CHAPTER 11 Eye and ear
DEVELOPMENT OF THE EYE
The eye is an organ of remarkable complexity and apparently flawless design. Eyes develop from three sources: (1) the neuroectoderm of the forebrain, (2) the surface ectoderm of the head, and (3) head mesenchyme of neural crest origin between these layers. Ectodermal outgrowth from the brain gives rise to the retina, iris and optic nerve, the surface ectoderm forms the lens, and the surrounding mesenchyme forms the vascular and fibrous coats of the eye.
Optic cup and lens vesicle
The area of the neural plate that gives rise to the eyes is initially a single medial region, the optic field, near the anterior margin of the future prosencephalon. Interactions of the neuroectoderm of the optic field with underlying mesoderm lead to a separation of the single optic field into lateral eye-forming regions. At the end of the third week of gestation in most species, shallow grooves are formed on the sides of the forebrain. With closure of the neural tube, these grooves expand as outpockets of the prosencephalon – the optic vesicles (Figs 11-1, 11-2). The optic vesicles remain attached to the prosencephalon by the optic stalk. It has been shown in the mouse that the transcription factor Rx is expressed very early in the optic field. In its absence, the optic vesicles fail to form.
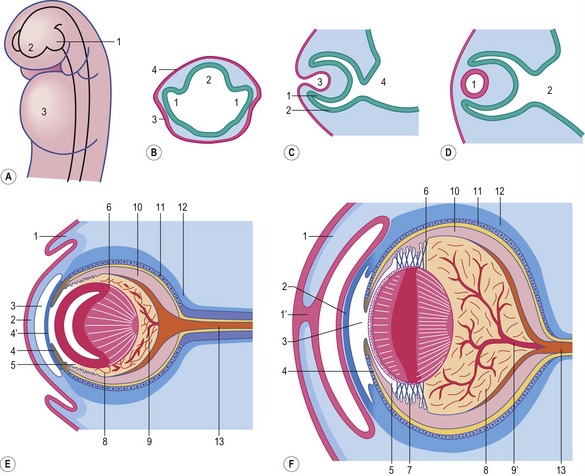
Fig. 11-1: Development of the eye. A: Evagination of the optic vesicles (1) from the prosencephalon (2); 3: Heart. B: Cross section through the optic vesicles (1) and the prosencephalon (2); 3: lens placode; 4: Ectoderm. As a result of the contact of the optic vesicles with the surface ectoderm, the ectoderm thickens and forms the lens placode. C: Longitudinal sections through the developing eye, showing the invagination of the lens placode (3). 1: Inner layer of the optic cup; 2: outer layer of the optic cup; 4: Diencephalon. D: The lens vesicle (1) loses contact with the surface ectoderm and lies in the mouth of the optic cup. 2: Diencephalon. E and F: Differentiation of the eye-ball. 1: Eyelid; 1′: Suture of the fused eyelids; 2: Cornea; 3: Anterior chamber of the eye; 4: Iris; 4′: Pupillary membrane; 5: Posterior chamber of the eye; 6: Lens; 7: Ciliary body with zonular fibres; 8: Vitreous body; 9: Hyaloid artery; 9′: Central retinal artery; 10: Neural layer of the retina; 11: Pigment layer of the retina; 12: Choroid and sclera; 13: N. opticus.
Courtesy Sinowatz and Rüsse (2007).
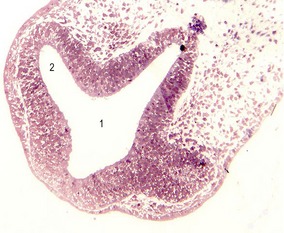
Fig. 11-2: Optic vesicles evaginating from the prosencephalon. Pig embryo of 6 mm CRL. 1: Prosencephalon; 2: Optic vesicle.
Each optic vesicle grows laterally until it comes into contact with the surface ectoderm, where it induces a circumscribed thickening of the ectoderm, the lens placode. This placode subsequently invaginates and forms a lens vesicle, which loses its contact with the surface ectoderm. As the lens vesicles develop, the optic vesicles invaginate and become double-walled structures, the optic cups (Figs 11-1, 11-3). The inner and outer layers of the optic cups are at first separated by a lumen, the intraretinal space, but it soon disappears, and the two layers appose each other. The inner layer of the optic cup later develops into the neural retina with its three layers of neurons that function in visual perception. The outer layer of the optic cup becomes the pigmented layer of the retina (Figs 11-4, 11-5). The optic stalk connects the optic cup with the prosencephalon and, later, the diencephalon; the stalk serves to guide the growth of neuronal axons from the retinal ganglion layer back to the developing brain.
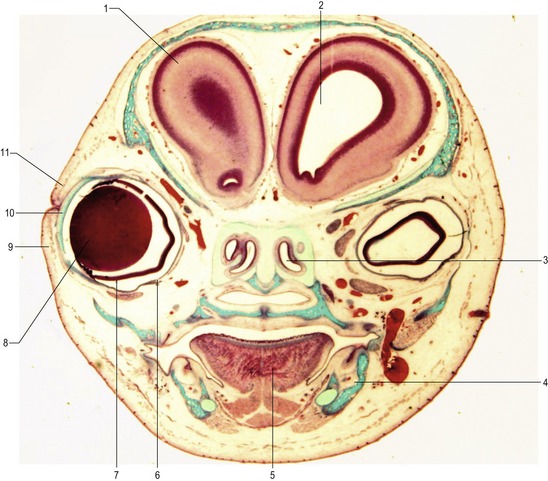
Fig. 11-4: Transverse section of a 45 mm feline fetus. 1: Telencephalon; 2: Lateral ventricle; 3: Nasal cavity; 4: Mandible; 5: Tongue; 6: Pigment epithelium of the retina; 7: Nervous layer of the retina; 8: Lens; 9: Lower eyelid; 10: Cornea; 11: Upper eyelid.
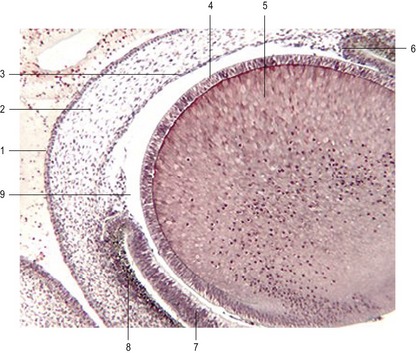
Fig. 11-5: Section through the anterior part of the eye of a 17 mm feline embryo. 1: Anterior corneal epithelium; 2: Corneal stroma; 3: Posterior corneal epithelium; 4: Anterior lens epithelium; 5: Lens fibres; 6: Margin of iris; 7: Neural (sensory) layer of the retina; 8: Pigment layer of the retina; 9: Anterior chamber.
Retina
The retina develops from the optic cups. The outer, thinner, layer, characterized in most species by cells containing small pigment granules, becomes the pigmented layer of the retina. The inner layer of the optic cup thickens, and the epithelial cells begin a complicated process of differentiation into neurons and light receptor cells of the multilayered neural retina (Figs 11-6, 11-7). The outer lips of the optic cup undergo a quite different transformation. They give rise to the iris and ciliary body, which control the amounts of light that reach the retina and the curvature of the lens, respectively.
In the adult, the neural retina is a multilayered structure. The sensory pathway of the retina consists of a chain of three neurons. The first link in the chain is a light- or photoreceptor, either a rod cell or a cone cell. The nuclei of the photoreceptors are located in what is referred to as the outer nuclear layer. The rod and cone cells send processes towards the outer plexiform layer, where they synapse with processes of the second neurons of the chain, the bipolar neurons, the nuclei of which occupy the inner nuclear layer. The other process of each bipolar neuron leads into the internal plexiform layer and establishes synapses with the third neuron in the chain, the ganglion cell. The bodies of the ganglion cells are located in the ganglion cell layer. Their long axons course through the innermost layer of the retina, the nerve fibre layer, towards the optic disc through which they leave the eye in order to reach the brain as the optic nerve (II).
As the number of cells in the inner layer of the early retina increases, the differentiation of the neurons begins. There are two major gradients of differentiation of the retina: the first proceeds more or less vertically from the inner to the outer layers; the second is more horizontal, from the centre towards the periphery. Differentiation in the horizontal gradient starts with the appearance of ganglion cells. As the ganglion cell layer is established, premature differentiation of neighbouring neural precursor cells is prevented by expression of the Notch gene. The protein coded by Notch keeps these cells in an undifferentiated state that will be overcome later by differentiation signals from the neighbourhood of the neuroblasts. Differentiation of amacrine and horizontal cells completes the differentiation of the inner and outer nuclear layers of the retina. These neurons send out processes that contribute to the definition of the inner and outer plexiform layers. The last retinal cells to differentiate are the bipolar neurons and receptor cells – the rod and cone cells.
Optic axon guidance
At a later stage of retinal differentiation, axons from the ganglion cells grow along the innermost layer of the retina towards and into the optic stalk, following molecular cues. The optic stalk connects the retina with the diencephalon (Fig. 11-3). On its ventral surface the stalk has a groove, the choroid fissure, that contains the hyaloid vessels. The optic stalk also serves as a guide directing axons from the ganglion cells of the retina back into the developing brain.
Attachment of the retina
The cleft between the inner and outer layer of the optic cup is never completely obliterated, even into adulthood, and so no firm attachment between the pigment epithelium and the nervous epithelium of the retina is established. The major factor keeping the two layers in contact is the maintenance of normal intraocular pressure, a major function of the aqueous humour and vitreous body.
Iris and ciliary body
Differentiation of the iris and ciliary body occurs at the lip of the optic cup, where the neural and pigment layers of the retina meet. The iris develops by the peripheral extensions of both layers of the optic cup so that they cover the edges of the lens vesicle. This brings the lens vesicle entirely inside the boundary of the optic cup (Fig. 11-1). Thus the iris consists of an inner non-pigmented epithelial layer and an outer pigmented layer, which are continuous with the neural and pigmented layers of the retina, respectively. The stroma of the iris is of neural crest origin and migrates into the iris separately. The muscles of the iris, the sphincter pupillae and dilator pupillae are, interestingly, of neuroectodermal origin; they result from a transformation of the anterior epithelial layers of the iris into smooth muscle cells. The levels and distribution of pigmentation in the iris determine eye colour. The bluish colour of the iris seen in most newborn animals is caused by the pigmentation of the outer pigmented layer of the iris. Pigment cells, however, also appear in the stroma of the iris; the greater the density of pigment cells in the stroma, the browner is the eye colour. Definitive pigmentation of the eye develops during the first months of postnatal life.
The ciliary body, containing the ciliary muscle, takes shape between the iris and the neural retina. The neural-crest-derived ectomesenchyme of this area proliferates unevenly and forms a series of ridges (processus ciliaris) that are covered by a two-layered epithelium (Fig. 11-1). The pigmented epithelium of the ciliary body is derived from the outer layer of the optic cup and is continuous with the retinal pigmented layer. The inner epithelial layer is continuous with the neural layer of the retina posteriorly and the epithelium of the iris anteriorly. This inner epithelium later becomes the source of aqueous humour. The ciliary body is connected to the lens by a set of radial elastic fibres (zonular fibres) forming the suspensory ligaments of the lens. Contraction of the ciliary muscle, which is derived from the ectomesenchyme within the ciliary body, diminishes the tension of the zonular fibres and results in a more spherical, relaxed shape of the lens, critical to the focusing of the lens.
Lens
When the optic vesicles contact the surface ectoderm, the ectoderm thickens to form the lens placodes (Fig. 11-1). These structures subsequently invaginate and form the lens vesicles, which break away from the surface ectoderm. Shortly after formation of the lens vesicles, cells of the posterior wall of the vesicles elongate to form primary lens fibres which fill the hollow lens vesicle cavity and thereby transform it into a solid lens (Fig. 11-5). Growth of the lens is not finished at this stage; new, secondary lens fibres are continuously added to the central core of the lens, extending almost pole to pole. On the posterior side of the lens, less differentiated cells that do not elongate move to the poles of the lens, proliferate and serve as a source for new lens fibres. The cells of the lens secrete a basal-membrane-like, elastic material rich in glycoproteins that covers the surface of the lens. This lens capsule contributes to the elastic properties of the lens, essential to its function. Zonular fibres, essentially collagenous fibres that are formed from mesenchymal cells situated between the ciliary body and the lens, attach to this capsule.
< div class='tao-gold-member'>
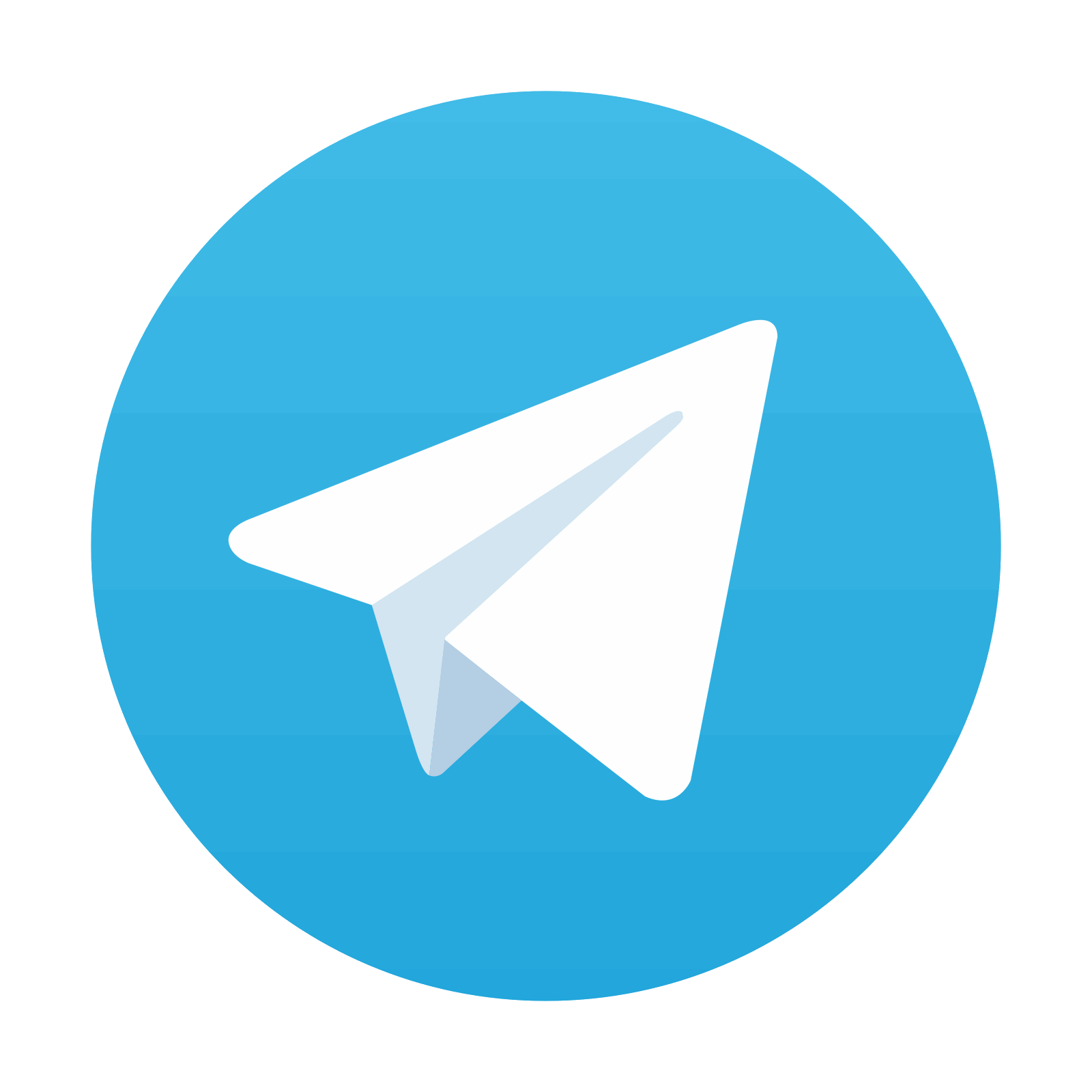
Stay updated, free articles. Join our Telegram channel

Full access? Get Clinical Tree
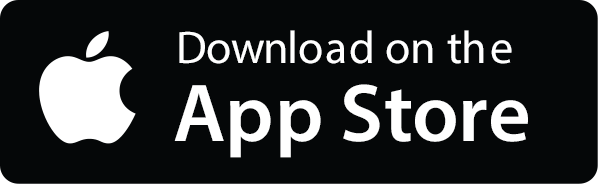
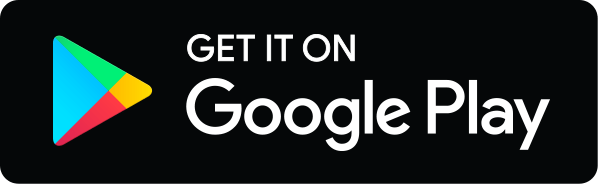