Mineral and Antioxidant Management of Transition Dairy Cows
Ian J. Lean, BVSc, DVSc, PhD, MANZCVSa∗, Robert Van Saun, DVM, MS, PhDb and Peter J. DeGaris, BVSc (Hons), PhDc, aSBScibus, PO Box 660, Camden, New South Wales 2570, Australia; bDepartment of Veterinary & Biomedical Sciences, Pennsylvania State University, University Park, PA 16802, USA; cTarwin Veterinary Group, 32 Anderson St, Leongatha, Victoria 3953, Australia. E-mail address: ianl@sbscibus.com.au ∗Corresponding author.
Keywords
Transition
Calcium
Skeleton
Antioxidant
Vitamins
Introduction
Although controlling disorders of macromineral metabolism, and in particular milk fever, forms a small part of the overall management of the transition cow, it is often the focus at a producer level. As a result, it is critical to ensure that any transition cow program is effective in controlling macromineral disorders. Further, recent developments in understanding of the role of calcium in metabolism and bone as an integrator of metabolism reinforce the need to ensure that there is careful attention to calcium metabolism. The concept of milk fever and hypocalcemia being central to the interactions of other diseases has been well understood since the pivotal studies of Curtis and colleagues.1 Recent understandings of the role of bone in integrated metabolism (reviewed later) provide a basis on which to understand the gateway role of milk fever in other disorders and reproduction.
The pathophysiology of hypocalcemia and dietary manipulations to control the risk of milk fever have been extensively reviewed,2–5 and insights from these reviews are incorporated in this article. Further, the understanding that transition management needs to be fully integrated to be effective6 is discussed in the context of review of a study that integrated these priniciples.7–9 Micronutrient needs are addressed in the context of vulnerability of cattle to oxidative stress and inflammatory disorders. This article concludes with a series of practical approaches to improving transition diets.
Milk Fever Control
The following recommendations for the dietary control of hypocalcemia are based on 4 meta-analyses examining factors influencing the risk of milk fever.10–13 These meta-analyses showed that the risk of milk fever can be predicted from dietary levels of calcium, magnesium, phosphorus, dietary cation-anion difference (DCAD) (as calculated by [Na+ + K+] − [Cl− + S2−]), breed of cattle, and duration of exposure to the diet. To effectively prevent these disorders, careful attention is needed to concentrations of calcium, magnesium, and phosphorus as well as the DCAD of the prepartum diet (Table 1).
Table 1
aBreed 1, Jerseys (Holstein Friesian used as the reference breed).
bBreed 2, Norwegian Red and White (Holstein Friesian used as the reference breed).
cCa, Mg, and P expressed as % of DM.
dDCAD 1 = (Na + K) − (Cl + S) in mEq/100 g DM.
eExposure is the mean time in days that the cows in a study were exposed to the precalving transition diet.
From Lean IJ, DeGaris PJ, McNeil DM, et al. Hypocalcemia in dairy cows: meta analysis and dietary cation anion difference theory revisited. J Dairy Sci 2006;89:673.
DCAD
The DCAD theory of milk fever prevention has its basis in the strong ion model of acid: base balance,14 modified in the 1980s15 and simplified in the late 1990s.16 Some contention still exists regarding the most appropriate equation for predicting DCAD. Charbonneau and colleagues13 preferred the equation (Na+ + K+) − (Cl− + 0.6 S2−) on the basis that it was the best equation at predicting blood pH, whereas DeGaris and Lean3 preferred the equation (Na+ + K+) − (Cl− + S2−), because the equations were equivalent for predicting the risk of milk fever. Given that the equations are equivalent for predicting milk fever, we recommend use of the latter and more simple equation.
The simplified strong ion model17 to predict plasma pH is:
where pK′1 is the ion product of water, Ka is the effective equilibrium disassociation constant for plasma nonvolatile weak acids, [SID+] is the strong ion difference, [ATOT] is the plasma nonvolatile weak acid concentration, S is the solubility of CO2 in plasma, and pco2 is the partial pressure of CO2 in plasma. The implication of this equation is that the major variable factor that can be readily influenced is the strong ion difference and prevention of milk fever involves, in part, the appropriate application of DCAD theory to reduce the strong ion difference ([SID+]) by lowering plasma pH and producing strong ion metabolic acidosis. This goal can be achieved by feeding salts of the strong cations (CaCl2, CaSO4, MgCl2, MgSO4, NH4Cl, and (NH4)2SO4) or acids of the anions (HCl and H2SO4). The strong cations Ca2+, Mg2+, and NH4+ are absorbed to a lesser extent from the gastrointestinal tract (GIT) than are the strong anions Cl− and SO42−. The differential absorption results in a relative excess of absorbed anions compared with absorbed cations lowering the [SID+] and subsequently plasma pH. Because Na+ and K+ are absorbed with near 100% efficiency in the intestine, NaCl and KCl have a net effect of zero on the [SID+].
• Metabolic acidosis in goats18,19 and cattle20
• Decreased renal sensitivity to parathyroid hormone (PTH) in cows fed a strongly positive DCAD precalving diet20,21
• Enhanced renal production of 1,25(OH) vitamin D3 in response to a low-DCAD precalving diet20,21
• Increased responsiveness of target tissues to 1,25(OH) vitamin D3 associated with increased calcium absorption from the intestinal tract22
• Increased resorption of calcium from bone stores23–25
Critically, the overall effect is to increase calcium turnover through increased GIT absorption and increased sensitivity of target tissues to homeostatic signals, rather than an improvement in overall calcium balance. The meta-analyses of milk fever risk factors10–13 have identified that the effect of DCAD on the risk of milk fever is linear and independent of the important effects of dietary Ca, Mg, and P concentrations. Consequently, any reduction in the DCAD decreases the risk of milk fever. This linear relationship should not be confused with the curvilinear relationship between DCAD and urine pH, with DCAD having little impact on urine pH until it reaches approximately 20 mEq/100 g dry matter (DM) (Fig. 1). This curvilinear relationship is caused by renal buffering systems that maintain an alkaline urinary pH until overwhelmed. Although recommendations exist for target urine pH to ensure adequate acidification, these assess only effectiveness of DCAD management of the diet and not the risk of milk fever.
Calcium
The optimum concentration of dietary Ca intake for the control of milk fever is also contentious, with Lean and colleagues6 and Thilsing-Hansen and colleagues5 suggesting that the precalving intake of calcium be limited to 60 on a negative DCAD diet and 20 g per day, respectively. McNeill and colleagues2 also concluded that excessive calcium intake was an important risk factor for milk fever, but less so than potassium. However, Goff4 concluded that calcium concentration in precalving diets had little influence on the incidence of milk fever when fed at levels higher than the daily requirements of the cow (approximately 30 g/d). Oetzel29 recommended a daily intake in the precalving diet of 150 g/d, a calcium concentration of between 1.1% and 1.5% of DM, in conjunction with a dietary DCAD of approximately –15 mEq/100 g DM. However, the meta-analyses of Oetzel10 and Lean and colleagues12 found that a calcium concentration of 1.1% to 1.5% of DM provided near-maximal risk of milk fever (Fig. 2). When the effects of length of time cattle were exposed to a transition ration before calving were investigated, a quadratic interaction with calcium was found.12 This relationship suggests that short exposures to high concentrations of calcium markedly increase milk fever risk, whereas a prolonged exposure to the same concentrations produces only a moderate risk (Fig. 3). These observations may explain the differences in recommended calcium concentrations of different workers.
The total exchangeable body calcium mass is only 1.5% of total body calcium in mature cows.30 Goff and colleagues21 estimated an even smaller pool of readily labile calcium bone stores, 6 to 10 g, based on responses of cattle to ammonium chloride-induced acidosis.31 We have observed mild milk fever cases arising before calving with low-DCAD and low-calcium diets, possibly reflecting calciuria stimulated by the low-DCAD diets, and find that diets containing 0.4% to 0.6% calcium overcome this problem.
Magnesium
The most significant factor influencing risk of milk fever is the magnesium content of the diet.12 Magnesium may prevent milk fever through roles in
• The release of PTH and in the synthesis of 1,25-dihydroxycholecalciferol
• In hypomagnesemic states, kidney and bone are less responsive to PTH4,32
• Reducing renal calcium excretion. Wang and Beede33 found that nonpregnant, nonlactating cows fed a diet high in Mg had lower renal calcium excretion than those fed a diet low in Mg
Contreras and colleagues34 and van de Braak and colleagues35 reported poor calcium mobilization in hypomagnesemic cattle. Although clinical hypomagesemia is rare in dairy cattle, very low dietary Na or high dietary K concentrations may interfere with Mg transport across the rumen wall and result in clinical disease. Magnesium is best supplied as magnesium sulfate, magnesium chloride, and magnesium oxide; caution needs to be applied to supply in the chloride form, because this is unpalatable (we have observed successful application in water). As with any other mineral, it should not be assumed that more is better; we have observed problems associated with supply of 0.8% magnesium in diets.
Phosphorus
Phosphorus also plays an important role in milk fever, with increasing phosphorus concentrations increasing milk fever risk. Although phosphorus concentrations are not as tightly regulated as calcium, both are closely related, with plasma PO4 concentrations regulated directly by 1,25(OH) vitamin D3 and indirectly by the PTH/calcium negative feedback loop.36 However, in rats, hyperphosphatemia can inhibit the renal production of 1,25(OH) D3 sufficiently to cause hypocalcemia.37–39 In cattle, there is evidence that a prepartum diet high in phosphorus can have a negative impact on calcium homeostasis, possibly by the same pathways.40–42 Hypophosphatemia may contribute to the alert downer cow syndrome and long-term dietary P deficiency has been implicated in the development of postparturient hemoglobinuria; however, it is likely that the latter disease is multifactorial, with copper, selenium, and antioxidant status playing an important role in the development of the disease.
Optimum Duration of Exposure
The duration of exposure to the transition diet was included in the models to predict milk fever risk developed by Lean and colleagues,12 but had not been validated in trial work. Subsequent studies tend to support an optimal period of exposure to transition diets of 25 days before calving.9 Increased urinary calcium loss on low-DCAD diets has been reported,43,44 and depletion of calcium stores over time may explain part of this effect.
Age and Protein
There is good evidence that increasing age increases the risk of milk fever as a result of decreased intestinal calcium absorption and responsiveness to hypocalcemia,45–47 reduced bone turnover,48 and decreased bone responsiveness to PTH and vitamin D.21 We found that the risk of milk fever increased by 9% per lactation in the subpopulation of studies used for a meta-analysis12 that reported age (unpublished data).
Increased protein concentration in the diet increased the risk of milk fever in some models and approached significance in many models tested by Lean and colleagues12 (unpublished data). This effect was not large compared with magnesium and calcium concentrations. However, too few studies provided data on this finding to include in final published meta-analytical models.
Importance of Bone in Energy Metabolism
Important homeostatic links between bone and energy metabolism have been established. This relationship was first postulated when obesity was discovered to reduce the risk of osteoporosis in humans.49 Ducy and colleagues50 then proposed that the bone and energy metabolism may be regulated by the same hormones. Mouse models show that osteocalcin, produced by mature osteoblasts, completes the negative feedback loop between bone and energy metabolism, which is the hallmark of homeostatic regulation.51 The uncarboxylated form of osteocalcin promotes
Although there is no specific research in cattle linking bone and energy metabolism, there are findings that support the hypothesis that these may be linked, and this interaction seems to be a vital aspect of the homeorhetic adaptations to lactation. Many studies support this conceptual framework at the physiologic level, and clinically, Heuer and colleagues52 found that obese cows (body condition scoring [BCS] >4.5/5) were at greater risk of milk fever. Binger and colleagues53 found an increase in insulin resistance in cows fed low-DCAD rations before calving. DeGaris and colleagues8 found a positive relationship between BCS and area under the curve of blood Ca after calving, and reanalysis of the data used in that study using time series techniques found significant, positive correlations between blood calcium and glucose both before and after calving (DeGaris, and Lean, unpublished data). Associations between ketosis and hypocalcemia are well recognized and have been recently reconfirmed in prospective, randomized trials with anionic diets54 and cohort studies examining the effects of hypocalcemia.55
Critically, links between calcium metabolism and health and reproduction are also evident. Hypocalcemia is a risk factor for many of the important diseases of lactation, including mastitis, ketosis, retained placenta, displaced abomasums, and uterine prolapse. Hypocalcaemia is also a risk factor for reproductive disorders and is an indirect risk factor for increased culling.25,56,57 Curtis and colleagues58 observed an increased odds of mastitis of 8-fold for cows with milk fever. Although such increases have been ascribed to recumbency and failure of teat sphincter closure, these mechanisms are speculative. Cows with subclinical hypocalcemia (defined as <8.59 mg/dL) were at greater risk of developing fever, metritis, and puerperal metritis compared with normocalcemic cows.55 There are differences in peripheral mononuclear cells function, which indicate impaired function in hypocalcemic cows.59 Borsberry and Dobson60 in the United Kingdom found that cows with clinical milk fever had 13 more days from calving to conception, a finding supported by Martinez and colleagues55 in hypocalcemic cows. These investigators also found lower conception rates in hypocalcemic cows and that 66.6% of metritis and 91.3% of puerperal metritis in this population was attributable to hypocalcemia. New Zealand studies61,62 found a tendency to improved interval to conception in cows treated with calcium-containing products after calving. Although further targeted research is needed to explore this potentially important aspect of energy and bone metabolism in dairy cattle, those evaluating or formulating diets should work on the premise that these links between bone health and energy metabolism are substantial.
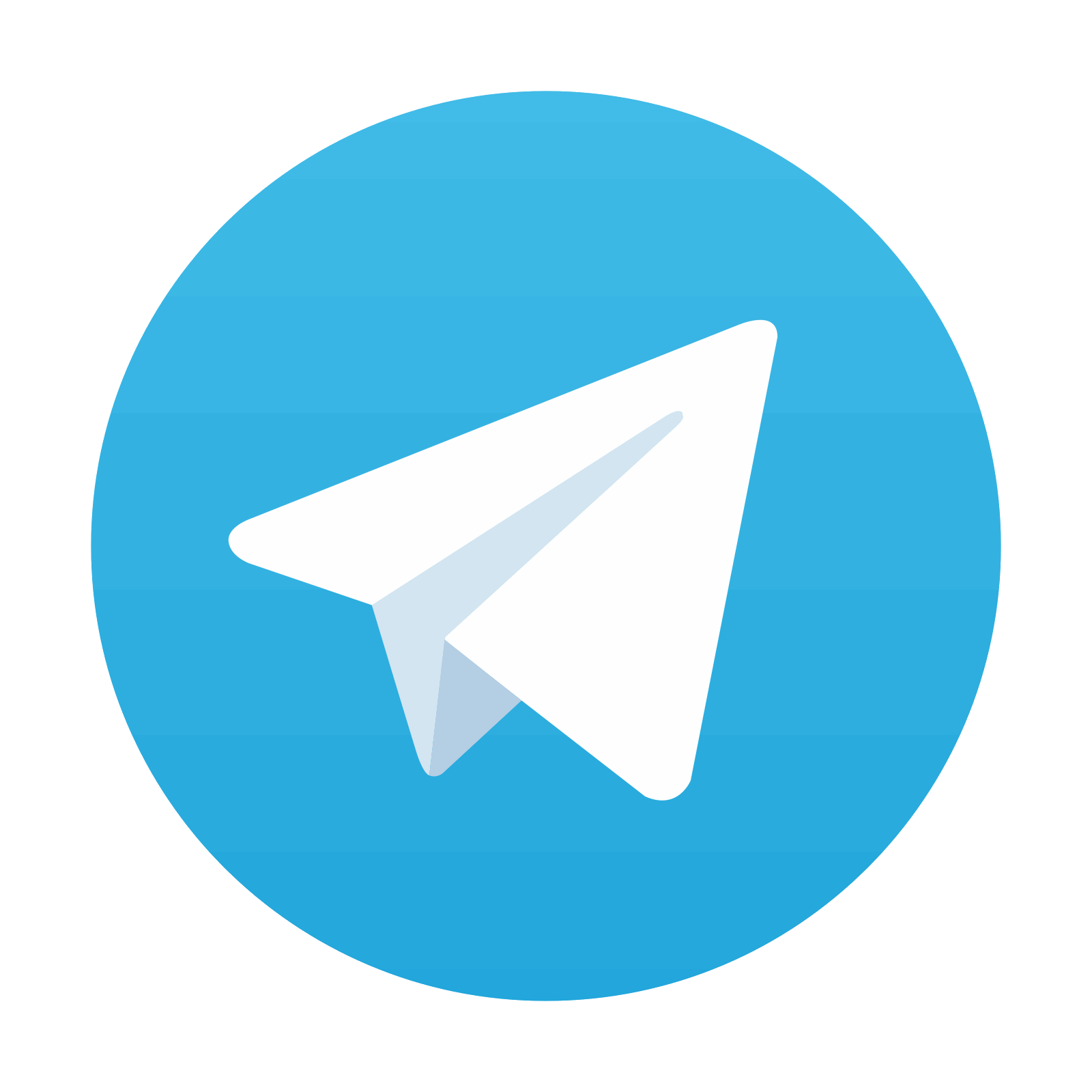
Stay updated, free articles. Join our Telegram channel

Full access? Get Clinical Tree
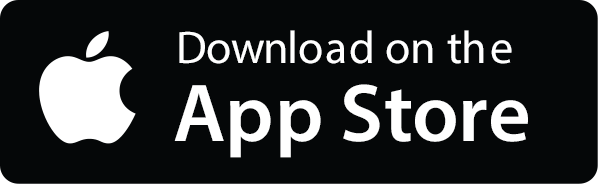
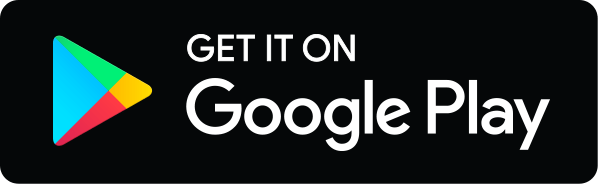