Fig. 5.1
(a) Fundus photograph of the right eye of a patient with NPDR and central involvement clinically significant macular oedema. Marked exudation is observed around the fovealcentre. (b) Trypsin digest retinal specimen of a 65-year-old type 2 diabetic patient with NPDR stained with PAS-haematoxylin. A centrally placed radial artery is flanked above and below by retinal veins and the intervening capillary beds. Numerous microaneurysms stain strongly with PAS and show a predominantly peri-arterial distribution (arrows)
If left untreated, NPDR can develop to PDR which is the presence of new blood vessels or neovascular membranes in the pre-retinal space. PDR typically occurs in approximately 25 % of patients with T1D and in about 15 % of patients with T2D with > 25 years disease duration (Klein et al. 1984) . Progressive retinal microvascular damage leads to severe ischaemia and the release of vasoproliferative factors from retinal cells, which subsequently stimulate new blood vessels to grow in the retina in order to improve oxygenation. PDR is closely associated with retinal ischaemia and neovascularisation is typically observed in areas adjacent to extensive vascular non-perfusion. These new vessels are fragile causing haemorrhages in the retina and vitreous. In addition, fibrovascular membranes may form around the neovascular site and their contraction may cause retinal tears and tractional detachment, resulting in severe vision loss .
When diabetes impacts the macula, it is termed diabetic maculopathy. In this area of central vision, excessive vasopermeability and excess fluid in the retina is called diabetic macular oedema (DMO). DMO typically occurs during the late stages of disease and is the most frequent cause of blindness in diabetic patients (Joussen et al. 2007) . The leakage of plasma proteins from the damaged BRB is the primary cause of DMO, which results in the accumulation of fluids within the retina, in particular within and/or around the macular area. DMO can readily be detected by optical coherence tomography (OCT), and is characterised by increased retinal thickness and/or the presence of fluid-filled cysts in the macular region (Fig. 5.2a and 5.2b). DMO is more common than PDR, although, both endpoints can occur together.
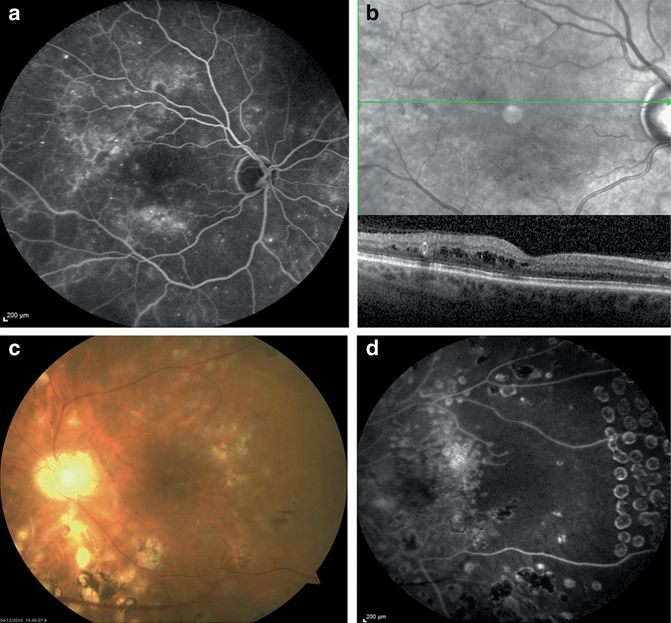
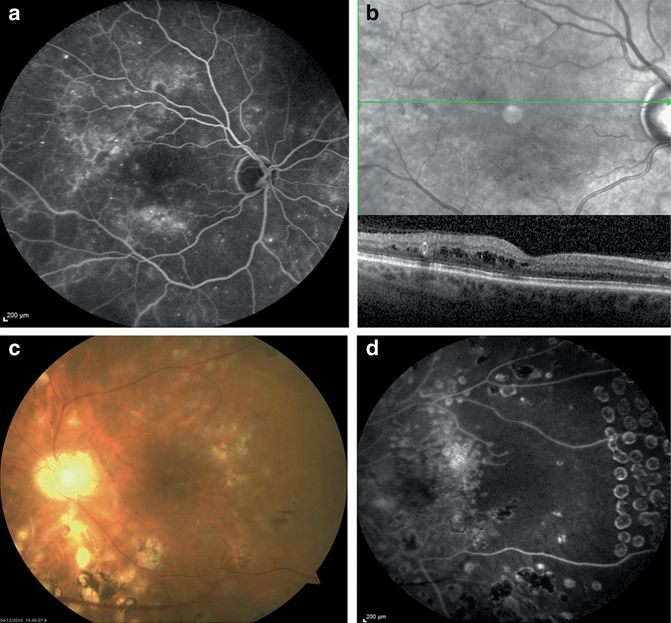
Fig. 5.2
Fundus fluorescein angiography (FFA) (a) and horizontal optical coherence tomography (OCT) (b), with the corresponding fundus image demonstrating the location from which the OCT section had been taken, obtained from the RE of a patient with diabetic macular oedema. On FFA, two areas of hyperfluorescence, located supero-temporally and inferior to the fovea, were detected. On OCT, intraretinal oedema extending to fixation was evident. Colour fundus photograph (c) and fundus fluorescein angiography (FFA) (d) of the left eye of a patient with proliferative diabetic retinopathy treated with extensive panretinal photocoagulation. Despite the latter treatment recurrent vitreous haemorrhages occurred. Fluorescein angiography was undertaken once the last vitreous bleeding had cleared and disclosed an area of retinal ischaemia which had been left untreated temporal to the fovea. This area seemed to support the persistence of a frond of active neovessels located in the nasal retina, whose presence was also demonstrated by FFA (not shown on the images)
While loss of retinal perfusion is a hallmark of DR, neural function is also compromised during this disease, and it has been suggested that this could occur prior to overt vessel pathology (reviewed by Antonetti et al. (2012)) . Electrophysiological studies of patients with diabetes display loss of colour vision (Roy et al. 1986) and contrast sensitivity (Sokol et al. 1985) and they often show abnormalities in the electroretinogram (ERG; Tzekov and Arden 1999) . DR may therefore be more accurately conceived as a disease of the neuro-vascular unit, resulting in dysfunction and eventual death of several vascular cells, neurons and glia (Antonetti et al. 2012) .
Prevention of DR in patients is reliant on metabolic control relating to maintenance of tight glycaemic control, blood pressure control and correction of dyslipidaemia (Stitt et al. 2013) . When patients progress to the late stages of DR, there are several treatments such as photocoagulation, intravitreal delivery of vascular endothelial growth factor (VEGF) blocking agents and intravitreal corticosteroids, all of which have proven value in reducing DMO. Laser photocoagulation and vitreoretinal surgery are the main treatments for PDR. Figure 5.2c and 5.2d shows a typical colour fundus photograph (Fig. 5.2c) and a fundus fluorescein angiography (Fig. 5.2d) of the eye with PDR treated with panretinal photocoagulation. Unfortunately, current approaches to DMO and PDR are, by definition, at the late stage and carry significant side effects. Furthermore, they fail to address the underlying cause of ischaemia and/or inflammatory pathology which drives these endpoints. It is clear that there are deficits in the current standards of care for DR and that there is a need for improved therapies, especially for the ones that show efficacy at the early stages of disease. The use of animals that model key aspects of the structural, functional and metabolic features of DR plays a critical role in understanding the disease and development of new therapeutic approaches .
5.2 The Need for Animal Models to Understand the Pathophysiology of DR
Retina-specific and systemic molecular defects that occur during diabetes make DR pathogenesis multifactorial and highly complex and many interacting pathogenic pathways have been proposed to play causative roles, as reviewed by Antonetti et al. (2012) and Brownlee M (2001) . This complexity and the decades-long progression of DR present a serious challenge for developing animal models of the clinical disease. For example, the various animal species used have differing retinal anatomy, metabolism, diets, lifespans, etc. and while they may be able to reproduce some aspects of human diabetes, no single model currently exists that faithfully reproduces all aspects of human DR. In particular, it is important to note that many key lesions that are regarded as typical for background DR in patients, including microaneurysms, exudates, haemorrhages and cotton wool spots seldom, if ever, occur in short-term diabetic rodent models and only appear in dog, pig or monkey models with longer durations of diabetes (Lai and Lo 2013) . Moreover, there are no mainstream T1D or T2D models that reproduce DMO or PDR endpoints. Therefore the value of various animal models currently available needs to be carefully evaluated to avoid injudicious extrapolation to clinical scenarios. In this section, we will discuss the merits and shortcomings of common animal models .
5.3 Rodent Models of DR
5.3.1 Chemically Induced Diabetes in Mice and Rats
The capacity for breeding and housing large numbers of animals in a relatively small facility has made rats and mice the most common model for DR research. While they bring cost, uniformity and transgenic technology advantages, rodents have obvious disadvantages such as metabolism, diet and lifestyle that are very different from humans. Mice and rats species used in laboratories evolved as nocturnal animals and, as such, their eyes are adapted for night vision with a large lens and a rod-dominant retina, with few cones and no macula. However, mice and rats do have an intraretinal vasculature and a neuroglial structural anatomy that is similar to the humans and this has made rodent models of DR the predominant species for research.
Streptozotocin (STZ) or alloxan are often used as chemicals to destroy pancreatic β-cells and thereby induce T1D in rodents. STZ is used most commonly to induce diabetes in rats of various strains and they have been the mainstay for many of the seminal studies demonstrating retinal lesions after diabetes durations of 1–12 months (Robinson et al. 2012) . In both mice and rats, diabetes leads to dysfunction or failure (or partial failure) of various organs, including the retina, kidneys, nerves and heart. Depending on the strain used and the duration of hyperglycaemia, these diabetic models reproduce some early lesions of DR, including thickening of the vascular BM, increased vascular permeability, loss of retinal pericytes and capillary closure (Robinson et al. 2012) . Nonvascular changes, including biochemical changes, neuronal and glial changes are often detected and might contribute to the vascular pathology observed in these models. Figure 5.3 shows some representative DR features observed in STZ-induced rodent models.
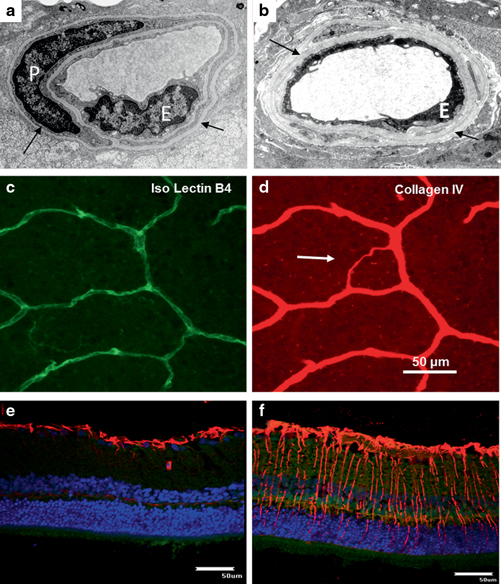
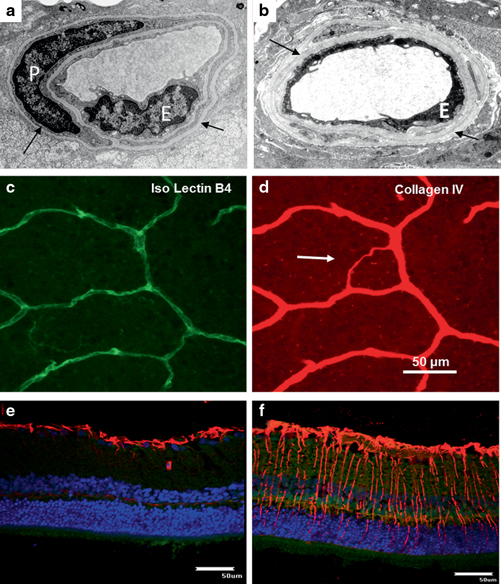
Fig. 5.3
Transmission electron microscopy (TEM) shows the thickened basement membrane (arrows) and loss of viable endothelial cell (E) in a diabetic rat (b). A typical normal retinal capillary (a) showing endothelial cell (E) and pericyte (P) with the cohesive basement membrane (arrows). Acellular capillary can be detected via the immunofluorescent staining technique. Acellular capillary ( arrow in d) loses endothelial cells (Isolectin B4, c) but persists with the basement membrane (Collagen IV, d). Müller cell activation can be detected in diabetic rodents. Expression of GFAP is limited at the end feet of Müller cells in the normal retina (e); however, GFAP can be detected across the whole retina in a diabetic rodent (f). Red: GFAP; blue: DAPI
Rodent models of DR have permitted the precise regulation of elements in the diabetic milieu, intervention with experimental drugs that modulate gene regulation and detailed cellular and molecular analysis of the retina. For example, studies in animal models of DR have strengthened our appreciation of pro-inflammatory pathways and their connectivity to lesions such as BRB breakdown . Using detailed experimental approaches in animals, especially rodents, it has been established that indicators of inflammation, including leukostasis, increased expression of adhesion molecules in retinal vascular endothelial cells and leukocytes, altered vascular permeability, and increased production of prostaglandins, nitric oxide, cytokines and other inflammatory mediators occurs in the retina after diabetes durations of 1–6 months (Tang and Kern 2011) . Microglia are the resident macrophage in the retina and they play an important role in maintaining homeostasis in the neuropile. These cells become activated in diabetes and contribute to the pro-inflammatory state. Microglia become altered in DR, with the cell morphology changing from a resting, surveillance state with long thin dendrites emanating from a small cell body (Fig. 5.4a and 5.4b) to an activated, amoeboid state with thick dendrites and an enlarged cell body (Fig. 5.4c and 5.4d) .
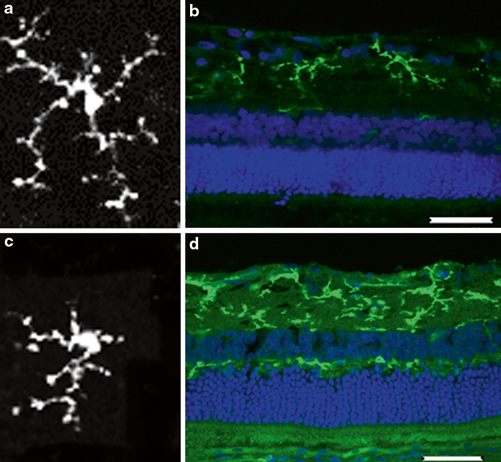
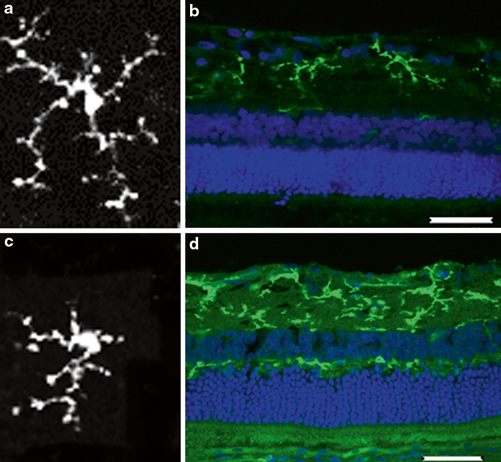
Fig. 5.4
Microglial activation in a diabetic retina. Microglia morphology changes from a resting state with long dendrites and small cell body (a) to an activated, amoeboid state with shorter dendrites (c). Increased immuno-reactivity is also observed in retinal cross-section of a diabetic rat (d) compared with a normal retina (b). Green: microglia; blue: diamidino-2-phenylindole (DAPI)
It is important to note that the retinal biochemistry and histopathological features differ between rat and mice and even between different strains of the same species. For example, pigmented Brown Norway rats develop sustained vascular leakage during the experimental period of 16 weeks of diabetes, whereas the albino Sprague–Dawley rats typically show retinal hyperpermeability from day 3 to day 10 after the onset of diabetes (Zhang et al. 2005) . Likewise, Lewis rats seem to demonstrate the most accelerated loss of retinal capillaries after 8 months diabetes as compared to Sprague–Dawley rats that show fewer lesions at the same time points (Kern et al. 2010) .
In recent years, mice have been commonly used in preference to rats, since they have reduced costs and provide greater availability of molecular tools and offer scope from transgenic technology. Although transgenic technology is not confined to mice (and transgenic diabetic rats have been used to great effect (Berner et al. 2012)), these murine models have provided a significant value in dissection of molecular mechanisms. The structural and functional lesions in diabetic mice are summarised in Table 5.1. In comparison to rats, mice are comparatively resistant to diabetes induction using STZ and considerably higher doses of STZ are required. While some studies have used a single high dose of STZ in mice (Cox et al. 2003) , this may produce less desired, non-beta cell effects. Commonly, five injections of low-dose STZ given over successive days has been the standard protocol for induction of diabetes in mice (McVicar et al. 2011) .
Table 5.1
The structural and functional lesions in diabetic mice
Structural lesions | Functional lesions |
---|---|
Vasculopathy | |
Capillary basement membrane thickening | Leukostasis |
Capillary degeneration, endothelial cell death and pericyte drop-off | BRB dysregulation of inner retina |
Neuropathy | |
Retinal degeneration | Abnormal retinal electrophysiology (reduced ERG a-, b-wave amplitude, prolonged oscillatory potentials implicit time |
Neuronal cell damage, loss of synaptic connectivity | |
Ganglion cell apoptosis |
5.3.2 Spontaneous Diabetic Rodents as Models of DR
Mice and rats can develop diabetes spontaneously either due to mutations in certain insulin-related genes or due to a susceptibility to obesity and insulin resistance. These animals can provide the advantage that their T1D or T2D diabetes is naturally occurring and is not induced by chemicals that could have possible non-diabetes-related effects in several organs, especially when high doses are used. The disadvantages of these models often relate to their increased cost because they are not widely bred and, in some cases, diabetes only occurs in homozygous or in a single sex which results in the need to breed much higher numbers of animals. Rat strains that develop spontaneous T1D or T2D include Zucker diabetic fatty rats, WBN/Kob rats, Otsula Long-Evans Tokushima fatty rats, Goto-Kakizaki (GK) rats and diabetic Torii rats. A number of mouse strains also develop diabetes spontaneously; these include nonobese diabetic mice (NOD), db/db mice and OB/OB mice which develop T1D and T2D, respectively. Retinal biochemistry and histopathological features such as vascular lesions (Abari et al. 2013) and neural retina thinning (Bogdanov et al. 2014) have been reported in these DR models .
Of particular note, the Ins2Akita mouse is a model of spontaneous T1D, which has been shown to develop a range of vascular, neural and glial lesions that are comparable to what has been shown in STZ diabetic mice (Barber et al. 2005; Gastinger et al. 2006) . Although one study reported no retinal thinning or vascular changes in Ins2Akita mice up to 6 months of age (McLenachan et al. 2013) , a more recent study has shown a progressive thinning of the retina from 3 months onwards and the loss of synaptic connectivity, particularly in second-order neurons in these mice (Hombrebueno et al. 2014) . The Ins2Akita mice is widely used in experimental studies of diabetes complications, including DR .
5.3.3 Diet-Induced DR in Rodents
An enriched diet can also induce diabetes. For example, high-fat fed mice develop early T2D, with impaired glucose intolerance and insulin resistance (Winzell and Ahren 2004) . Rodents fed with a galactose-rich diet for a prolonged period of time develop a retinopathy that resembles the early stages of DR, including capillary dropout and pericyte loss (Kern and Engerman 1994) .The galactose-fed model was initially used for investigating the role of polyol pathway in the pathogenesis of DR (Robison et al. 1983) . Studies suggested that aldose reductase inhibitors (ARIs) reduced the incidence and severity of diabetic retinal lesions occurring in these galactose-fed animals (Robison et al. 1983).
An interesting diet-induced rodent model of DR has been reported in the sand rat ( Psammomysobesus). These animals are active in daytime and have cone-rich retinas unlike nocturnal laboratory rats and mouse strains. When sand rats are maintained in captivity for 4–7 months and fed standard chow, ~60 % develop T2D-like symptoms and show facets of DR such as BRB dysfunction, loss of retinal neurons and capillary degeneration (Saidi et al. 2011) . There is also a suggestion that these animals may show some degree of pre-retinal neovascularisation (Saidi et al. 2011), although, how frequently this occurs and the underpinning pathology remains ill-defined .
5.4 Dog Models of DR
Next to rodents, dogs have been used most extensively as models of DR and although there are obvious ethical constraints and significant cost issues, this species is an excellent model of the early-stage clinical disease. Most studies have used chemical induction of diabetes (Anderson et al. 1993) or feeding with galactose (Kador et al. 1994) , although the same caveats exist when comparing hyperglycaemia with galactosaemia (Engerman et al. 1990) . The main advantage of dogs over mice and rats is the duration of diabetes that can be achieved due to longer lifespan of this species and, typically, 5 years hyperglycaemia or galactosaemia produces lesions that are much closer to clinical NPDR than what is possible with rodent models (Howell et al. 2013) . With daily insulin delivery, the degree of hyperglycaemia in diabetic dogs can be maintained to much lower levels, which provides a more clinically relevant disease state than can be achieved with insulin-treated diabetic rodents (Anderson et al. 1993; Gardiner et al. 1994) . Notably, dogs which have been diabetic for 5 years show microaneurysms and loss of arteriolar smooth muscle (Gardiner et al. 1994, Fig. 5.5), lesions that are rarely, if ever, observed in diabetic rodents.
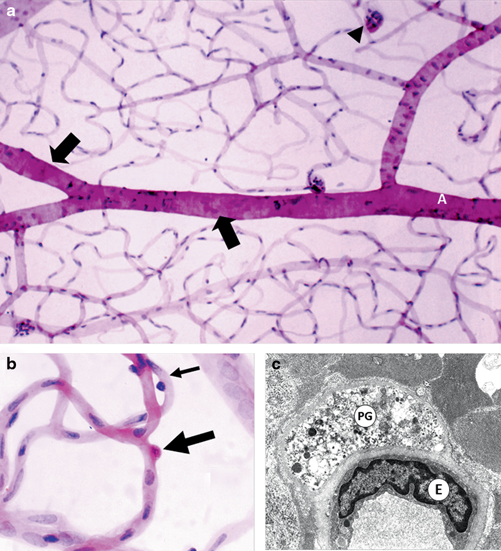
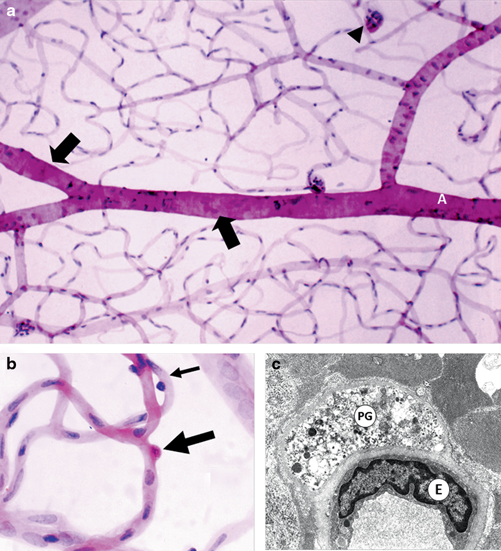
Fig. 5.5
a Retinal vasculature from a 5-year-old diabetic dog using trypsin digest showing microaneurysm (arrow head) and widespread loss of arteriolar smooth muscle (SM) cells (arrows). b Trypsin digest of a 4-year-old diabetic dog showing a pericyte ghost stained red by the PAS technique (large arrow). A viable pericyte staining strongly with haematoxylin is also apparent on the capillary wall (small arrow). c Electron micrograph of a 4-year-old diabetic dog shows a pericyte ghost (PG) represented as a pocket of vesicular debris within the basement membrane (BM). The endothelial cell (E) remains viable. Lumen (L) (With permission of Springer Science+Business Media)
Dogs fed with a galactose-rich diet for a prolonged period of time develop a retinopathy that resembles the early stages of NPDR (Engerman et al. 1990). Galactosaemia leading to DR-like lesions in dogs has been more extensively reported than induced diabetes (Engerman and Kern 1984; Kern and Engerman 1996; Robison et al. 1983) and this model shows retinal dot and blot haemorrhages, pericyte death, capillary loss, microaneurysms starting after approximately 3 years and becoming more confluent after 6 years feeding (Engerman and Kern 1984; Kern and Engerman 1996; Robison et al. 1983). Other retinal vascular lesions, including intraretinal microvessels, arterial occlusion and formation of arteriovenous shunts, have been reported (Kador et al. 2002) . Interestingly, retinal neovascular lesions have been described after prolonged galactose feeding (for up to 84 months; Kador et al. 2002; Cusick et al. 2003) .
Since the profile of DR in dogs is much closer to the clinical scenario, this species has been used to assess drug efficacy (Gardiner et al. 2003b; Kador et al. 1990; Kowluru et al. 2000) . Moreover, diabetes induction in dogs has been used as strong evidence for the glycaemic memory phenomenon observed in patients (DCCT 2014). Engerman et al. studied four experimental groups of dogs including diabetics, non-diabetics, poor control diabetics, good control diabetics and diabetic dogs that were switched from poor to good glycaemic treatment (Engerman and Kern 1987) . Diabetic dogs that were switched to good glycaemic control using insulin injections after 2.5 years of poor glycaemic control, continued to develop retinopathy similar to the poor glycaemic control group (Engerman and Kern 1987). This dog study utilised the long-term advantages of this experimental model to demonstrate that early intensive glycaemic control is capable of delaying the development of DR while an early period of poor glycaemic control carries increased risk .
5.5 Other Large Animals of DR
Pig retina has a cone-rich region known as the area centralis which is somewhat analogous to the primate macula. This combined with the large eye-size for imaging and surgery provides a significant advantage as a model of human DR. While this model has not been extensively reported in comparison to rodents (and even dogs), chemical diabetes induction in pigs is achievable (King et al. 2011) and has often been combined with high-fat diet (Hainsworth et al. 2002) . Following 4 months diabetes , pig retina shows pericyte degeneration, vascular BM thickening and a compromise of the BRB (Lee et al. 2010) .
Although not a commonly used model of DR, diabetes induction in cats (T1D) also produces retinal lesions such as capillary BM thickening, vessel tortuosity, BRB dysfunction, capillary degeneration and microaneurysms (Mansour et al. 1990; Hatchell et al. 1995) .
Non-human primate models of diabetes provide the ultimate model of clinical DR since these animals are anatomically closest to humans and the retina has a macula. There have been several, high-value studies examining retinopathy following T1D and T2D in rhesus monkeys. The Lutty and Tso groups have made a significant contribution to this area and they have reported DR lesions in spontaneously T2D monkeys (Johnson et al. 2005; Kim et al. 2004) and in monkeys with STZ-induced T1D (Tso et al. 1988; Buchi et al. 1996) . These studies have shown, perhaps unsurprisingly, that in primates, DR follows a more clinically linked profile, with variation between subjects but some severe retinopathy occurring especially in the T2D models with up to 12 years of disease. Neural retina abnormalities were present, including ERG defects (Kim et al. 2004) and the lesions included extensive retinal ischaemia with the presence of neural retina infarctions (cotton-wool spots), extensive microaneurysms, capillary degeneration, large vessel occlusions, BRB damage, intraretinal microvascular abnormalities (IRMA) and atrophy of the macula (Johnson et al. 2005). Interestingly, despite of extensive ischaemia, no advanced PDR was apparent (Johnson et al. 2005).
These larger animals have many advantages as models of DR, although the cost disadvantages and inability to use large experimental groups are obvious. The use of non-human primates as models of human disease is also challenging from an ethical point of view and limits the use of these models in DR research.
5.6 Non-mammalian Models of DR
The high cost and ethical constraints of mammalian models of DR have led to the evaluation of the zebrafish ( Daniorerio) as a viable model for some aspects of human disease. These fish have been extensively characterised from the embryological, anatomical and genetic point of view, and combined with their rapid reproductive timescale and improved imaging technology, they provide opportunities for high-throughput in vivo modelling. In the context of DR research, zebrafish have had diabetes induced by direct STZ injection with hyperglycaemia achieved (Olsen et al. 2010) . Interestingly, with this diabetes-inducted model, zebrafish show impaired limb regeneration when compared with non-diabetic counterparts (Olsen et al. 2010) and there is enticing evidence that the glycaemic memory phenomenon also occurs in these diabetic fish (Intine et al. 2013; Sarras et al. 2013; Olsen et al. 2012) .
Some groups have also exposed zebrafish to ‘hyperglycaemic-like’ conditions by adding high glucose to their water. Zebrafish exposed to high glucose for 28 days resulted in neural retina degenerative changes when compared with normal glucose controls (Gleeson et al. 2007) . Furthermore, oscillating high glucose exposure for 30 days caused the zebrafish to lose visual function linked to cone photoreceptor degeneration and a degree of BM thickening and BRB damage (Alvarez et al. 2010) , although it should be appreciated that these fish have a pre-retinal hyaloid vasculature.
< div class='tao-gold-member'>
Only gold members can continue reading. Log In or Register a > to continue
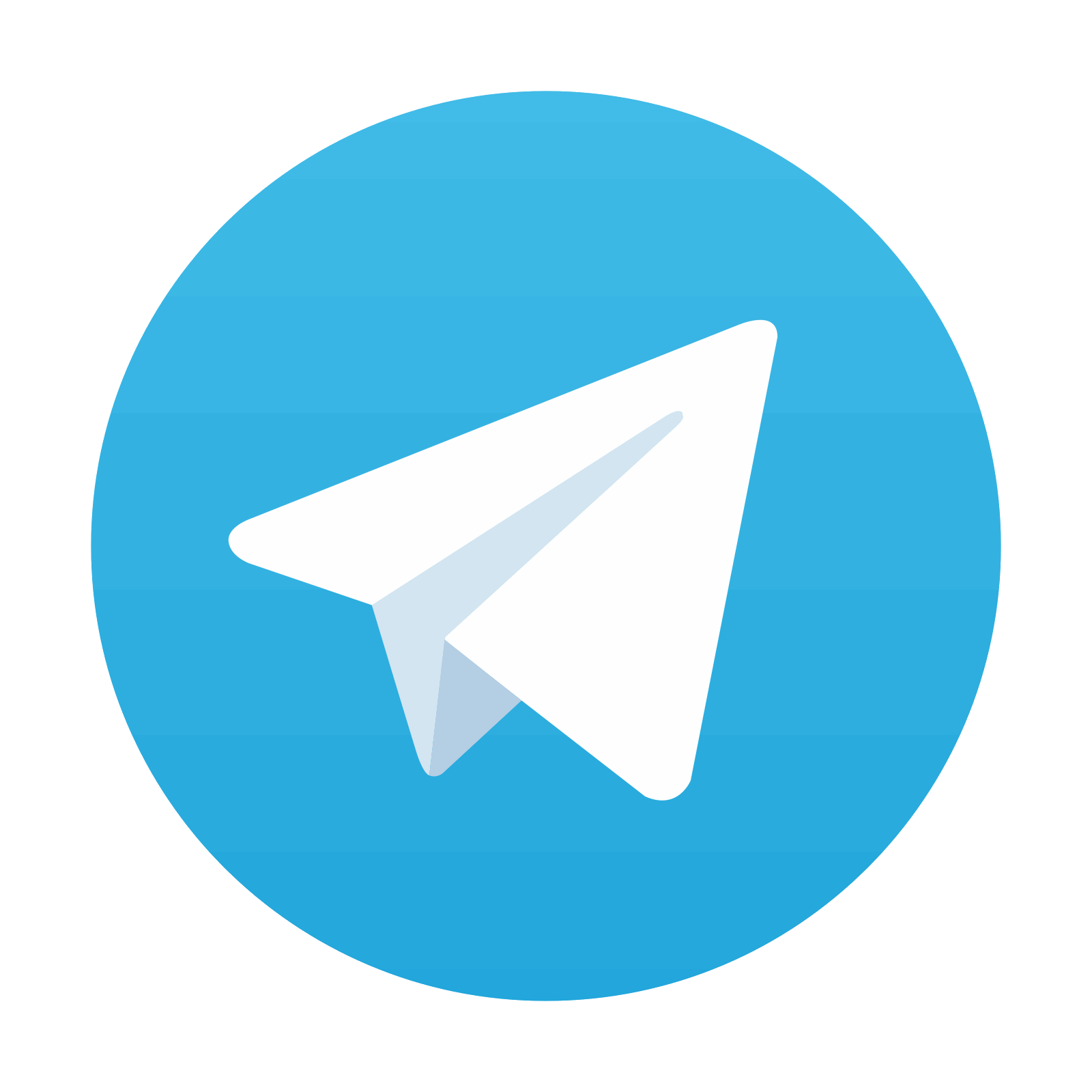
Stay updated, free articles. Join our Telegram channel

Full access? Get Clinical Tree
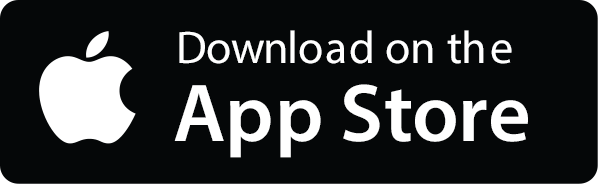
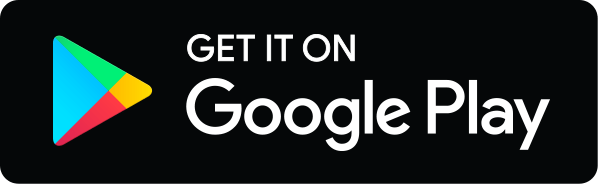