© Springer International Publishing Switzerland 2016
Chi-Chao Chan (ed.)Animal Models of Ophthalmic DiseasesEssentials in Ophthalmology10.1007/978-3-319-19434-9_33. Animal Models of Glaucoma
(1)
Wilmer Eye Institute, Johns Hopkins Hospital, 600 N Wolfe Street, Wilmer B-29, 21287 Baltimore, MD, USA
(2)
Section on Retinal Ganglion Cell Biology, Laboratory of Retinal Cell and Molecular Biology, National Eye Institute, National Institutes of Health, 6 Center Drive, MSC 0608, Building 6, Room 212, 20892 Bethesda, MD, USA
3.1 Introduction
Glaucoma represents a heterogeneous group of chronically progressive optic neuropathies. It is characterized by progressive retinal ganglion cell (RGC) death and axon loss manifesting as retinal nerve fiber layer thinning and optic nerve head (ONH) cupping, which functionally result in a characteristic pattern of visual field loss that begins peripherally, sparing central fixation until late in the disease course. Glaucoma is a major heath burden worldwide, projected to affect more than 110 million people by the year 2040 (Tham et al. 2014) . Animal glaucoma models are critical to our understanding of the pathogenesis of the disease and the development of new therapies to prevent blindness. Similar to all animal models of disease, glaucoma models are most valuable when they are readily accessible, efficient for experimentation, and share important pathophysiological and treatment-response characteristics with the human condition, thereby facilitating clinical translation and improving patient outcomes (Casson et al. 2012) . In this chapter, we review the most commonly used rodent models of glaucoma while evaluating their strengths and weaknesses in simulating various aspects of human glaucoma.
The most important modifiable risk factor for the onset and progression of glaucoma in humans is IOP ; however, it is notable that the 5-year risk of developing glaucoma for people with IOP greater than 21 mmHg is less than 10 % (Kass et al. 2002) , and at least 30–40 % of patients with glaucoma have a pretreatment IOP of less than 21 mmHg (Sommer et al. 1991; Dielemans et al. 1994; Mitchell et al. 1996) , a figure that increases significantly for persons of East Asian descent (Iwase et al. 2004; Kim et al. 2011) . Nonetheless, therapeutic reduction of IOP reduces the risk of glaucomatous conversion in patients with ocular hypertension (Kass et al. 2002) and of progression in patients with glaucoma and IOP less than 21 mmHg (Collaborative Normal-Tension Glaucoma Study Group 1998a, b). As such, IOP appears to play an important role in the pathogenesis of glaucoma, and so the animal models generally regarded as most applicable to human disease tend to be those which achieve RGC degeneration via experimental elevation of IOP. At least with regard to medications aimed at lowering IOP, ocular hypertensive animal models of glaucoma are better able to identify those drugs that will go on to commercial availability (Stewart et al. 2011) . Alternative models that trigger RGC death through more direct toxic or traumatic mechanisms, such as optic nerve crush or transection, intraocular injection of excitotoxic compounds, ischemia/reperfusion, or induced autoimmune models, are useful for understanding RGC pathophysiology in general, but may be less directly applicable to clinical glaucoma and will not be discussed here.
3.2 Rodent Glaucoma Models
Mice and rats are attractive model systems for human disease because of their reasonable approximation to human ocular physiology, low cost, ease of maintenance, relatively short life cycle, and amenability to genetic manipulation. Anatomically, rodent and human eyes share many important characteristics including the anatomy of the anterior chamber drainage structures and physiology of aqueous humor dynamics that can be experimentally manipulated to elevate IOP . It should be noted, however, that rodents exhibit a glial rather than fibrous lamina cribrosa. This could be an important physiological difference given that the site of RGC injury in primates and humans is thought to arise from axonal interactions with the lamina cribrosa, which has prompted a recent emphasis on clinical evaluation of this tissue (Kim et al. 2013) . Another drawback of rodent models of ophthalmic disease , in particular, has been the relatively small ocular size making certain procedures and manipulations (i.e., vitreal sampling and injection) technically more difficult than in larger mammals. Nonetheless, rodent models of glaucoma dominate research within the field because of their broad accessibility and versatility. The relatively recent advent of rebound tonometry has improved researchers’ ability to accurately measure IOP quickly and repeatedly in awake or anesthetized rodents (Johnson et al. 2008; Morrison et al. 2009; Pease et al. 2011) , and is another factor that has led to an increased popularity of rodent glaucoma models in recent years.
Rodent glaucoma models can be broadly classified into those with ocular hypertension induced experimentally and those occurring genetically . However, these artificial boundaries are now being blurred by newer techniques that induce ocular hypertension by viral gene transfer (see below) and by experiments that take advantage of mouse genetics to induce IOP in animals with gene-encoded cell-specific labels and/or pathogenesis-related mutations of interest. For instance, several mouse strains that express various fluorescent proteins under control of the RGC-specific gene Thy-1 promoter (Feng et al. 2000) are becoming popular for studying RGC morphology and survival in conjunction with IOP-dependent models of experimentally induced glaucoma (Raymond et al. 2009; Tsuruga et al. 2012; Williams et al. 2013) .
We and others recently reviewed the benefits and limitations of several important rodent models of glaucoma (Pang and Clark 2007; Howell et al. 2008; McKinnon et al. 2009; Johnson and Tomarev 2010) . Here, we will briefly revisit the most popular of the ocular hypertensive rodent glaucoma models and discuss more recent developments in the generation of new rodent glaucoma models.
3.3 Episcleral Vein Injection/Ablation
The earliest rodent models of IOP-dependent glaucoma involved manipulation of the episcleral veins leading to reduced aqueous humor drainage from the eye. In the mid-1990s, Morrison and colleagues (Morrison et al. 1997) performed intravenous injection of hypertonic (1.65–1.85M) saline into the episcleral vessels of adult rats, which causes trabecular meshwork (TM) sclerosis and formation of anterior synechiae, thereby reducing aqueous humor outflow. This leads to an increase in IOP of roughly between 5 and 25 mmHg that lasts for, on average, 1–5 weeks. Cumulative IOP exposure in this model correlates with RGC axon loss and ONH cupping (Chauhan et al. 2002) . Limitations of this technique include the technical difficulty of cannulating the episcleral veins with a custom glass microneedle and the relative variability in the extent and duration of IOP elevation, which sometimes requires reinjection. The method has also been adapted to induce ocular hypertension in the smaller eyes of mice; however, IOP elevation was limited to <5 mmHg and required multiple injections in 80 % of eyes (Kipfer-Kauer et al. 2010) . In addition, the method has proven useful in demonstrating protection from optic nerve damage by therapeutic IOP-lowering interventions (Morrison et al. 1998) . Recent evidence suggests that this method is associated with structural and functional degeneration of outer retinal neurons in addition to RGCs at relatively late time points well after IOP has returned to baseline (16–26 weeks after induction of ocular hypertension), although visual evoked potentials recorded from the visual cortex remained preserved (Georgiou et al. 2014) . This may imply a particular utility for this model in studying the late-term effects of advanced glaucoma on non-RGC retinal neurons.
Another method for experimental IOP elevation in rats also first reported in the 1990s is episcleral vein cauterization (Shareef et al. 1995) . The IOP response to cauterization is dose-dependent with respect to the number of episcleral veins involved, with one vessel causing no appreciable IOP elevation and four vessels causing IOP elevation to around 60 mmHg with subsequent proptosis and corneal decompensation. Cauterization of two or three vessels, however, results in increase of IOP to 20–30 mmHg that is sustained for at least several weeks and is associated with progressive RGC apoptosis (Garcia-Valenzuela et al. 1995) . An analogous method has more recently been applied to mice with comparable results (Ruiz-Ederra and Verkman 2006) . Given that ocular blood flow is disrupted in this method, concern exists that retinal vascular congestion may lead to secondary effects beyond that of IOP elevation alone. Still, recent re-evaluations of this method, and variations thereof, have confirmed its relative ease and reproducibility, and its apparent similarity to human glaucoma with progressive loss of RGC structure and function (Mittag et al. 2000; Bai et al. 2014) . The fact that IOP can be maintained at moderate levels (approximately 30 mmHg) for extended periods of time—up to 16 weeks (Urcola et al. 2006) —makes this model especially useful for long-term studies and arguably approximates chronic human glaucoma better than more acute models of IOP elevation.
3.4 Translimbal Laser Photocoagulation
Photocoagulation of the TM and anterior chamber drainage angle structures is a widely employed technique for reducing aqueous humor outflow and increasing IOP in mice and rats. An early study utilized the method of injecting India ink into the anterior chamber to enhance laser uptake into the TM (Ueda et al. 1998) , but more recent adaptations of this technique have shown that IOP elevation is attainable without pigmentary pretreatment. Levkovitch-Verbin et al. (Levkovitch-Verbin et al. 2002) compared four different laser photocoagulation approaches and found that circumferential treatment of the anterior chamber angle without treatment of the episcleral veins resulted in IOP elevation initially to the 40–50 mmHg range that slowly dropped back to baseline over about 3 weeks and caused slowly progressive optic nerve damage. Of note, this technique often requires multiple laser treatments to sustain IOP elevation over the course of weeks. An adaptation of this technique involves paracentesis to flatten the anterior chamber and induce iridocorneal contact at the time of laser treatment, which results in sustained IOP elevation for at least 2–3 weeks without the need for retreatment (Biermann et al. 2012) . The intensity of laser power that is used for photocoagulation is an important procedural consideration, and one must take into account whether the animal is pigmented or albino, since trabecular and iris pigment increases laser uptake. According to the protocol, pigmented rats generally require 100 mW for 50–300 ms duration, whereas albino rats require 400–600 mW for 500–700 ms duration. An important factor complicating this technique is corneal decompensation that manifests in a subset of animals and may be related to limbal stem cell destruction from laser treatment.
Mice are also amenable to induction of ocular hypertension by translimbal laser photocoagulation. Pigmentary pretreatment with indocyanine green followed by diode photocoagulation leads to IOP elevation for at least 30 days with subsequent loss of RGC structure and function (Grozdanic et al. 2003) . Flattening of the anterior chamber via paracentesis appears to be more critical in this species, and leads to longer periods of IOP elevation, with one report suggesting that IOP remains above 20 mmHg for 4 months following photocoagulation (Feng et al. 2013a) . Without these adaptations, translimbal laser photocoagulation in the mouse leads to a doubling of IOP that lasts only for 7 days (Fu and Sretavan 2010) . Repeated laser photocoagulation 1 week after the first treatment was reported to increase the mean IOP above 20 mmHg in 57 % of treated eyes at 24 weeks. Treated eyes demonstrated a 59 % reduction in the average axon number as well as a significant decrease in the photopic negative ERG response (Yun et al. 2014) .
Recent investigations have identified important neuropathological characteristics of this model. RGC loss following laser trabecular meshwork photocoagulation appears to be topographically sectorial (Soto et al. 2011) , which is similar to the pattern of degeneration seen in other models, such as the DBA/2J mouse. Translimbal laser photocoagulation of Thy1-YFP animals has demonstrated a topographic pattern for RGC dendritic tree degeneration that precedes changes to cell soma morphology and begins along the retinal vertical axis (Feng et al. 2013b) . Whereas retrograde labeling of RGCs via application of tracers such as fluorogold to the superior colliculus or transected optic nerve stump is often employed to identify and quantify cell survival, a proportion of RGCs are only identifiable through alternative immunohistochemical methods (gamma synuclein or phosphorylated neurofilament), likely because of axonal disruption prior to overt cell death (Soto et al. 2011) . This proportion appears to be dependent on the degree of RGC degeneration that is triggered and equates to about 10 % with mild degeneration but up to 85 % in cases of severe degeneration (Soto et al. 2011) . This may have important implications for the utilization of glaucoma animal models in general, where retrograde RGC labeling is a common method for quantification of neurodegeneration .
3.5 Microbead Injection
The aforementioned glaucoma models involve obstruction to aqueous humor outflow by physical destruction of anatomical drainage structures. Alternative methods aim to obstruct outflow through the introduction of exogenous substances into the outflow pathway while leaving the biological structures intact, which is likely less inflammatory. Weekly injections of chondroitin sulfate into the anterior chamber is capable of raising IOP in rats to more than 20 mmHg and inducing subsequent RGC loss and electrophysiological changes, but is labor intensive (Belforte et al. 2012) . An early approach with microbead injection in rats involved anterior chamber injection of 10-µm latex microspheres with or without viscous hydroxypropylmethylcelluose, but this still required multiple injections to attain sustained IOP elevation (Urcola et al. 2006) . Direct comparisons to episcleral vein cauterization show that microspheres with or without hydroxypropylmethylcelluose were capable of attaining sustained IOP elevation to moderate levels (30–40 mmHg) for a longer period of time (more than 30 weeks) with a comparable level of RGC loss at comparable time points (Urcola et al. 2006) . Subsequent work has refined the technique to define protocols for reproducible IOP elevation in both mice and rats. Calkins and colleagues (Sappington et al. 2010) evaluated beads measuring 5–15 μm and found that 15-μm beads raised IOP by a modest level of 5–10 mmHg in rats for 2 weeks and in mice for 3 weeks following a single injection, with ocular hypertensive periods extended to 8 weeks in rats injected twice. A variant of this technique in mice utilizes a mixture of 1- and 6-μm beads injected into the anterior chamber followed by viscous sodium hyaluronate, which acts to direct the beads into the anterior chamber angle and maintains elevated IOP for approximately 2 weeks following a single injection (Cone et al. 2012) . The injection of a mixture of 6- and 10-μm beads was also used for reproducible and persistent IOP elevation in rats (Smedowski et al. 2014) . The relative technical simplicity of this model, low cost, and high degree of reproducibility has provided a system in which relatively large experiments can be conducted with efficiency, leading to a recent increase in new data regarding the pathogenesis of glaucoma .
The effects of clinically used topic ocular hypotensive medications have been evaluated using the microbread model, where experiments have demonstrated a strong IOP lowering response to aqueous suppressants but not to medications that increase outflow (Yang et al. 2012) . Recent investigations using the microbead model in mice have elucidated important differences in age and strain-specific responses to experimental glaucoma. Microbead injection induces a greater rise in IOP level when performed in 8-month old as compared with 2-month-old C57BL/6 mice, but this does not hold for CD1 mice (Cone et al. 2012) . In addition, older C57BL/6 mice appear to be more resistant to RGC soma and axonal degeneration than younger mice of the same strain, even when statistically corrected for differential IOP exposure, and this effect is associated with less ocular axial elongation in response to ocular hypertension (Cone et al. 2010) . CD1 mice, on the other hand, tend to lose more axons than other strains, even when normalizing for IOP exposure (Cone et al. 2010), and older CD1 mice are more susceptible to RGC loss at a given IOP level than younger CD1 mice (Steinhart et al. 2014) . Individual RGC classes are also differentially susceptible to IOP-associated cell dysfunction and death as shown in this model, with OFF-transient RGCs showing the earliest structural and functional perturbations (Della Santina et al. 2013) . Recent studies using this glaucoma model have begun to elucidate an important role for connective tissue biomechanics in the susceptibility of the optic nerve to neurodegeneration in the face of elevated IOP. Whereas elastin haploinsufficient animals and fibromodulin knockout mice exhibit similar rates of RGC loss as their wild-type (WT) littermates (Steinhart et al. 2014) , mice with the Aca23 mutation in collagen 8A2 have eyes that enlarge to sustained ocular hypertension but are resistant to neurodegeneration (Steinhart et al. 2012) .
3.6 Other Models of Induced Ocular Hypertension
An alternative method for inducing elevated IOP in rodents includes viral gene transfer. This approach has the added benefit of generally sustained effects on IOP following a single treatment; however, it benefits from increased flexibility over inborn genetic models of glaucoma in that the timing and in some cases extent of IOP elevation can be controlled by the investigator, and contralateral eyes are available to serve as internal controls. Several genes have been shown to increase IOP when transferred to anterior chamber cells. Bone morphogenetic protein-2 was transduced into TM cells by intracameral injection of modified cytomegalovirus (CMV) expressing the gene. This resulted in calcification of the aqueous outflow pathway and elevation of IOP to 20–30 mmHg for at least a month following a single treatment, which was normalized by topical prostaglandin-analog therapy (Buie et al. 2013) . Intravitreal injection of adenovirus encoding soluble CD44 reduced outflow facility in mice, which elevated IOP to nearly 30 mmHg for a period of 50 days following treatment (Giovingo et al. 2013) . Similarly, viral transduction of anterior chamber outflow tract cells with transforming growth factor-β2 (TGF-β2) results in increased IOP in both mice and rats, although the effect decreased over the course of about 2 weeks in rats and 3 weeks in mice (Shepard et al. 2010) . Interestingly, viral transduction of TM cells in mice with herpes thymidine kinase followed by systemic gancyclovir administration has been used to specifically ablate aqueous outflow pathway cells, thereby reducing rather than elevating IOP (Zhang et al. 2014) . This model may prove useful for studying methods to regenerate TM, as meshwork cellularity recovered following ablation (Zhang et al. 2014) . Corticosteroid treatment has also been explored as a method for inducing ocular hypertensive glaucoma in rodents, stemming from the tendency of steroids to trigger glaucoma in human patients. Whereas subconjunctival injection of triamcinolone reduces outflow facility in mice but does not alter IOP (Kumar et al. 2013) , topical application of dexamethasone three times daily has been shown to increase IOP (Zode et al. 2014) .The effect, however, was relatively modest with IOP increasing by only 3 mmHg after 2 weeks of treatment and rising to 8 mmHg at 6 weeks. This did lead to detectable RGC death and optic nerve degeneration; however, the labor intensity of the experimental regimen may limit its utility to studying steroid-induced glaucoma specifically. A potentially more versatile model of steroid-induced glaucoma involves dexamethasone delivery by a subcutaneously implanted osmotic mini-pump in mice. This led to an IOP increase by about 2.5 mmHg over 3 weeks, a decreased conventional outflow facility by 52 % versus control, and increased fibrillar material in the TM (Overby et al. 2014) .
3.7 Genetic Mouse and Rat Models
Several genetic models have been described that mimic different types of glaucoma, including primary open angle glaucoma (POAG) that occurs with or without elevation of IOP (the latter is also sometimes referred to as normal tension glaucoma or NTG), primary angle closure glaucoma (PACG), pseudoexfoliation syndrome often associated with glaucoma, and congenital glaucoma. Genes known to be involved in human glaucoma have very often been targeted for the development of new mouse models of the disease. Interestingly, however, the observed pathological changes detected in mouse eyes after modification of glaucoma-associated genes sometimes differ from those observed in humans with mutations in the corresponding genes, and the boundaries between subclasses of glaucoma produced in mice have sometimes been blurred.
3.8 Primary Open-Angle Glaucoma Models
Several mouse models of glaucoma have been developed using modifications of genes where mutations are known to cause POAG in humans. MYOCILIN (MYOC) was the first gene in which mutations were identified that lead to human POAG (Stone et al. 1997) . The MYOC gene encodes a secreted glycoprotein. Mutant myocilin proteins associated with severe glaucoma in humans are not secreted from cultured cells or from TM into the aqueous humor (Jacobson et al. 2001, Gobeil et al. 2004) . Instead, these mutant myocilin proteins accumulate in the endoplasmic reticulum (ER), which leads to deleterious effects and cell death (Joe et al. 2003; Liu and Vollrath 2004) . Expression of mutant myocilin also impairs mitochondrial function (He et al. 2009) and makes cells more sensitive to oxidative stress (Joe and Tomarev 2010), which is intimately connected to ER stress. It is not surprising that a number of labs have attempted to express mutant myocilin using different approaches.
Neither null mutations in the Myoc gene (Kim et al. 2001) nor overexpression of the WT myocilin protein in eye drainage structures (Gould et al. 2004a) leads to a glaucoma phenotype in mice, although defects in myelination in the optic nerve of Myoc null mice have been reported (Kwon et al. 2014) .
The mutant form of human myocilin Y437H expression which triggers severe juvenile-onset POAG in humans (Alward et al. 1998) and the analogous mouse myocilin mutant, Y423H, have been used to generate rodent glaucoma models with variable effects noted. Transgenic mice have been produced using bacterial artificial chromosomes containing mutated human or mouse myocilin genes and long flanking sequences (Senatorov et al. 2006; Zhou et al. 2008) . The observed pathological changes using this system were quite modest and similar for both human and mouse mutant myocilin . A moderate elevation in IOP (about 2 mmHg at day time and about 3–4 mmHg at night time above WT littermates) was detected only in mice that were older than 12 months but not in younger animals. A 20 % reduction in the number of peripheral but not central RGCs and degeneration of axon fibers mainly in the peripheral areas of the optic nerve were observed only in old transgenic mice (Senatorov et al. 2006; Zhou et al. 2008) . Interestingly, RGC electrical responsiveness as judged by pattern electroretinogram was reduced before any reduction in the number of RGCs was detected and was more pronounced in aged versus young mice (Chou et al. 2014) . In contrast to the above observations, replacement of the endogenous mouse Myoc allele with a mutant Y423H allele did not lead to a detectable elevation of IOP or degenerative changes in the retina or optic nerve (Gould et al. 2006) . This might be explained by different genetic backgrounds of mice (McDowell et al. 2012) and/or different levels of mutant myocilin produced. Indeed, expression of high levels of mutated human Y437H myocilin in the eye drainage structures and sclera under the control of the CMV promoter in transgenic mice led to elevation of IOP that was detectable as early as 3 months of age (about 3 and 6 mmHg at day and night time, respectively) (Zode et al. 2011) . This was accompanied by the loss of RGCs (about 18 % by 3–5 months of age and 30 % by 12–14 months of age) and optic nerve axons. Functional deficits in RGCs were observed as early as 3–5 months of age. Since the expression of mutant (and WT) myocilin was not detected in the retina, pathological changes in the retina of transgenic mice were not attributed to the retinal expression of the transgene. Expression of mutant human myocilin induced ER stress in the drainage structures (Joe and Tomarev 2010; Zode et al. 2011) and induced apoptosis associated with TM loss, while physiological levels of mutant mouse myocilin did not induce ER stress (Gould et al. 2006) . Addition of 20 mM of a chemical chaperone, phenylbutyric acid, to the drinking water reduced ER stress and prevented the glaucoma phenotype in transgenic mice expressing mutant human myocilin under the CMV promoter. Phenylbutyric acid decreased intracellular accumulation of myocilin in ER and promoted the secretion of mutated myocilin in the aqueous humor, thus preventing TM cell death (Zode et al. 2011) . Phenylbutyric acid has been approved by FDA for the treatment of a number of diseases, and data obtained with a myocilin-induced mouse model of POAG as well as with a glucocorticoid-induced mouse model of POAG provide the basis for evaluating the use of chemical chaperones in the treatment of myocilin- and glucocorticoid-induced glaucoma in humans (Zode et al. 2011, 2014) .
A genetic mouse model based on the synergistic interaction of mutated myocilin and another significant risk factor, oxidative stress, has been recently described. Double-mutant mice were produced bearing human MYOC with a Y437H point mutation as well as a heterozygous deletion of the gene for the primary antioxidant enzyme, superoxide dismutase 2 (SOD2). These mice demonstrated more dramatic changes in the retina , optic nerve, and TM compared with mice carrying the MYOC mutation alone. Elevation of IOP (about 4 mmHg over WT littermates at day time) was also detected in 10- to 12-month-old double mutant mice, whereas no elevation of IOP was detected in single MYOC mutant or SOD2 deficient lines. Based on this interaction, it was suggested that patients with myocilin-induced glaucoma should avoid habits such as smoking that cause free radical production and/or utilize antioxidant supplements to delay the progression of the disease (Joe et al. 2015) .
Another transgenic mouse model of POAG involves expression of connective tissue growth factor (CTGF) (Junglas et al. 2012) . Although there are no reports describing mutations in CTGF in human glaucoma, it is expressed in abundance in the human TM (Tomarev et al. 2003) , and CTGF levels are increased in the aqueous humor of patients with pseudoexfoliation glaucoma (Browne et al. 2011) . Moreover, CTGF is modified by the TGF-β2 pathway and activation of TGF-β2 in the aqueous humor of patients with POAG has been demonstrated (Tripathi et al. 1994; Inatani et al. 2001) . Expression of CTGF under the control of the βB1-crystallin promoter led to elevation of IOP in the eyes of 2- to 3-month-old mice from about 17 mmHg in control mice to 21–22 mmHg in transgenic mice. Light microscopy was unable to identify structural differences in the ciliary body, iris, TM, and Schlemm’s canal of 1- to 3-month-old transgenic mice when compared with their WT littermates, and the chamber angle was wide open (Junglas et al. 2012) . However, a 150- to 200-nm broad area underneath the cell membrane was observed in TM cells of transgenic mice. This area contained bundles of 6- to 7-nm microfilaments corresponding to the diameter of actin filaments. Such bundles were more rarely observed and were considerably thinner in WT littermates (Junglas et al. 2012) . Thus, the effects of CTGF on IOP are probably caused by a modification of the TM actin cytoskeleton. Increased IOP in these mice was accompanied by degenerative changes in the optic nerve. A 26 % reduction in the number of optic nerve axons was detected in 3-month-old βB1-CTGF transgenic mice compared with their WT littermates (Junglas et al. 2012) . CTGF-overexpressing mice represent a useful model that mimics the essential functional and structural aspects of POAG (Junglas et al. 2012).
Mice lacking the α1-subunit of the nitric oxide receptor-soluble guanylate cyclase (sGCα1 −/−) represent another model of POAG, as they have been shown to undergo thinning of the retinal nerve fiber layer and loss of optic nerve axons without detectable changes in the morphology of the iridocorneal angle (Buys et al. 2013) . Nitric oxide-cGMP signaling has been suggested to be involved in the regulation of aqueous humor outflow and IOP (Kotikoski et al. 2003) . In the sGCα1 −/− line, aqueous humor outflow rate was reduced in 57-week-old mice, and a statistically significant elevation of IOP was detected starting from 39 weeks of age, while at 73 weeks of age IOP was about 4 mmHg higher than in age-matched WT mice. Retinal arterial dysfunction has also been detected in sGCα1 −/− mice compared with WT mice (Buys et al. 2013) . The sGCα1 −/− line might be a useful tool to study involvement of nitric oxide-cGMP signaling in POAG.
As mentioned above, the Aca23 mutation in collagen 8A2 leads to greater globe enlargement in response to ocular hypertension but not to neurodegeneration (Steinhart et al. 2012) . In contrast, expression of the mutated α1-subunit of collagen type I (Col1a1) in transgenic mice leads to not only IOP elevation, but also optic nerve damage (Mabuchi et al. 2004) . The mean IOP of the transgenic Col1a1 mutant mice was significantly elevated compared with that of controls at 16–54 weeks by 20–40 %. It has been demonstrated that IOP and outflow facility in these transgenic mice were inversely correlated over the 12- to 56-week study period (Dai et al. 2009) . The mean axonal loss between 24 and 54 weeks of age in the transgenic Col1a1 mice was about 28 % (Mabuchi et al. 2004). Col1a1 mutant mice may serve as a useful model of POAG and for evaluating the relationship between collagen type 1 metabolism and aqueous outflow (Dai et al. 2009) .
Several mouse models of NTG have been developed. Two NTG models are based on the expression of mutated optineurin (Optn) or WD repeat-containing protein 36. Mutations in the OPTINEURIN (OPTN) (Rezaie et al. 2002) and WDR36 (Monemi et al. 2005) genes were originally identified as being associated with POAG. Subsequent publications suggested that WDT36 may act as a modifier gene for POAG (Hauser et al. 2006a) . The OPTN gene has also been associated with a number of different diseases including amyotrophic lateral sclerosis and Paget’s disease of bone (Albagha et al. 2011) . Optn plays a role in the regulation of cell division, membrane trafficking, protein secretion, and host defense against pathogens (Kachaner et al. 2012) . The E50K mutation on Optn has been associated with more severe forms of NTG (Aung et al. 2005; Hauser et al. 2006b) . Transgenic mouse lines have been created that express the E50K Optn mutant under the control of chicken beta-actin promoter and CMV enhancer (Chi et al. 2010a) . These mice did not show statistically significant elevation of IOP even in 16-month-old animals, but did demonstrate loss of RGCs and connecting synapses in the peripheral retina . Expression of the E50K Optn mutant led to the appearance of apoptotic cells and degeneration of the entire retina, leading to approximately a 28 % reduction in transgenic retinal thickness at 16 months of age (Chi et al. 2010a) . Transgenic mice also demonstrated reactive gliosis and E50K mutant protein deposits in the outer plexiform layer (Minegishi et al. 2013) . The E50K mutation disrupts the interaction between Optn and Rab8 GTPase, a protein involved in the regulation of vesicle transport from the Golgi to the plasma membrane, and it was suggested that disruption of the Optn-Rab8 complex may affect the trafficking in the RGCs as well in the photoreceptors (Chi et al. 2010a) . The E50K mutant also demonstrated enhanced interaction with TANK-binding kinase 1 (TBK1) (Minegishi et al. 2013) . Mutations in TBK1 or variations in its copy number have been associated with NTG (Fingert et al. 2011; Seo et al. 2013) . The cross talk of Optn and TBK1 is likely to play a significant role not only in glaucoma but also in other retinal diseases, and it was suggested that compounds reducing or abolishing the interaction between the E50K mutant and TBK1 are likely to be beneficial in the treatment of NTG patients (Minegishi et al. 2013) .
WD repeat-containing protein 36 is involved in the maturation of 18S rRNA. Expression of mutant Wdr36 in transgenic mice under the control of the chicken beta-actin promoter and CMV enhancer led to a 25 % reduction in the thickness of the peripheral retina and loss of RGCs of 16-month-old mice compared with WT mice without statistically significant changes in IOP (Chi et al. 2010b) . At the same time, mice carrying only a single copy of the Wdr36 gene did not develop glaucoma and did not show any detectable eye pathology (Gallenberger et al. 2014) .
Mice deficient in the glutamate transporters Glast or Eaac1 have also been considered as mouse models of NTG (Harada et al. 2007) . These mice demonstrate spontaneous RGC and optic nerve degeneration without elevated IOP. The number of RGCs was reduced by about 50 % in 8-month-old Glast null mice compared with their WT littermates. A 20 % reduction in the number of cells in the inner nuclear layer was also observed in Glast null mice of this age. In Glast-deficient mice, the glutathione level in Müller glia was decreased and the administration of glutamate receptor blocker or overexpression of dedicator of cytokinesis 3 (Dock3) (Namekata et al. 2013) prevented RGC loss. In EAAC1-deficient mice, RGCs were more vulnerable to oxidative stress. Data obtained with these models suggest that glutamate transporters are necessary both for prevention of excitotoxic retinal damage and for synthesis of glutathione, a major cellular antioxidant (Harada et al. 2007). Since Dock3 directly binds to the intracellular C-terminus domain of an N-methyl-d-aspartate receptor subunit NR2B, it was also proposed that Dock3 overexpression prevents glaucomatous retinal degeneration by suppressing NR2B-mediated glutamate neurotoxicity and oxidative stress (Namekata et al. 2013) . In general, these described models of NTG might be used to investigate the mechanisms of RGC death independent of IOP elevation as well as to develop possible therapies that could augment IOP reduction.
It has been proposed that endothelin 1 (EDN1), a potent vasocontractor, may contribute to glaucoma pathophysiology (Prasanna et al. 2011) . Overexpression of End1 under the control of the receptor tyrosine kinase tie-1 promoter in transgenic mice led to a progressive loss of RGCs and a decreased thickness of the inner nuclear layer and outer nuclear layer as early as around 10–12 months without elevation of IOP (Mi et al. 2012) . Degeneration of optic nerve axons, together with blood vessel changes and retinal gliosis, was also observed in 24-month-old mice (Mi et al. 2012) . This model might be useful to study the contribution of endothelial EDN1-related mechanisms to glaucoma.
3.9 Primary Angle-Closure Glaucoma Models
Unlike POAG in which IOP elevation occurs in the presence of structurally unimpeded aqueous outflow pathways, PACG is associated with physical loss of access to the drainage angle structures. The incidence of PACG is higher in Asians than in Europeans and Africans, and PACG causes blindness more frequently than POAG (Quigley and Broman 2006; Tham et al. 2014) . Several mouse models of PACG have been developed.
The genes for VAV2 and VAV3, guanine nucleotide exchange factors for Rho guanosine triphosphatases, have been reported to cause POAG in the Japanese (Fujikawa et al. 2010) . Although the involvement of VAV2 and VAV3 variants in POAG, NTG, and PACG has not been confirmed by other studies (Rao et al. 2010; Shi et al. 2013) , analysis of Vav2/Vav3 null mice, and to a lesser degree Vav2 null mice, showed that these mice develop eye pathologies that resemble glaucoma in humans (Fujikawa et al. 2010) . Eyes of Vav2/Vav3 null mice develop buphthalmos and frequently demonstrate angle closure starting between 6 and 12 weeks of age. Six-week-old Vav2/Vav3 null mice showed elevated IOP (18.2 ± 3.1 vs. 14.0 ± 2.4 mmHg), which increased further by 10 weeks of age (22.5 ± 7.4 vs. 14.6 ± 4.2 mmHg)(Fujikawa et al. 2010) . IOP in Vav2/Vav3 null mice could be reduced by a number of drugs used for IOP reduction in humans. Although quantitative estimates were not presented, Vav2/Vav3 null mice demonstrated RGC loss and ONH cupping at 10 weeks of age which was more pronounced in aged mice (Fujikawa et al. 2010) . The early onset of IOP elevation and high frequency of ocular pathologies makes this model rather attractive for neuroprotective studies as well as for the investigation of molecular mechanisms involved in pathological structural changes.
Another mouse mutant line that mimics PACG, glaucoma relevant mutant 4 or Grm4, was identified in an N-ethyl-N-nitrosourea mutagenesis screen designed to discover new genes that may affect IOP (Nair et al. 2011) . Grm4 mice carry a mutation in the Prss56 gene encoding serine protease. The identified mutation leads to a truncation of the C-terminal part of the protein, which does not affect protease activity with the synthetic substrate. The homozygous Prss56 mutants show elevated IOP and the incidence of high IOP (more than 19 vs. 15 mmHg in WT) increased with age (~ 50 % of mice at 3 months and ~ 90 % of mice at 12 months of age) (Nair et al. 2011) . The eye angle was narrow and the iris was in close proximity to the TM in mutant mice. In addition, Grm4 mice had a shorter axial length than control mice (Nair et al. 2011) . Mutations on the PRSS56 gene have also been shown to segregate with disease in human families with posterior microphthalmia (Gal et al. 2011; Nair et al. 2011) . Grm4 mice therefore may serve as a model to study a spectrum of ocular phenotypes, including changes in the size of the eye, hyperopia, and PACG (Nair et al. 2011) .
3.10 Pigmentary Dispersion and Exfoliation Glaucoma Models
Certainly, the most widely used and best characterized model of naturally occurring glaucoma is the DBA/2J mouse (Libby et al. 2005a) , which bears recessive mutations in the Gpnmb and Tyrp1 genes, encoding tyrosinase-related and glycosylated transmembrane proteins, respectively. These mutations lead to pigment dispersion, iris transillumination, iris atrophy, and anterior synechia (Anderson et al. 2002) . The subsequent blockade of aqueous outflow results in ocular hypertension by the age of 9 months, which is accompanied by the typical signs of glaucoma including death of the RGCs, optic nerve atrophy, ONH cupping, and visual deficits (Libby et al. 2005a) . Interestingly, DBA/2J mice are more resistant to the RGC death after optic nerve crush than 14 other inbred lines of mice tested. It was suggested that two dominant loci are linked to the resistance phenotype (Li et al. 2007) .
Several important observations have been made using the DBA/2J model. It has been shown that degeneration of RGCs does not occur in the uniform manner across the retina. Regions of cell death and survival radiating from the ONH in fan-shaped sectors have been detected (Jakobs et al. 2005; Howell et al. 2007) . Early signs of axon damage are localized to an astrocyte-rich glial lamina region of the optic nerve just posterior to the retina (Howell et al. 2007) . It has been suggested that axon damage at the ONH might be a primary lesion in this model, similar to the pathophysiology of human glaucoma (Jakobs et al. 2005) . The proapoptotic protein BAX was shown to be necessary for RGC death but not for RGC axon degeneration (Libby et al. 2005b) . At the same time, deficiency in Bim, proapoptotic Bcl-2 family member that induces BAX activation, did not prevent RGC death in DBA/2J eyes with severe optic nerve damage (Harder et al. 2012) . It has, therefore, been proposed that BAX may be a candidate human glaucoma susceptibility gene and that characterization of BAX alleles may be used as a predictive value for glaucoma progression (Libby et al. 2005b) .
Similar to the results obtained with rat and monkey models (Ahmed et al. 2004; Stasi et al. 2006; Guo et al. 2010) , genes involved in glial activation and immune response are activated in DBA/2J retina (Steele et al. 2006; Panagis et al. 2010, 2011) . The complement component C1q, the initiating protein of the classical complement cascade, is upregulated in the retina in several animal models of glaucoma and human glaucoma (Stasi et al. 2006) . In DBA/2J mice, C1q, and downstream C3 relocalize to adult retinal synapses at an early stage of glaucoma prior to visible signs of neurodegeneration . C1q in the adult glaucomatous retina may mark synapses for elimination at early stages of the disease, suggesting that the complement cascade mediates synapse loss in glaucoma similar to synapse elimination during development (Stevens et al. 2007; Rosen and Stevens, 2010) . It has been shown that upregulation of the complement cascade and the endothelin system (Edn2) occurs at early stages of glaucoma before detectable glaucomatous changes (Howell et al. 2011) . Disturbing any of these pathways separately through a mutation in C1qa or through inhibition of the endothelin system with bosentan, an inhibitor of endothelin receptor type A, was protective against retinal and optic nerve damage in DBA/2J mice (Howell et al. 2011) . Combinatorial targeting of the endothelin system with bosentan and the complement system with a mutant in C1qa was more effective in protecting the optic nerve than separately inhibiting either process (Howell et al. 2014) . It is interesting to note that DBA/2J mice are naturally deficient in complement component 5, and backcrossing of a functional C5 gene into DBA/2J leads to a more severe glaucoma at an earlier age than in regular DBA/2J mice (Howell et al. 2013) . Novel therapeutic strategies for glaucoma have also been suggested on the basis of the results obtained with DBA/2J mice. It has been demonstrated that high-dose γ-irradiation together with bone marrow transfer protected RGCs in DBA/2J mice (Anderson et al. 2005) . It has been later shown by the same group that the entry of proinflammatory monocytes into the DBA/2J optic nerve occurs very early prior to neural damage and a one-time treatment of an individual eye in young mice with X-rays protects a treated eye from glaucoma even without bone marrow transfer. The X-ray treatment modifies activation of endothelial cell signaling and abolishes migration of monocytes into the eye (Howell et al. 2012) . At the same time, exposure of rats to head-only X-ray irradiation provided no protection from optic nerve degeneration induced in rats by an episcleral vein injection of hypertonic saline (Johnson et al. 2014) . The elucidation of the biological basis of differences in response to radiation in two different models of glaucoma, as well as in other glaucoma models, may be essential for a better understanding of glaucomatous nerve injury in human glaucoma and for improvements of glaucoma treatment (Johnson et al. 2014) .
< div class='tao-gold-member'>
Only gold members can continue reading. Log In or Register a > to continue
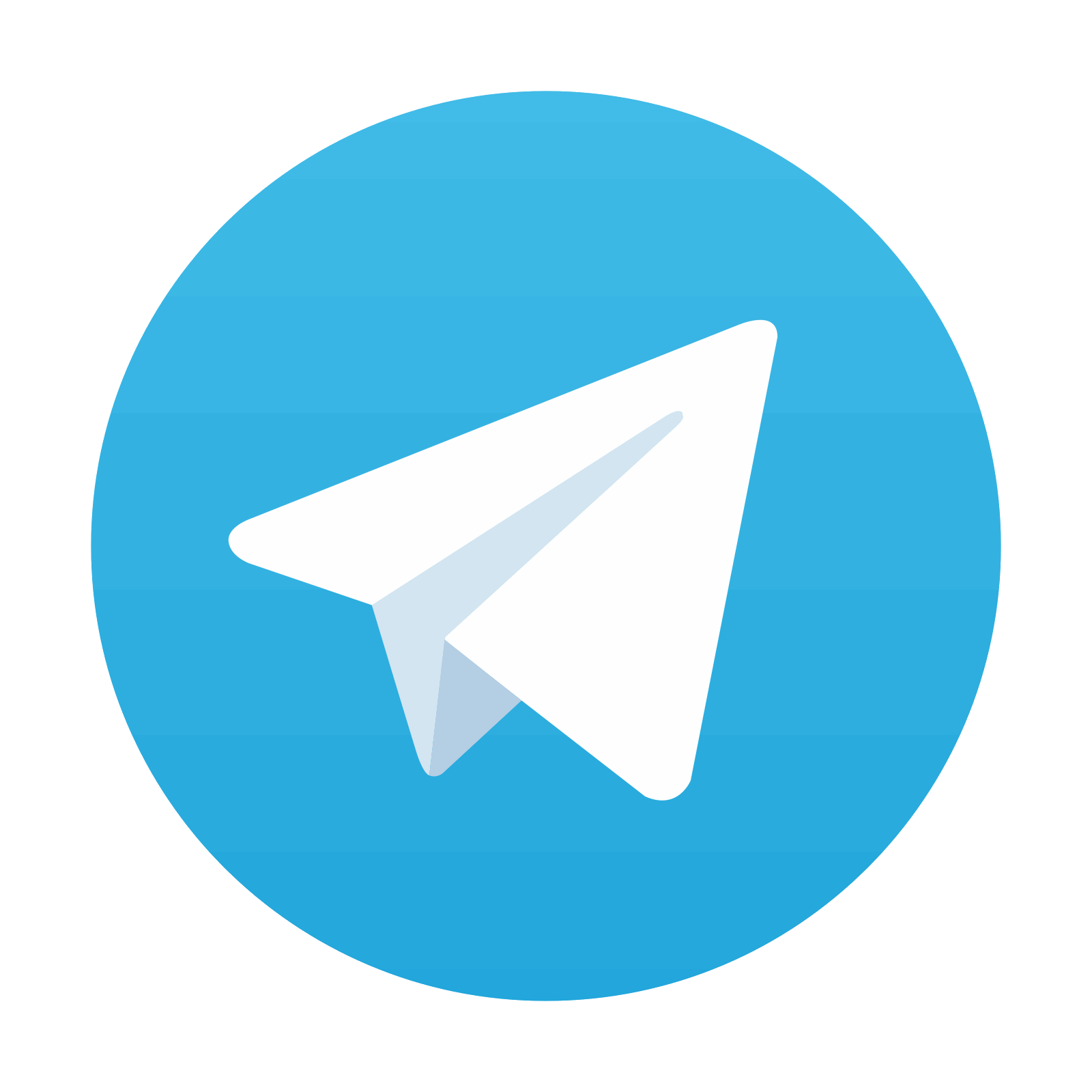
Stay updated, free articles. Join our Telegram channel

Full access? Get Clinical Tree
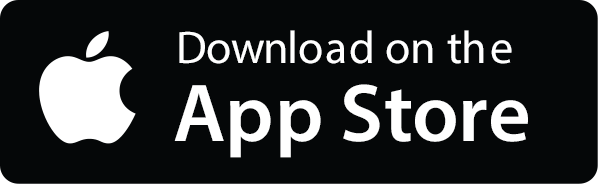
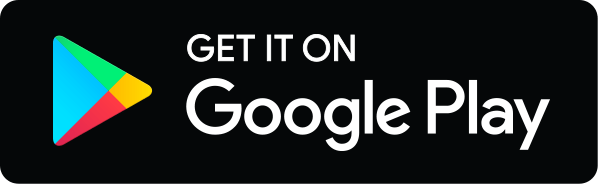