© Springer International Publishing Switzerland 2016
Chi-Chao Chan (ed.)Animal Models of Ophthalmic DiseasesEssentials in Ophthalmology10.1007/978-3-319-19434-9_11. Animal Models of Herpes Keratitis
(1)
Department of Ophthalmology, University of Pittsburgh School of Medicine, 15213 Pittsburgh, PA, USA
(2)
Department of Immunology, University of Pittsburgh School of Medicine, 15213 Pittsburgh, PA, USA
(3)
Department of Microbiology and Molecular Genetics, and Graduate Training Program in Molecular Virology and Microbiology, University of Pittsburgh School of Medicine, 15213 Pittsburgh, PA, USA
(4)
Department of Ophthalmology, and Graduate Training Program in Molecular Virology and Microbiology, University of Pittsburgh, Pittsburgh, PA, USA
Keywords
Herpes simplex virusHerpes stromal keratitis (HSK)MouseCorneaCorneal nervesCD4+ T cells1.1 Introduction to Herpes Keratitis
Herpes simplex virus type 1 (HSV-1) is a prevalent pathogen. Based on serologic testing, more than half of the human population in the USA (much higher in some developing countries) has been infected with HSV by age 70 (Xu et al. 2002) , and recent studies of human cadavers suggest that the frequency of human TG harboring latent HSV-1 might be even higher than that suggested by serology (Hill et al. 2008) . Herpes keratitis is an ocular condition that usually results from remittent-recurrent HSV-1 infections of the eye. In both humans and in a variety of animal models of herpes keratitis, primary HSV-1 infection of the eye or oral facial region leads to HSV-1 colonization of many sensory neurons in the trigeminal ganglion (TG). The virus has a special relationship with neurons in which the viral genome is retained as episomal DNA in the nucleus of the neuron in a quiescent state called latency. HSV-1 genomes can persist in the latent state in neurons throughout the lifetime of the person, or can periodically reactivate from latency, produce infectious virions, and transport these virions by axonal transport back to the corneal surface. These reactivation events can trigger recurrent herpes keratitis characterized by lesions that are restricted to the corneal epithelium and caused by virus replication and destruction of epithelial cells, or are focused in the stroma and are immunopathological in nature. The latter form is referred to as herpes stromal keratitis (HSK) or interstitial keratitis, and can lead to blindness due to progressive scarring of the cornea with recurrences. Recurrent HSK is the leading infectious cause of blindness in the USA and worldwide.
1.2 HSV-1 Latency
HSV-1 latency can be defined in molecular terms as retention of episomal viral DNA in the nucleus of a neuron with transcription of viral genes restricted to a family of latency-associated transcripts (LATs) that do not appear to be transcribed (Javier et al. 1988; Stevens et al. 1988) . Alternatively, latency can be defined as retention of episomal HSV-1 DNA without production of infectious viral particles (Gardella et al. 1984) . The latter definition permits low-level intermittent production of a limited array of viral lytic gene products without virus production during latency, which is supported by a growing body of data on latently infected mice (Kramer and Coen 1995; Feldman et al. 2002) . These findings also raise the possibility that the immune system can respond to viral lytic gene products before the virus escapes latency, a concept that is also supported by experimental data.
Since reactivation from the latent state predisposes to recurrent herpes keratitis, a great deal of emphasis has been placed on studies of herpes latency and reactivation in animal models . These studies have demonstrated that ill-defined genetic characteristics of the virus determine the likelihood of a reactivation event. For instance, infection of rabbit corneas with the HSV-1 strains McKrae and Syn + results in spontaneous reactivation from latency in the TG, whereas spontaneous reactivation does not occur following similar infections with strains such as KOS (Hill et al. 1987b) . However, even with the more neurovirulent McKrae and Syn + strains, spontaneous reactivation does not typically result in recurrent keratitis in rabbits. Moreover, even corneal infection with the more virulent McKrae and Syn + strains does not typically result in spontaneous reactivation in mice. However, reactivation of the McKrae and Syn + strains can lead to a high incidence of recurrent herpes keratitis following inductive signals to the cornea such as epinephrine in rabbits (Hill et al. 1987a, 1987b, 1987c; Berman and Hill 1985) and ultraviolet irradiation in mice (recently reviewed in Stuart and Keadle 2012) .
Evidence in mice suggests that both viral- and host-encoded microRNAs (miRNAs), many encoded within the viral LATs, can repress HSV-1 lytic gene expression during latency (Umbach et al. 2008; Pan et al. 2014) . A recent study suggested that siRNAs that regulate the neurovirulence factor γ34.5 may contribute to latency (Tang et al. 2008) . Interestingly, it appears that the LAT region might function differently in latently infected mice and rabbits. HSV-1 deletion mutants lacking LAT show reduced lytic gene expression in mice (Leib et al. 1989; Garber et al. 1997) , but dramatically increased lytic gene expression in rabbits during latency (Giordani et al. 2008) . These species-specific differences in LAT function might maintain the viral genome in a more activated state in rabbits than in mice, accounting for spontaneous reactivation in rabbits. Recent evidence also suggests a role for epigenetic repression of viral gene expression during latency (Bloom et al. 2010) . Using a mouse footpad infection model, the authors demonstrated that during latency the LAT locus is enriched for euchromatin marks associated with gene expression, while lytic genes are enriched for heterochromatin marks, consistent with lytic gene repression. Moreover, a growing body of evidence establishes that T cells (both CD8+ and CD4+ T cells) surround latently infected TG neurons in both mouse and human TG, and that CD8+ T cells can block HSV-1 reactivation from a latent state both in vivo and in vitro in mice (reviewed in Rowe et al. 2013) . Many of the CD8+ T cells surrounding latently infected neurons in TG of both mice and humans exhibit an activation phenotype including expression of the lytic granule component granzyme B and production of interferon gamma (IFN-γ) (Knickelbein et al. 2008; Liu et al. 2000; Verjans et al. 2007; Theil et al. 2003) . In mice CD8+ T cells have been shown to form an immunologic synapse with neurons and to even release lytic granules into the synapse (Khanna et al. 2003; Knickelbein et al. 2008) . Mice can use both IFN-γ and lytic granules to prevent HSV-1 reactivation without killing the infected neurons. These observations in latently infected mice call into question the once prevalent view that latent HSV-1 is ignored by the host immune system, and this has led many to hypothesize a more dynamic form of latency in which the virus is constantly trying to reactivate in a small number of neurons, but these attempts are counteracted in part by the surveillance of CD8+ T cells and perhaps also CD4+ T cells .
An interesting difference between mice and humans is the greatly increased sensitivity of transporters of antigen presentation (TAPs) to inhibition by the HSV-1 immediate early protein infected cell protein 47 (ICP47) in humans relative to mice. The important role of TAPs in loading peptides onto major histocompatibility complex (MHC) class I molecules for presentation to CD8+ T cells suggests that HSV-1-infected cells might be less visible to CD8+ T cells in humans than in mice. This effect might be more profound in latently infected neurons that express very low levels of MHC and viral proteins, and could account for the highly increased rate of spontaneous reactivation of HSV-1 in human TG relative to mouse TG. However, the fact that CD8+ T cells surround latently infected neurons and show a similar activation phenotype in mice and human TG would seem to militate against this theory.
Identification of these immunologic and non-immunologic mechanisms of regulating HSV-1 gene expression during latency informed current attempts to understand how these repressive mechanisms are regulated in animals. A variety of environmental and physiological stimuli (e.g., stress, exposure to UV irradiation, and immunosuppression) are associated with HSV-1 reactivation in both mice and humans. One common thread between these stimuli and HSV-1 reactivation is the association of both with suppression of T-cell function. For instance, the exposure of latently infected mice to restraint stress significantly inhibits the function of CD8+ T cells that surround latently infected neurons in their TG and leads to HSV-1 reactivation from latency (Freeman et al. 2007) . Moreover, persistent antigenic exposure of HSV-specific CD8+ T cells in latently infected TG of mice can lead to a phenomenon called T-cell exhaustion, which is associated with a loss of T-cell function and a corresponding reduction in CD8+ T-cell protection from reactivation (Jeon et al. 2013; St Leger et al. 2013) . These findings suggest that bolstering the number and function of CD8+ T cells in latently infected ganglia might represent an effective approach to reduce the rate of recurrent herpetic disease.
Given the apparently important role of HSV-specific CD8+ T cells in controlling HSV-1 latency, one might predict that HSV-1 vaccines targeting CD8+ T cells would be effective in preventing HSV-1 reactivation from latency. In fact, this approach has been advocated based on ex vivo studies with latently infected mouse TG (Hoshino et al. 2007) . However, a recent study provided the important caveat that adoptively transferred HSV-specific CD8+ T cells (mimicking the effect of vaccination) were completely excluded from the TG after HSV-1 latency was established, even following induction of HSV-1 reactivation (Himmelein et al. 2011) . These findings suggest that simply increasing the number and frequency of circulating HSV-specific CD8+ T cells through vaccination might not be sufficient to increase the CD8+ T cell population that is resident in latently infected TG. More heroic measures such as the use of neurotrophic viral vectors to express chemokines within the latently infected TG might be necessary adjunct to vaccination for this purpose.
1.3 HSV-1 Keratitis
Unfortunately, HSV-1 is able to escape the rather significant mechanisms for viral gene repression in many humans and frequently reactivates and is shed at the corneal surface. The consequences of such reactivation and shedding events can vary significantly in different individuals. In many individuals viral shedding into the tear film is completely asymptomatic (Kaufman et al. 2005) . In others, viral reactivation and shedding can lead to recurrent lesions that are restricted to the corneal epithelium, referred to as infectious epithelial keratitis (Darougar et al. 1985) . The lesions are caused by HSV-1 replication and destruction of epithelial cells, and for reasons that are not clear typically have a dendritic or geographic morphology. Still, in other individuals, HSV-1 reactivation and shedding can lead to recurrent bouts of HSK or interstitial keratitis. HSK is characterized by mild to severe inflammation in the corneal stroma with or without an overlying epithelial necrosis, referred to as necrotizing or immune HSK, respectively. This variability in HSV-1-induced corneal pathology appears to reflect the combined effect of genetic factors in the virus and the host.
These so-called “genetics squared” effects are difficult to study in outbred rabbits, but can be modeled to some extent in mice where different laboratory strains of HSV-1 induce varying levels of corneal disease in different inbred strains of mice. For instance, primary corneal infection of BALB/c mice with the KOS strain of HSV-1 induces epithelial lesions with subsequent development of necrotizing or immune HSK, whereas a similar infection of the corneas of C57BL/6 mice results only in epithelial disease without progression to HSK. In contrast, the RE strain of HSV-1 induces both epithelial lesions and progression to HSK following corneal infection of both BALB/c and C57BL/6 mice (Fenton et al. 2002) . The complexity of the viral genome (containing approximately 86 open-reading frames) and the host genome has thus far defied rendering of a genetic explanation for this disease variability. However, recent advances in the RNA and DNA sequencing technology will likely increase the feasibility of such studies in the near future.
1.3.1 HSV-1 Epithelial Keratitis
HSV-1 epithelial keratitis has been studied extensively in rabbits and mice. In both the models the lesions have the characteristic dendritic or geographic morphology seen in humans. The duration of the epithelial lesions is somewhat longer in rabbits, making them a popular model system for studying the efficacy of antiviral drugs. Moreover, one can readily induce recurrent HSV-1 corneal epithelial lesions in rabbits by approaches such as corneal iontophoresis of epinephrine (Santos et al. 1987) . Immune mechanisms that control HSV-1 replication in the corneal epithelium have been mainly studied in mouse primary corneal infection models. Since the resulting epithelial lesions heal within about 3–4 days, and the virus is typically cleared from the cornea by 7–8 days, it is not surprising that the innate immune response is primarily involved in viral clearance from the cornea in the mouse primary infection model. Mice lacking type-1 interferon (IFN-α and IFN-β) responses not only fail to clear the virus from the cornea but also experience disseminated lethal infections (Luker et al. 2003; Noisakran and Carr 2000; Carr and Noisakran 2002) (Hendricks et al. 1991) . Mice lacking type 2 (IFN-γ) responses are less compromised, but do show delayed HSV-1 clearance from the cornea (Frank et al. 2012) . Evidence suggests that viral clearance is primarily mediated by the combined action of the innate immune cells: natural killer (NK) cells, monocyte/macrophages , and cells expressing a γ/δ T-cell receptor (Ghiasi et al. 2000; Bukowski and Welsh 1986; Sciammas et al. 1997) . Recent evidence demonstrates an important role of cornea-resident dendritic cells (DC) in regulating the migration of NK cells and monocytes into the HSV-1 corneal lesion (Frank et al. 2012) . It should be noted, however, that most human corneal epithelial HSV-1 lesions result from reactivation of the latent virus and occur in the face of an established adaptive immune response. Therefore, the relative contribution of the innate- and adaptive immune system to HSV-1 clearance from the corneal epithelium needs to be examined in mouse or rabbit recurrent disease models.
1.3.2 Herpes Stromal Keratitis
HSK represents an immunopathological response that can occur in the absence of detectable replicating virus in both mice and humans. The disease is characterized by progressive leukocytic infiltration, opacity, and vascularization of the cornea that with recurrences can lead to progressive corneal scarring. From the seminal observation in mice that T cells are necessary for the development of HSK, mice have become by far the most popular model for studying the disease (Metcalf et al. 1979) . In mice, HSK initiates around 7–8 days postinfection (dpi), after the detectable replicating virus has cleared from the cornea and enters a latent state in the TG. Following primary infection, HSK appears to be preferentially mediated by CD4+ T cells in mice, although the infection of CD4+ T-cell-deficient mice with a relatively high dose of HSV-1 leads to CD8+ T-cell-mediated severe, but transient HSK that begins to resolve by around 20 dpi (Lepisto et al. 2006) . HSK induced by primary infection of the mouse cornea is characterized by a diffuse inflammatory infiltrate in the corneal stroma consisting primarily of neutrophils with smaller, but significant populations of macrophages and CD4 T lymphocytes (Hendricks and Tumpey 1990) . Their corneas become vascularized and edematous and the inflammation persists for months. Humans can develop similar severe HSK, but in most the disease is milder, more focal, and more transient. The difference might in part reflect the fact that HSK represents recurrent disease in most humans, but develops following primary infection in most mouse models . Indeed, when recurrent disease is induced in mice, HSK tends to be milder with focal stromal opacities (Miller et al. 1996) . Histological examination of human corneas with HSK is usually restricted to corneal buttons removed during transplantation, which is typically performed only after inflammation subsides, so the leukocytic infiltrate is almost certainly not a reflection of that present during active HSK.
1.3.2.1 Neurotrophic Damage as a Major Component of HSK in Mice
Neurotrophic keratitis is corneal pathology resulting from impairment of trigeminal corneal innervation leading to a decrease or an absence of corneal sensation (Cruzat et al. 2011) . The most frequent cause of corneal anesthesia is viral infection (especially herpes simplex and varicella zoster), followed by chemical burns, physical injuries, and corneal surgery. Corneal hypoesthesia (reduced sensation) is a hallmark of HSK in both mice and humans. The cornea is primarily innervated through the ophthalmic branch of the trigeminal nerve that is comprised of the frontal, lacrimal, and nasociliary nerves, with the latter providing sensory innervation of the cornea. The nasociliary nerves with a density of as many as 100 nerve endings per 0.01 mm2 of corneal surface are responsible for the blink reflex that helps to maintain tear film protection of the corneal surface and prevents corneal inflammation associated with exposure keratitis. In vivo confocal microscopy reveals that the loss of corneal sensation in HSK correlates strongly with profound diminishment of the subbasal nerve plexus after HSV infection. A similar, though less profound retraction of the subbasal nerves can result from bacterial and fungal infections (Hamrah et al. 2010) . Whether hypoesthesia associated with HSK is the direct effect of HSV-1 infection of nasociliary nerves, is caused by corneal inflammation, or results from a combination of both is unclear. The loss of sensitivity in human corneas with HSK rarely recovers, leading to the prevalent view that nerve loss is permanent.
Although the loss of corneal sensitivity and corneal nerves has been demonstrated in mouse models of HSK , until recently the contribution of nerve loss to corneal pathology was not evaluated. Mice lack consensual blink reflex (Yun et al. 2014) , a reflex arc in humans that causes both eyes to blink when one cornea is irritated. Therefore, mice likely experience a more profound loss of blink reflex when corneal nerves are damaged by unilateral HSK. Infecting the corneas of BALB/c mice with 1 × 105 pfu of HSV-1 (RE or KOS strain) results in severe vascularization and dense corneal opacity that persists for at least 70 days after infection, and is associated with loss of corneal tactile sensation based on the loss of blink reflex (Yun et al. 2014) . The loss of corneal blink reflex was associated with a dramatic reduction of corneal nerve fibers mainly involving the sensory endings and the plexus of nerve fibers extending from trunks in the corneal stroma into the epithelial layer of the cornea. Since the loss of corneal blink reflex can result in corneal desiccation and exposure keratopathy, the authors asked if the severe and chronic inflammation observed in the mouse HSK model could be ameliorated by protecting the cornea from desiccation by performing tarsorrhaphy. Tarsorrhaphy (stitching the eyelid closed) is a procedure commonly used in the clinic to protect corneas from desiccation. They observed that tarsorrhaphy could not only dramatically reduce inflammation in mouse corneas with HSK but also prevent the development of severe HSK when performed prior to its onset. Tarsorrhaphy did not prevent or repair corneal nerve damage or the associated loss of blink reflex, but did reduce inflammation associated with the corneal desiccation. It appears, therefore, that the severe and chronic inflammation associated with HSK in mice actually reflects exposure keratopathy associated with the inability to blink in response to corneal irritation. Thus, HSK in mice appears to reflect two distinct processes: a mild and potentially transient immunopathological response (presumably to HSV-1 antigens) resembling that seen in most humans; followed by a chronic severe immunopathological response to corneal desiccation.
Only gold members can continue reading. Log In or Register to continue
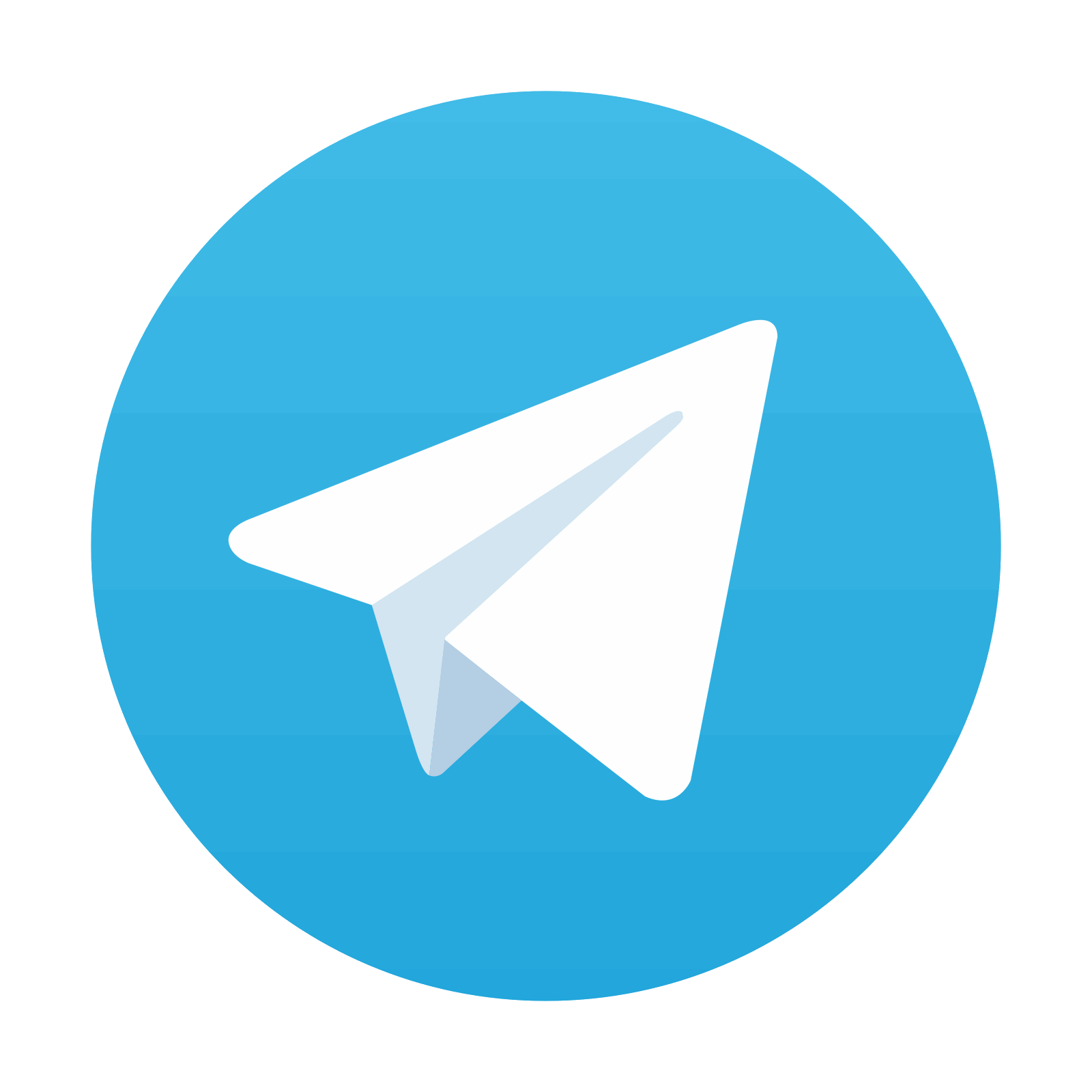
Stay updated, free articles. Join our Telegram channel

Full access? Get Clinical Tree
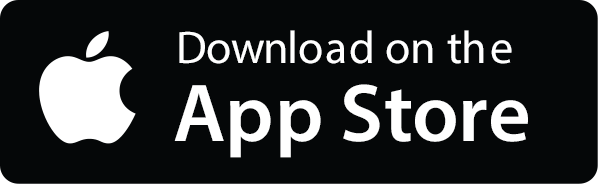
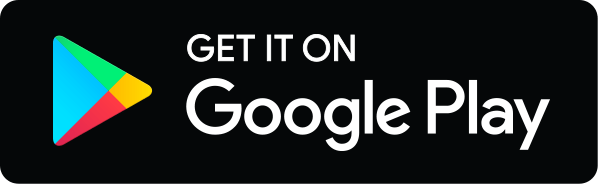