Chapter 3 Anesthesia for ophthalmic surgery
Ophthalmic effects of general anesthetics 37
Injectable general anesthetics 40
Inhalational general anesthetics 40
Neuromuscular blocking agents 41
Ophthalmic drug and anesthetic drug interactions 42
Systemic diseases and general anesthesia 43
Local or regional eyelid injections/nerve blocks 43
Retrobulbar injections/nerve blocks in animals 44
Choice of general anesthetic for selected ophthalmicsurgical procedures 47
Adaptations for large animals and special species 47
Ophthalmic effects of general anesthetics
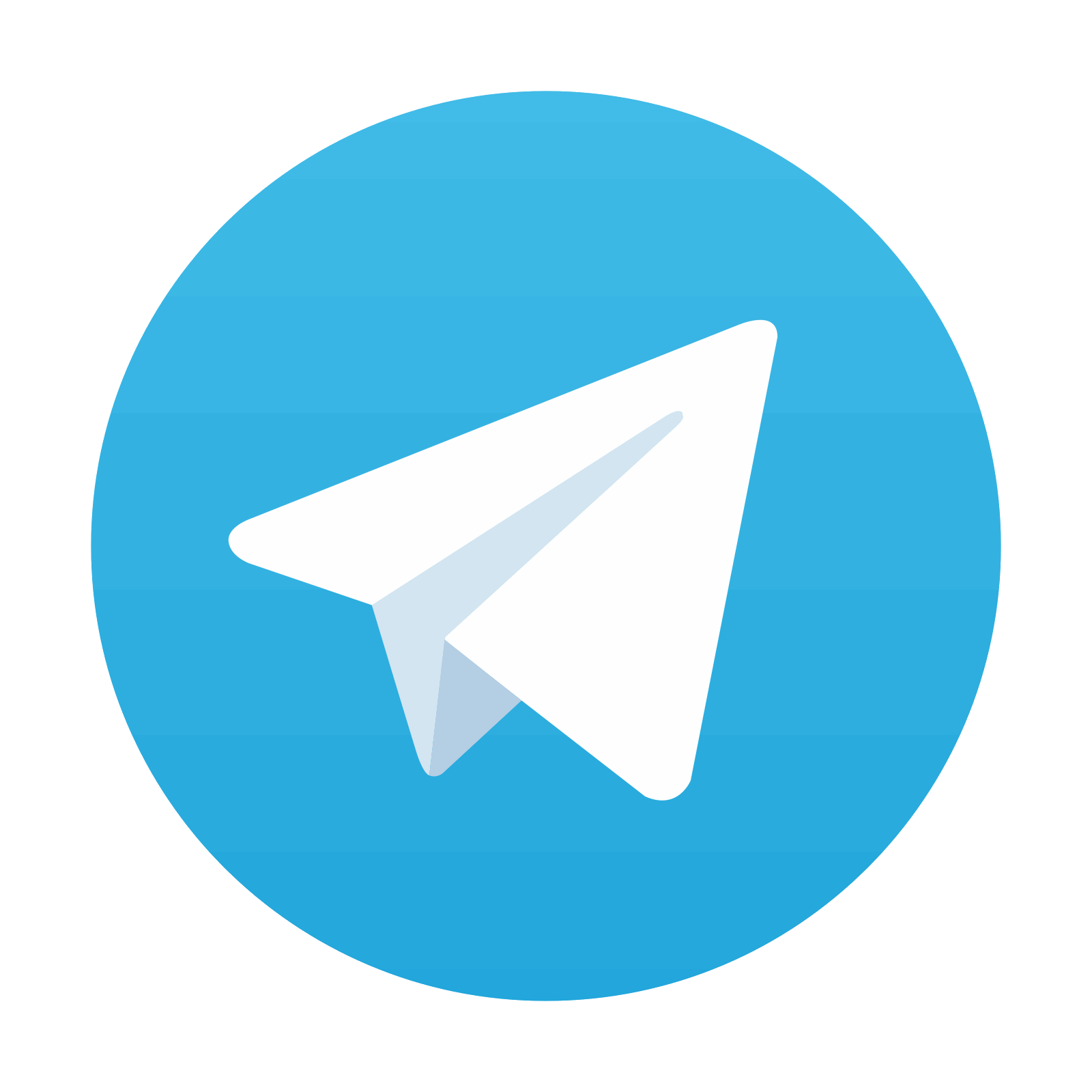
Stay updated, free articles. Join our Telegram channel

Full access? Get Clinical Tree
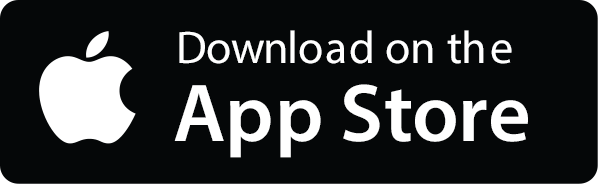
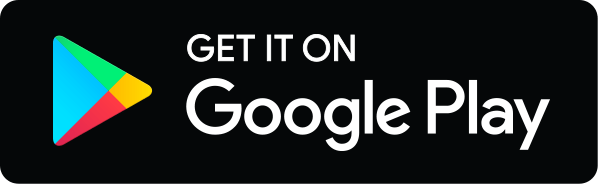