adverse effects such as liver damage and ventricular fibrillation. Deaths as consequence of cardiac failure have been sporadically reported within 24 hours posttreatment. Emesis and diarrhea are the most frequent adverse effects following bunamidine administration. More efficacious cestodicidal drugs have displaced bunamidine, which is no longer available in the US market. This salycilanilide compound(5-chloro-N-(2-chloro-4-nitrophenyl)-2-hydroxybenzamide (Figure 40.2) was widely used for treatment of cestode infections in dogs and cats from the 1960s to the 1980s. Niclosamide is highly effective against most of the tapeworm species in companion animals. However, it has poor efficacy against Dipylidium spp. and E. granulosus. This drug is also effective for the treatment of common tapeworms in ruminants such as Moniezia spp. and Thysanosoma spp. In horses it may be used for the treatment of Anoplocephala spp. Niclosamide’s taenicidal activity involves interference with glucose absorption, inhibition of oxidative phosphorylation, and stimulation of adenosine triphosphatase activity at mitochondrial level. All these mechanisms lead to the death of the parasite and its subsequent digestion within the gut (Al-Hadiya, 2005). It may be administered as either suspension by oral or intraruminal routes in ruminants or as tablets for oral treatment in companion animals. Niclosamide administration in fasted animals increases its efficacy. Its oral administration, at doses of 100–157 mg/kg, produced only low plasma concentrations, which correlates with low gastrointestinal (GI) absorption and the well-known safety of the drug. The absorbed drug is rapidly inactivated in the liver into aminoniclosamide. Similarly to bunamidine, niclosamide has been replaced in small animal practice by modern cestocides. Interested readers are referred to a previous edition of this text (Roberson and Courtney, 1995) for a thorough discussion of the therapeutic properties of niclosamide in animal species of veterinary interest. Interestingly, niclosamide has been identified as a potential anticancer agent due to its inhibitory effects on multiple intracellular signaling pathways. The signaling molecules in those pathways may be over-expressed, constitutively active, or mutated in different cancer cells (Li et al., 2014). Consequently, a number of in vitro and in vivo studies have reported on the anticancer effectiveness of niclosamide, used either alone or in combination with other antineoplastic agents. Figure 40.2 Chemical structure of the salicylanilide drug niclosamide. Praziquantel is a synthetic isoquinolinepyrazine derivative: 2-(cyclohexykcarbonyl)-1,2,3,6,7,l1b-hexahydro-4H-pyrazino[2,1-a] isoquinolin-4-one (Figure 40.3). Praziquantel is highly efficacious against a variety of cestode and trematode parasites and it is widely used in both veterinary and human medicine. It has extremely high activity against adult stages of all species of tapeworms in farm and companion animals (Thomas and Gonnert, 1978). It also has good activity against larval forms of cestodes. Administration of praziquantel at 5 mg/kg in cats and dogs is completely effective against all stages of Taenia hydatigena, T. pisiformis, T. ovis, T. taeniaeformis, D. caninum, Mesocestoides corti, E. multilocularis, and E. granulosus (Thomas and Gonnert, 1978). This dose is generally recommended for elimination of the common cestode species of dogs and cats, except Spirometra mansonoides and Diphyllobothrium erinacea, which require 25 mg/kg on each of 2 consecutive days. A 10 mg/kg dosage is required to achieve good efficacy against the juvenile forms of these parasites. The drug ensures 100% elimination of E. granulosus in dogs and is the unique drug recommended for the treatment of this tapeworm. Oral administration of a praziquantel-containing paste (at 50 mg/kg) was effective for the control of Dicrocoelium dendriticum in llamas (Dadak et al., 2013). A combined formulation of febantel and praziquantel is available on the veterinary market for use in the treatment of GI nematodes and cestodes. This formulation must be administered on 3 consecutive days, at doses of 10–15 mg/kg (febantel) and 1–1.5 mg/kg (praziquantel) for adult and young dogs. A triple combination of praziquantel, pyrantel, and oxantel is also marketed as broad-spectrum dewormer for dogs and cats. Other commercial combinations used in companion animals include praziquantel plus oxibendazole, mebendazole, or fenbendazole. Combinations of praziquantel with either ivermectin (paste) or moxidectin (gel formulation) are commonly available as broad-spectrum antiparasitic formulations for use in the treatment of GI nematode and cestode infections in horses (Holm-Martin et al., 2005; Rehbein et al., 2007). A combined spot-on preparation of praziquantel and the novel compound emodepside (see Chapter 39) has been introduced into the market as a broad-spectrum anthelmintic for cats. This anthelmintic formulation has been proven to be effective for the treatment of the feline lungworm Aelurostrongylus abstrusus (Traversa et al., 2009). Figure 40.3 Chemical structures of praziquantel and its active metabolite (4′hydroxy-praziquantel). The asterisk (*) indicates the asymmetric (chiral) center in both molecules. Praziquantel is also highly effective against cestodes of ruminants. All species of Moniezia, Stilesia, and Avitellina of sheep and/or goats are eliminated by a single dose of 10–15 mg/kg. Praziquantel has been approved for tapeworm treatment in horses in different countries. It has been successfully used for the treatment of the cestode A. perfoliata in this species (Lyons et al., 1998). Combined formulations (gel, paste) containing ivermectin plus praziquantel are marketed for use in the treatment of GI nematode and cestode infections in horses. Most studies concerning praziquantel’s mode of action have been done using the human trematode Schistosoma mansoni as a model parasite (Day et al., 1992). Praziquantel induces a rapid and sustained paralytic muscle contraction of the parasite and tegumental disruption. The contraction of parasite musculature is the primary effect, which is followed by a rapid vacuolization of the syncytial tegument. Muscular contraction and tegumental disruption are followed by exposure of parasite antigens, binding and penetration of host immune cells into the parasite (Martin, 1997). Metabolic changes include decrease in glucose uptake, glycogen storage, ATP content, and lactate release. All these effects are attributed either directly or indirectly to an alteration of intracellular Ca2+ homeostasis. Praziquantel effects are thought to be mediated by the release of intracellular stored Ca2+, in addition to the increase of Ca2+ influx across the schistosome tegument (Day et al., 1992). Exposure and release of parasite antigens, as well as metabolic changes are attributable to the primary effect on the tegument. The exact molecular target, as well as its location within parasite tissues, remains to be completely understood. Several investigations have been focused on the beta subunit of voltage-gated Ca2+ channels as proposed targets for praziquantel action (Greenberg, 2005; Jeziorski and Greenberg, 2006). These channels are critical sites of extracellular Ca2+ influx and also play an important role in regulation of intracellular Ca2+ homeostasis. A variant of the beta subunit of voltage-gated Ca2+channel was also found in platyhelminthes such as Taenia solium (Jeziorski and Greenberg, 2006). In addition, it has been demonstrated that praziquantel is an inhibitor of adenosine uptake and a relationship between this effect and praziquantel-mediated effects on Ca2+ influx has been hypothesized (Angelucci et al., 2007). Considering that platyhelminthes lack the biochemical pathway for purine biosynthesis, this mechanism may be involved on praziquantel antiparasitic activity. The chiral nature of praziquantel is other feature related to its pharmacological effects on the parasite. The drug has an asymmetric center in position 11b (see Figure 40.3) and, consequently, two enantiomeric forms: R(−) and S(+) praziquantel. Only the R(−) enantiomeric form has antiparasitic activity as shown by in vitro and in vivo experiments (Staudt et al., 1992; Cioli and Mattoccia, 2003; Angelucci et al., 2007). Praziquantel can be formulated as tablet, paste, or suspension for oral administration, and as solution for SC or IM injections. Commercial formulations are racemates composed of equal parts of both praziquantel enantiomers. The pharmacokinetic behavior and metabolic fate of praziquantel has been investigated in sheep and dogs (Giorgi et al., 2001, 2003). These authors used a higher oral dose (30 mg/kg) than those recommended in these species in order to generate sufficiently high plasma concentrations for analysis. In sheep, praziquantel peak plasma concentration (Cmax) and area under concentration-time curve (AUC) values administered by the IM route at 15 mg/kg were sixfold higher compared to those values observed following oral treatment at 30 mg/kg (Figure 40.4). The pharmacokinetics of praziquantel after its oral and IM administration was studied in water buffaloes, the major reservoirs for the human trematode Schistosoma mansoni in Asia (Sun and Bu, 2012); compared to the enteral administration, its relative bioavailability after the IM treatment was approximately 3.5-fold higher. It has been shown that praziquantel is completely absorbed in the GI tract in almost all species studied. Thus, its low oral bioavailability in sheep and water buffaloes is not attributed to poor GI absorption. An extensive hepatic first-pass effect accounts for the reduced praziquantel systemic availability. Liver oxidative metabolism of praziquantel in sheep resulted in the production of one hydroxylated metabolite, which might be either 11b-hydroxy- or 1-hydroxy-praziquantel (Giorgi et al., 2001). A cytochrome P450 3A isoenzyme was found to be involved in praziquantel hydroxylation in sheep liver. Conversely, the metabolite formed following praziquantel administration to dogs is 4′-hydroxy-praziquantel (Giorgi et al., 2003). This is consistent with previous studies in other species, such as rats, monkeys, and humans, in which 4′-hydroxy-praziquantel was the main metabolite (Masimirembwa and Hasler, 1994). Praziquantel and its 4′-hydroxylated metabolite were depleted in parallel from plasma in dogs, which is supported by an AUC praziquantel/4′-hydroxy-praziquantel ratio equal to 1.3 (Figure 40.5). Increased levels of the parent drug and its metabolite following administration of liquid or dry grapefruit juice combined with praziquantel to dogs has been observed. It has been shown that grapefruit juice may be an inhibitor of the P-glycoprotein transport protein and cytochrome P450-mediated oxidative drug metabolism. Grapefruit juice may interfere with either the P-glycoprotein-mediated efflux or cytochrome P450-mediated oxidation of praziquantel. Interestingly, 4′hydroxy-praziquantel has been shown to exert roughly similar pharmacological activity compared to its parent compound (Staudt et al., 1992). Metabolism of praziquantel is also stereoselective for the two enantiomers. Thus, two 4′-hydroxy-praziquantel isomers were identified in the plasma of human volunteers (Westhoff and Blaschke, 1992). It has been shown that(−)4′-hydroxy-praziquantel is far more abundant in human plasma than its respective (+)isomer. This finding is highly relevant since, in vitro, there is no significant difference in the pharmacological effect of (−)praziquantel and its respective (−)4′hydroxylated isomer against S. mansoni (Staudt et al., 1992). It has been suggested that efficacy of praziquantel in humans is mainly due to the pharmacological activity of its (−)4′hydroxylated metabolite rather than to (−)praziquantel itself. Renal excretion is the main route of elimination of praziquantel and its metabolites. Figure 40.4 Praziquantel plasma concentration (µg/ml) profiles measured after its oral (30 mg/kg) and intramuscular (IM) (15 mg/kg) administrations to sheep. The inserted table shows mean pharmacokinetic parameters obtained following both treatments. Cmax, peak plasma concentration; Tmax, time to peak plasma concentration; AUC, area under concentration-time curve; T1/2el, elimination half-life. Source: Adapted from Giorgi et al., 2001. Reproduced with permission of John Wiley & Sons. Figure 40.5 Plasma concentration profiles of praziquantel parent drug and its main hydroxylated metabolite (4′hydroxy-praziquantel) following praziquantel administration to dogs (orally, 30 mg/kg). The inserted table shows the comparative kinetic parameters for both molecules. Cmax, peak plasma concentration; Tmax, time to peak plasma concentration; AUC, area under concentration-time curve; T1/2el, elimination half-life. Source: Adapted from Giorgi et al., 2003. Reproduced with permission of Elsevier. Acute and chronic toxicity studies indicate a wide margin of safety for praziquantel. An acute, oral LD5O has not been established in dogs because they vomit when dosages exceed 200 mg/kg. The single therapeutic dose is 3.8–12.5 mg/kg in dogs and 4.2–12.7 mg/kg in cats. Overdoses of up to fivefold are tolerated without adverse effect. Studies in pregnant rats and rabbits detected no embryotoxic or teratogenic effects. Similar tests support the use of praziquantel in breeding and pregnant animals without restrictions. This cestocidal compound (2-(cyclohexylcarbonyl)-oxo-1,2,3,4,6,7,8,12b-octahydropyrazino[2,1-a] [2] benza-zepine) (Figure 40.6), is chemically related to praziquantel. Epsiprantel is used specifically for treatment of the common tapeworms of dogs (D. caninum, T. pisiformis) and cats (D. caninum, T. taeniaeformis). Recommended dosages are virtually 100% effective against those cestode parasites. Epsiprantel causes tegumental damage in larval and adult stages of E. granulosus. At a dosage of 5 mg/kg in dogs, the drug is 94% effective against immature (7-day-old) worms and more than 99% effective against mature stages (Thompson et al., 1991). At doses of 5.5 mg/kg in dogs and 2.5 mg/kg in cats, the drug was >99% effective against E. multilocularis, although residual worms may persist in some animals (Eckert et al., 2001). The recommended dose of 5.5 mg/kg is also 100% effective against Taenia spp. and 99.8% against Dipylidium (Corwin et al., 1989). Figure 40.6 Epsiprantel chemical structure. Similar to praziquantel, epsiprantel affects Ca2+ homeostasis within the parasite. The drug damages the tegument, making it vulnerable to lysis and digestion within the host’s gut. Epsiprantel is only formulated for oral administration in dogs and cats. It is poorly absorbed in the GI tract and is thereby available to act against intestinal cestodes, being eliminated in feces. There are no metabolites and only 0.1% of the administered drug is eliminated by urine. The comparative pharmacological properties of praziquantel and epsiprantel are summarized in Figure 40.7. Figure 40.7 Comparative pharmacodynamic and pharmacokinetic properties of the chemically related anticestodal drugs: praziquantel and epsiprantel. GI, gastrointestinal tract. Due to its low GI absorption, epsiprantel is a safe drug. For example, cats tolerate five times the therapeutic dose of this cestocide once daily for 3 consecutive days. Emesis has been reported in cats administered 40 times the therapeutic dose daily for 4 days. Beagle puppies (7–10 weeks of age) given 100 mg/kg once (18 times the recommended dosage) exhibited no signs of toxicity. Adult dogs treated once with a 36 times overdose were not adversely affected. Emesis is the most commonly observed side effect of epsiprantel and may occur in prolonged treatments. Fasciolosis, caused by the cosmopolitan liver fluke Fasciola hepatica, is the most common and economically important disease caused by trematode parasites in domestic animals worldwide. Fasciolosis is an important zoonotic disease, particularly in underdeveloped countries (Mas-Coma, 2004). Chemotherapy, based on the use of flukicidal compounds, is the main tool to control liver flukes. Most of the antitrematodal drugs discussed herein are compounds used for the treatment of fasciolosis. The tropical counterpart of F. hepatica is F. gigantica and all drugs active against one of the species are equally effective against the other (Boray, 1986). Rumen fluke (Paramphistomum spp.) infections are common in cattle and sheep throughout the world. Adult flukes attach to the rumen wall and are of little consequence to the health of the animal. Large numbers of the immature stages, however, can be seriously pathogenic as they migrate within the gut lumen from the duodenum to the rumen. Intestinal paramphistomosis generally responds well to treatment with drugs that are effective against liver fluke and/or cestode infections in ruminants. Readers interested on specific issues regarding treatment of infections caused by either rumen flukes (Paramphistomum spp.) in sheep and cattle or lung flukes (Paragonimus spp.) in dogs and cats, are referred to the previous edition of this textbook (see Reinemeyer and Courtney, 2001). The liver fluke’s life cycle has several features that should be considered for a better understanding of the pharmacological properties of the antitrematodal drugs. Both immature and mature flukes damage the host liver. After the infective metacercariae are ingested by grazing sheep or cattle, the immature fluke emerges from its cyst, penetrates the wall of the small intestine, traverses the peritoneal cavity, and penetrates the liver capsule within 4 days of infection. During the next several weeks, immature flukes tunnel through liver tissues, feeding and increasing rapidly in size. Acute and subacute fasciolosis are most common in sheep. Both are characterized by the migration of a high number of immature flukes through the liver parenchyma causing severe hepatic hemorrhage, jaundice, and anemia. These stages are often fatal. Clinical signs of acute and subacute fasciolosis are presented within 6–8 weeks postinfection. Antitrematodal drugs used against immature forms must achieve effective concentrations within the liver tissue. The severity of F. hepatica infections in sheep may be enhanced by the fluke’s potential role in disseminating enteric bacteria (Clostridium novyi) in the liver during migration (Reinemeyer and Courtney, 2001). During the eighth week of infection, flukes begin to penetrate the main bile ducts, where they attain sexual maturity by ∼10–12 weeks after infection. Adult flukes are blood-sucking parasites and penetrate within the bile ducts, causing biliary hyperplasia and progressive occlusion. The infected areas may be surrounded by connective tissue, which progressively becomes calcified. Such type of tissue reaction is most common in cattle, were fasciolosis is typically more chronic and subclinical in nature. Although adult flukes are most susceptible (or perhaps most accessible) to fasciolicidal drugs at this stage, the affected areas of the liver tissue become progressively less penetrable by therapeutic agents and consequently more difficult to treat (Reinemeyer and Courtney, 2001). Carbohydrate and energy metabolism in helminth parasites differs from those of the mammalian host. Also, parasites exhibit a wide variation in their carbohydrate break-down pathways during their life cycle (Kita et al., 1997). Parasite metabolism uses an aerobic–anaerobic transition. In general, free-living larval stages obtain their energy from aerobic processes, whereas adult forms have mainly an anaerobic metabolism. A transition in the pathways of energy metabolism was observed in both the cytosol and mitochondria of the liver fluke F. hepatica (Tielens et al., 1987; Tielens, 1994). Overall, energy metabolism in the adult stages is almost completely anaerobic, and aerobic processes remain only in the tegument of the parasite, which is also one of the main absorptive surfaces for drug uptake by the fluke and may be a primary target for anthelmintic action. Studies in F. hepatica have shown that the transtegumental transfer mechanism is critical to achieve sufficient drug concentration at the site of action to exert its flukicidal action (Mottier et al., 2006). Thus, adult forms in the bile ducts are exposed to anthelmintics or its metabolic products eliminated by bile excretion. Although the tegument represents only 1% of the total volume of the adult parasite, the integrity of the surface plasma membrane and the tegumental syncytium is essential for the viability of the fluke. The disruption of the tegument by different mechanisms (including those involved in energy generation) may have severe consequences for the parasite because the drug would penetrate deeper and disrupt also subtegumental tissues. The surface damage will be exacerbated by the surfactant action of the bile. In addition, adult blood-sucking stages may uptake the anthelmintic drugs through the alimentary canal. In fact, most of the fasciolicides have high efficacy against adult flukes due to their extended residence in the systemic circulation as a consequence of their strong plasma protein binding (∼98–99%). Since the introduction of carbon tetrachloride for treatment of helminth infections of animals in the 1920s, numerous other compounds have been investigated for efficacy against F. hepatica. Carbon tetrachloride and other halogenated hydrocarbons have a narrow therapeutic index and their adverse effects (mainly hepatotoxicity) restrict their use for the treatment of fasciolosis. Based on their chemical structure, the fasciolicidal drugs can be classified into several groups: Most of the halogenated hydrocarbons, bisphenolic compounds, the nitrophenolics disophenol, and niclofolan, as well as the bromosalans, are old drugs not widely used today, and have been extensively reviewed in previous editions of this text (see Roberson and Courtney, 1995). This current chapter is focused on description of the pharmacological properties of the most currently used antitrematodal drugs. A schematic representation of the efficacy of different flukicidal drugs against immature and adult stages of F. hepatica is shown in Table 40.1. Table 40.1 Comparative efficacies of the most widely used antitrematodal compounds against different stages of the liver fluke Fasciola hepatica. Source: Fairweather 1999. Reproduced with permission of Elsevier. Nitroxynil (3-iodo-4-hydroxy-5-nitrobenzonitrile) (Figure 40.8) was developed in the late 1960s as an injectable fasciolicide for sheep and cattle. It is a trematodicidal compound highly effective against F. hepatica, which also holds activity against the abomasal nematode Haemonchus contortus. Nitroxynil is highly effective against adult stages of F. hepatica (from 8 weeks postinfection) and F. gigantica. Activity against earlier stages is rather erratic and it is not effective for the treatment of flukes younger than 6 weeks. Moreover, this anthelmintic is used for the control of ivermectin- and benzimidazole-resistant H. contortus in sheep. It is also marketed for the control of Oesophagostomum spp. and Bunostomum spp. in both sheep and cattle, and shows good activity against Parafilaria bovicola infections in cattle. On the contrary, nitroxynil is not effective for the treatment of paramphistomes in ruminants. Figure 40.8 Chemical structure of the nitrophenolic compound nitroxynil. Due to the structural similarity between nitrophenols and 2,4-dinitrophenol, a known uncoupler of oxidative phosphorylation, it was assumed that nitroxynil and related compounds act in a similar way in the fluke. However, there is little direct evidence showing this mechanism of action for these fasciolicides. It has been shown that nitroxynil produced a rapid spastic paralysis (within 3 hours) of the fluke in vitro at similar concentrations to those found in the systemic circulation in vivo (Fairweather et al., 1984). This action was not believed to be consequence of an uncoupling effect on the oxidative phosphorylation (Fairweather and Boray, 1999). More recently, it was shown that nitroxynil causes severe disruption of the tegument of F. hepatica, both in vitro and in vivo (McKinstry et al., 2003). Liver flukes recovered from rats after 24 hours of nitroxynil treatment showed extensive swelling and blebbing of the tegument in both dorsal and ventral surfaces. These changes became more severe after 48 and 72 hours following nitroxynil administration and specimens recovered following these posttreatment intervals showed more widespread tegumental loss. Although the intrinsic mechanism of nitroxynil action on the parasite has not been established, it was shown that the tegument is an important target for this flukicidal drug. Although nitroxynil can be administered orally or intraruminally (IR), it is more effective when administered by parenteral routes (SC, IM). A ruminal microflora-mediated nitroreduction may account for its low efficacy after oral or IR treatments. Solutions (25% or 34%) of nitroxynil-N
Anticestodal and Antitrematodal Drugs
Anticestodal Drugs
Introduction
Bunamidine
Niclosamide
Praziquantel
Epsiprantel
Antitrematodal Drugs
Introduction
Nitrophenolic Compounds
Nitroxynil
Stay updated, free articles. Join our Telegram channel

Full access? Get Clinical Tree
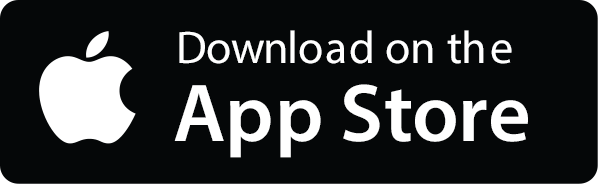
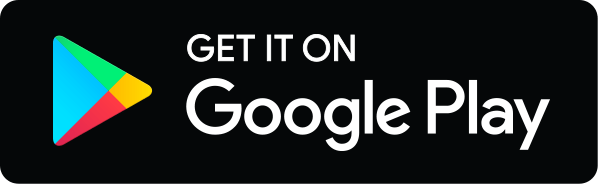