Chapter 12
Anaesthesia of cattle
Introduction
Cattle are by no means good subjects for heavy sedation or general anaesthesia. Regurgitation followed by aspiration of ruminal contents into the lungs can easily occur. Once a ruminant animal is in lateral or dorsal recumbency, the oesophageal opening is submerged in ruminal material, normal eructation cannot occur, and gas accumulates. The degree of bloat depends on the amount of fermentation of the ingesta and on the length of time that gas is allowed to accumulate. Gross distension of the rumen becomes a hazard if anaesthesia or recumbency is prolonged and regurgitation can follow from this. In addition, the weight of the abdominal viscera and their contents prevents the diaphragm from moving freely on inspiration and ventilation becomes shallow, rapid and inefficient for gas exchange within the lungs.
The danger of regurgitation and inhalation of ingesta is always present but the likelihood of it occurring can be minimized by:
3.When the animal is in lateral recumbency during anaesthesia arranging that the occiput is above general body level and that the head slopes so that saliva and any regurgitated material runs freely from the mouth (Fig. 12.1).
Figure 12.1 Animal’s head inclined over a support to allow saliva and any regurgitated ruminal content to drain out of the mouth.
An additional procedure to prevent regurgitation that has been tried but not often used is to pass a modified stomach tube as far down the oesophagus as possible. The tube has a balloon firmly attached to its end that can be inflated to obstruct flow of ingesta from the rumen into the pharynx.
Regurgitation occurs during both light and deep anaesthesia so that it is probable that two mechanisms are involved in the process. During light anaesthesia, ingesta may pass up the oesophagus into the pharynx as a result of an active, but uncontrolled, reflex mechanism. It is then a matter of chance whether or not the protective reflexes, e.g. laryngeal closure, coughing, etc., are active and can or cannot prevent aspiration. Fortunately, laryngeal closure often occurs but, as hypoxia develops, at some point, the animal will take a large breath and any ingesta accumulated in the pharynx will be aspirated. The order in which the reflexes of laryngeal closure, coughing, swallowing, and regurgitation disappear as anaesthesia is deepened differs from one anaesthetic drug combination to another but the relative safety of the various agents is not documented. During deep anaesthesia, on the other hand, regurgitation is a passive process. The striated muscle of the oesophagus loses its tone and any increase in the intraruminal pressure – whether from pressure on the abdominal wall from a rope or belly band or from gas accumulation in the rumen itself – may force ingesta up into the pharynx. The protective reflexes are not active and aspiration may occur easily.
Regurgitation and pulmonary aspiration is a real and serious hazard in sedated or anaesthetized cattle.
Tracheal intubation is not always performed in all sedated or anaesthetized recumbent bovine animals and regurgitation does not always occur. However, some animals will regurgitate, and this has an unreasonably high risk for fatal outcome. Should regurgitation occur during the induction of anaesthesia before endotracheal intubation has been accomplished, the endotracheal tube may be immediately passed into the oesophagus and its cuff inflated so that the regurgitated material passes along the tube and out of the mouth. The trachea can then be intubated with a second tube, taking care to cover the end of the tube to avoid scooping material into its lumen. In actuality, the presence of one endotracheal tube in the pharynx often makes passage of a second tube nearly impossible due to the small size of the bovine pharynx. One option is to intubate the trachea with a stomach tube and attempt to feed the second endotracheal tube over the stomach tube. An alternative is to wait until the flow of ingesta stops, remove the tube from the oesophagus and to rapidly intubate the trachea. However, if the conditions initiating the regurgitation such as ropes tight around the thorax and abdomen have not been removed, or the depth of anaesthesia is light, regurgitation may recommence during the removal of the endotracheal tube from the oesophagus.
Salivation continues as a copious flow throughout general anaesthesia but the loss of saliva is unlikely to produce a significant effect on acid–base status. Antisialagogues are not of much use for they make the secretion more viscid in nature and do not significantly reduce its production. It is important to arrange the head of the anaesthetized animal so that saliva drains from the mouth and does not accumulate in the pharynx. Intubation with a cuffed endotracheal tube will prevent inhalation of saliva.
In two reports involving restraint of unsedated cows or bulls in dorsal or lateral recumbency, a decrease in PaO2 from an average standing value of 11.4 kPa (86 mmHg) to less than 9.3 kPa (70 mmHg) was measured, with a decrease to below 6.6 kPa (50 mmHg) in some individuals (Semrad et al., 1986; Klein & Fisher, 1988). Arterial PCO2 remained at or slightly below 5 kPa (38 mmHg). In another investigation of cows from which food was withheld for 18 h, mean PaO2 in standing animals was 14.5 kPa (109 mmHg) (Wagner et al., 1990). Significant decreases in PaO2 occurred in both lateral and dorsal positions but only in dorsal recumbency did values decrease to below 9.3 kPa (70 mmHg). These changes indicate that even in unsedated cattle, recumbency creates abnormalities of ventilation and perfusion that are not counterbalanced by normal compensatory mechanisms.
In contrast, when calves 25–53 days of age that had milk replacer withheld for 12 hours were restrained in dorsal recumbency for 95 minutes, the cardiopulmonary changes induced by the change in body position were much less than previously described for adult cattle, perhaps due to the lesser weight of the abdomen on the diaphragm or the caudal vena cava (Meyer et al., 2010). Cardiac output decreased by 15%, O2 delivery decreased with no change in O2 extraction ratio, and respiratory rate (RR) and systemic vascular resistances increased. PaO2 and PaCO2 were unchanged.
Withholding feed before sedation and anaesthesia may reduce pressure on the diaphragm, limit lung collapse and modify the decrease in PaO2. In a study of fed and non-fed cows anaesthetized with halothane and breathing oxygen, cows fed before anaesthesia had a progressive decrease in PaO2, reaching hypoxaemic levels after an hour of anaesthesia, whereas cows that were starved before anaesthesia were well oxygenated (Blaze et al., 1988). Severe hypercapnia was measured in both groups with a greater increase measured in the fed cows. Thus, hypoxaemia may develop in recumbent non-starved cattle even when inhaling high O2 concentrations but supplementation of inspired air with O2 may prevent hypoxaemia if the cow has been starved first. Respiratory acidosis usually develops in anaesthetized cattle.
Normal values for cardiovascular parameters in unsedated healthy adult cattle are approximately 73 ± 14 beats/min (mean ± SD) for heart rate, 150 ± 27 mmHg for mean arterial pressure (MAP), and 64 ± 14 mL/kg/min for cardiac output (CO) (Wagner et al., 1990). Withholding feed for 48 h has been determined to cause significant decreases in heart rates in cattle (Rumsey & Bond, 1976; McGuirk et al., 1990). Normal values in calves 2–26 days old have been measured at approximately 112 beats/min, 117–127 ± 16 mmHg for systolic arterial pressure (SAP), 77–83 ± 15 mmHg for diastolic pressures (DAP), on average 94–98 mmHg for MAP, and on average 150 mL/kg/min for CO (Kerr et al., 2007). Normal values measured in calves 25–53 days old were on average heart rates 105–125 beats/min, 84–96 mmHg for MAP, and 205 mL/kg/min for CO (Meyer et al., 2010).
Restraint
The majority of bovine clinical surgery is carried out under local analgesia, frequently in the standing animal. Surgical procedure is made easier by use of appropriate sedation and/or restraining ‘crushes’ or ‘chutes’. Ropes are useful additions for restraining sedated or unsedated cattle and anyone in cattle veterinary practice soon becomes expert at tying quick-release knots, tying bowline knots for rope loops, applying Reuff’s method of casting a mature bovine by squeezing with a neck loop and two half-hitches around the body, and assembling figure of eight ties to secure flexed front and hind limbs.
Electrical devices are available in some countries to immobilize cattle by causing muscle tetanus from application of an electrical current between electrodes at the lip and rectum. There are concerns that this technique is neither humane nor analgesic. Holstein cows trained to be led with a halter and enter a set of stocks were observed for behavioural and physiological responses to either immobilization by application of an electric current for 30 s from a commercially available electroimmobilizer or to an intramuscular injection with an 18 gauge needle (Pascoe, 1986). The electrical immobilization was associated with significant aversive behaviour and evidence of distress and the results led the author to believe that electroimmobilization was a strong noxious stimulus that was remembered for several months.
Sedation and analgesia
Cattle may not strongly exhibit behavioural signs commonly associated with pain, which may lead to the mistaken perception that bovine animals and young calves do not require analgesia such as is afforded to horses, dogs, and cats. Administration of sedatives and analgesics, including local nerve blocks and non-steroidal anti-inflammatory drugs (NSAIDs), not only fulfills our moral obligation to provide analgesia during surgical procedures but also may increase the safety of the veterinary practitioner during performance of the procedure. These factors must outweigh the economic concern of the cost and increased time involved in administration of analgesic agents to the large numbers of calves that must be castrated and dehorned, and for other disabilities and surgeries. Surveys completed by practitioners who work with cattle indicate an increased awareness by practitioners for the need to provide analgesia in this species (Huxley & Whay, 2006; Hewson et al., 2007; Thomsen et al., 2010; Fajt et al., 2011). Commonly expressed comments were that administration of analgesic drugs makes it safer to work with cattle, that the potency and duration of action of available drugs were considered very important, and that there is a shortage of analgesic drugs available that are both long acting and cost effective. When analgesics were administered, they most commonly were NSAIDs, local anaesthetics, and α2-adrenergic receptor agonists.
Respondents to the questionnaires were asked to assign pain scores to a variety of disease conditions and surgical procedures and although moderate pain scores were assigned for castration, a high proportion of respondents in North America did not provide analgesia for castration (Fajt et al., 2011). Even when a high pain score was assigned, for example for claw amputation, caesarian section, and umbilical hernia repair, only approximately 60% of practitioners administered NSAIDs for postoperative pain control (Huxley & Whay, 2006; Fajt et al., 2011). Current updates on analgesia practices must include consideration that administration of local anaesthetics and sedatives at the time of the procedure does not guarantee analgesia postoperatively.
Choice of drugs may be influenced by current legislature concerning use of anaesthetic agents in food-producing animals. The licensing of withdrawal periods is done by national agencies so that not only are the kinetics of individual drugs considered but also regional practices and requirements. Human ingestion of drug residues of anaesthetic agents at injection sites has the potential of inducing toxicities or allergic reactions. Requirements of national licensing authorities are not universal. The food safety approach of European agencies specifies a withdrawal period based on a whole-of-life maximal residue limit whereas USA, Canada, and Australia evaluate the risk based on acute exposure (Reeves, 2007).
Many of the anaesthetic agents are administered to cattle as extralabel use, for example ketamine, and some countries offer recommended withdrawal times for meat and milk. In the USA, recommended withdrawal after ketamine is 3 days for meat and 48 hours for milk, however, recent pharmacokinetic measurements after administration of ketamine, 5 mg/kg IV, to lactating Holstein cows indicate that milk withdrawal probably should be extended to at least 60 hours (Sellers et al., 2010). Persistence of plasma concentrations will be altered by dose administered such that a terminal half-life of approximately 30 minutes was measured following a subanaesthetic dose of ketamine, 0.1 mg/kg IV, given in combination with a sedative dose of xylazine for castration in 4–6 month old Angus calves (Gehring et al., 2008). In Europe, if the drug has a licence for any food animal (including the horse) then withdrawal times for a food animal species for which it does not have a licence are (at the time of writing) 28 days for meat and 7 days for milk. However, any animal that has received certain drugs may never go for human consumption. For example, phenylbutazone in cattle of any age (UK) or cattle >20 months of age (USA) (Davis et al., 2009). In this chapter, information on the pharmacological effects of various agents and their combinations is given in order that informed decisions can be made about their use in all situations. Some of the anaesthetic agents mentioned may be unsuitable in some countries for use in animals for subsequent slaughter.
Knowledge of withdrawal times is important to avoid anaesthetic agent residues in animals and animal products intended for human consumption.
Agents used in bovine species
Xylazine
Xylazine has been used as a sedative in cattle for a long time. The dose rate of xylazine in cattle, 0.02–0.20 mg/kg, with the highest dose intended for IM use, is one-tenth of that used in horses (Table 12.1). Intravenous injection results in deeper sedation than IM administration. Cattle may assume recumbency even at the lowest dose rates, although breed differences in sensitivity to xylazine have been reported. In an investigation comparing Hereford and Holstein cattle, 84% of Herefords spontaneously lay down after xylazine administration, whereas only 22% of Holsteins did so (Raptopoulos & Weaver, 1984). Furthermore, the average duration of recumbency in the Herefords was 90 min compared with 50 min in the Holsteins. Thus, in some situations, the dose rate in Holsteins may be increased to 0.3 mg/kg. Even higher doses may be necessary for free-ranging cows to be immobilized using a dart gun in order to overcome the high circulating catecholamine concentrations induced by excitement. The environmental conditions under which xylazine is administered may influence the response. In a study comparing the effects of xylazine in Holstein heifers under a thermoneutral condition compared with a hot environment (temperature approximately 33°C and relative humidity 63%), the time to standing was increased from 41 min to an average of 107 min during heat stress (Fayed et al., 1989).
Xylazine may cause mild to severe decreases in PaO2 and moderate increases in PaCO2 in mature cattle (DeMoor & Desmet, 1971; Raptopoulos & Weaver, 1984). A more detailed discussion of the effects of α2-agonists on pulmonary function is in the following chapter. Xylazine administration induces bradycardia, decreased MAP and CO, and increased peripheral resistance (Campbell et al., 1979, Rioja et al., 2008). Campbell et al. (1979) also noted second degree atrioventricular heart block in one out of five calves receiving xylazine.
Xylazine has a number of side effects that may have an adverse effect on the animal. It abolishes the swallowing reflex so that regurgitation can result in pulmonary aspiration. The inability of the cow or bull to withdraw its tongue into its mouth and swallow may persist until after the animal regains the standing position. Thus, it is advisable to withhold food and water from cattle before giving xylazine. Gastrointestinal motility is decreased and bloat may develop, while diarrhoea may be observed 12–24 hours after sedation (Hopkins, 1972). Hyperglycaemia may persist for about 10 hours. Increased urine production occurs within 30 minutes of administration and continues for 2 hours (Thurmon et al., 1978). Use of xylazine in animals with urethral obstruction may be responsible for rupture of the urinary bladder or urethra. Xylazine causes contraction of the bovine uterus similar to oxytocin (LeBlanc et al., 1984), and premature birth has been reported after administration to heavily pregnant cows. Administration of xylazine, 0.04 mg/kg IV, to pregnant cows between 219 and 241 days of gestation was followed by a decrease in uterine arterial blood flow by 56% from baseline values associated with an initial 59% reduction in O2 delivery (Hodgson et al., 2002). The impact on uterine blood flow progressively lessened but was still significant at 45 minutes. Consequently, use of xylazine in pregnant cows in the last trimester of pregnancy is not recommended.
Minor surgical procedures have been performed on cattle sedated with xylazine but local infiltration techniques or nerve blocks should be utilized to ensure sufficient analgesia.
Detomidine
In contrast to xylazine, the dose rates for detomidine in cattle are similar to those used in horses. In Europe, detomidine is licensed for cattle at doses of 0.01–0.04 mg/kg IM or IV, with (at the time of writing) withdrawal times of 12 hours for milk and 2 days for meat. At the higher doses, cattle may lie down. One study (Lin & Riddell, 2003) documented that detomidine, 0.01 mg/kg, IV in Holstein cattle resulted in greater sedation than xylazine, 0.02 mg/kg, during which the cows remained standing. Elimination is mainly by metabolism as there is negligible excretion of the drug in urine. No detomidine was detectable in milk 23 h after dosing and tissue concentrations measured 48 h after dosing were less than 3% of the original dose (Salonen et al., 1989). Detomidine, 0.04 and 0.06 mg/kg, increases electrical activity of the bovine uterus (Vainio, 1988). A lower dose rate of detomidine, 0.02 mg/kg, decreased electrical activity of the uterus and sedation at this dose rate may be safe in the pregnant animal (Vainio, 1988).
The pharmacological effects of detomidine in cattle are very similar to those of xylazine in that it causes bradycardia, hyperglycaemia, and increased urine production. An exception is that detomidine causes a transient increase in blood pressure that is dose dependent in duration, whereas xylazine decreases blood pressure in cattle.
Medetomidine
Deep sedation without recumbency can be obtained with intravenous doses of medetomidine, 0.005 mg/kg, while 0.01 mg/kg produces recumbency and sedation equivalent to that obtained with intravenous doses of 0.1–0.2 mg/kg xylazine (England GCW & Clarke KW, unpublished observations). Duration of sedation of medetomidine, 0.03 mg/kg, IV in calves lasted significantly longer than xylazine, 0.3 mg/kg IV with the mean times to sternal recumbency and to standing after agent administration being 66 and 242 minutes, and 19 and 128 minutes, respectively (Rioja et al., 2008). In a separate experiment, with calves suspended in the upright position, injection of medetomidine, 0.03 mg/kg, IV resulted in hypoxaemia and hypercarbia at 5 and 15 minutes, significant decreases in heart rates and CO, and increases in MAP, systemic vascular resistance, and central venous pressure. Administration of atipamezole, 0.1 mg/kg, IV 20 minutes after medetomidine satisfactorily reversed the sedation and the respiratory and cardiovascular effects, including hypoxaemia. In contrast, atipamezole was not effective in reversing hypoxaemia in sheep when given more than 5 minutes after medetomidine administration. Medetomidine, 0.015 mg/kg, has been administered by epidural injection for analgesia in cows (Lin et al., 1998).
Antagonists of α2-agonists
As prolonged recumbency causes so many problems in cattle, the availability of α2-agonist antagonists is of particular value. These antagonists not only cause the animal to awaken, they also antagonize the majority of the side effects of the agonists, including restoring ruminal motility to normal and allowing release of gas from the rumen.
Almost all the α2-agonist antagonists have been used in cattle sedated with xylazine. Yohimbine, 0.125 mg/kg, with aminopyridine, 0.3 mg/kg, will awaken cattle sedated with 0.2–0.3 mg/kg of xylazine, but will not restore a normal state of consciousness. Tolazoline, 0.5 mg/kg, was documented to be superior to yohimbine for antagonizing xylazine sedation in cattle (Hikasa et al., 1988). Tolazoline at 0.2 mg/kg reverses the suppression of ruminal motility induced by xylazine but higher doses, 0.5–2.0 mg/kg, are required for full reversal of sedation. Xylazine sedation, 0.3 mg/kg, IM in 6-month old Holstein Friesian calves was rapidly reversed within minutes by IV injection of tolazoline 1 and 2 mg/kg, with a more rapid return to normal gait following the larger dose rate (Powell et al., 1998). Care must be taken to administer tolazoline slowly as there are anecdotal reports that intravenous injection has been associated with abrupt adverse haemodynamic changes. In one study, reversal of xylazine sedation in calves with tolazoline caused transient sinus bradycardia and sinus arrest, accompanied by severe systemic arterial hypotension (Lewis et al., 1999). Withholding times are increased after administration of tolazoline. Xylazine is below the limits of detection in meat and organs by 72 hours and in milk by 12 hours whereas the limit of detection after tolazoline administration was reached in 96 hours for meat and 48 hours for milk, leading to a recommended withholding time (in New Zealand) for tolazoline of 7 days for cattle meat and offal (Delehant et al., 2003).
Atipamezole has been used to reverse sedation induced by administration of α2-agonist sedatives in cattle with a wide range of dose rates, 0.025–0.2 mg/kg. Atipamezole, 0.025, 0.03, 0.04, and 0.05 mg/kg (25–50 µg/kg), has been used to reverse the effects of xylazine, 0.2–0.3 mg/kg (Thompson et al., 1991; Rioja et al., 2008). Atipamezole, 0.06–0.08 mg/kg, IV effectively reversed within 2 minutes free-ranging cattle immobilized by darting with xylazine, 0.55 mg/kg, or medetomidine, 0.04 mg/kg (Arnemo & Søli, 1993). Of interest, eight out of 26 cows administered high doses of xylazine or medetomidine were in the last 2 months of pregnancy and were observed subsequently to calve normally. Atipamezole has been used at different dose rates to reverse the effects of medetomidine in cattle and calves. In one study (Raekallio et al., 1991), atipamezole, 0.06 mg/kg, given either IV or half IV and half IM to antagonize medetomidine in calves resulted in a rapid smooth recovery to ambulation and, in another (Rioja et al., 2008), atipamezole, 0.1 mg/kg, IV had the same effect. Ranheim et al. (1999) reversed sedation in calves given medetomidine, 0.04 mg/kg, by injecting atipamezole, 0.2 mg/kg, however, the authors’ recommendation was that this dose should not be exceeded. Time of administration of atipamezole to standing has been reported from 2 to 12 minutes. Relapse to sedation or recumbency at times varying from 80 minutes to 2 to 3–4 hours after administration of atipamezole have been reported (Arnemo & Søli, 1993; Ranheim et al., 1998, 1999). Atipamezole given to unsedated cattle, or as sedation is waning, may induce a state of excitement, hyperactivity, kicking and bucking.
Acepromazine
Acepromazine may be given in doses of 0.02–0.05 mg/kg IV or 0.1 mg/kg IM to induce mild tranquillization. Acepromazine will decrease the dose rate of subsequently administered anaesthetics and may increase the risk of regurgitation.
Chloral hydrate
Chloral hydrate has been used as a sedative for adult cattle administered orally or by IV injection. To obtain a recumbent, very lightly unconscious adult cow, the drug can be administered by drench or by stomach tube in doses of 30–60 g as a 1 in 20 solution in water. Sedation attains its maximum depth in 10–20 minutes and local analgesics may be injected for nerve blocks while sedation is developing. The degree of sedation is not predictable in an excited animal. Withholding of water to increase thirst may act as an inducement for the animal to drink medicated water. Intravenous injection of chloral hydrate can be given with the cow standing or after casting with ropes. The dose required is between 80 and 90 mg/kg of a 10% solution and the infusion should be stopped before reaching the desired level of sedation because narcosis will continue to deepen after the IV injection is completed. The induction and recovery periods are excitement free. Chloral hydrate should be used cautiously in debilitated cows as rapid IV administration can cause cardiovascular collapse.
Sedative–opioid combinations
Administration of butorphanol to healthy unsedated cows will not predictably induce sedation and may induce behaviour changes including restlessness and bellowing. Butorphanol may provide sedation in cattle that are sick or in pain and may increase the quality of sedation when administered in conjunction with xylazine or detomidine. Reports of use of butorphanol with xylazine in healthy cattle have conflicting results on the degree of contribution of butorphanol to analgesia. Comparisons in adult cows between xylazine, 0.02 mg/kg, detomidine, 0.01 mg/kg, with or without butorphanol, 0.05 mg/kg, all administered IV, revealed no differences in duration or degree of sedation induced by the addition of butorphanol, and that the greatest sedation was observed after detomidine alone (Lin & Riddell, 2003). Sedation was accompanied by impaired swallowing indicated by drooling saliva and, in some cows, lowering of the head; all cows remained standing. A combination of xylazine, 0.02–0.05 mg/kg, butorphanol, 0.025 mg/kg, and ketamine, 0.1–0.4 mg/kg, IM has been used to restrain cattle with the low doses administered for standing restraint and the higher doses when recumbency is desired (Anderson, 2008). The duration of effect is about 45 minutes and tolazoline, 2 mg/kg, IM was recommended when reversal of xylazine was desired. Butorphanol can be detected in the milk for up to 36 hours following administration (Court et al., 1992).
Minimal information is available on the use of µ opioids in cattle. A thermal nociceptive device applying heat to the coronary band of the forefoot of cattle was devised to test multiple IV doses of morphine, 0.1–0.4 mg/kg (Muchado et al., 1998). No conclusion was reached about the analgesic effect except that individual variation was noted and no increased locomotion was observed.
Non-steroidal anti-inflammatory drugs (NSAIDs)
The beneficial effects of NSAIDs in ameliorating signs of post-surgical pain in cattle have been well documented, for example, use of ketoprofen, meloxicam, flunixin, and carprofen have been studied in large groups of calves subjected to castration or dehorning. Not all NSAIDs are licensed for use in cattle in all countries and the veterinarian must adhere to local laws. Use of phenylbutazone in cattle is prohibited.
Local analgesia
A number of local anaesthetic agents (see Chapter 4), with or without the addition of epinephrine, may be used, the choice being influenced by desired duration of action, irritancy, volume, and cost. Lidocaine and mepivacaine are considered to be agents of intermediate duration and bupivacaine and ropivacaine as longer acting. Lidocaine is not approved for use in animals, other than horses, intended for human consumption in EU member countries; in the USA the recommended meat and milk withdrawal time after administration of lidocaine to cattle is 24 hours or one day. However, in adult Holstein cows injected with 100 mL 2% lidocaine (approximately 3.5 mg/kg) in a flank inverted L-block pattern, the last measurable time of lidocaine detection in milk was 32.5 ± 16.2 hours with a mean concentration of 46 ± 30 ng/mL (Sellers et al., 2009). Three of nine cows showed residues at 48 hours with no detectable residues at 60 hours. The authors calculated withdrawal time for meat for safety at 10 times the t½b, as 4 days and for milk as 3 days for this dose of lidocaine. No lidocaine was detected in serum or milk after caudal epidural administration of lidocaine, 2.2 mg/kg.
Complications following local analgesia include systemic toxicity, infection if aseptic precautions are not followed, and neurological injury. Accidental intravenous or intra-arterial injection should be avoided by syringe aspiration before injection of a local anaesthetic. Recommendations of maximum doses of local anaesthetic have limited evidence base in cattle but clearly depend on the site, volume and speed of administration, and uptake into the systemic circulation. The recommended limit of 6 mg/kg for lidocaine for local infiltration may be valid as obvious sedation and recumbency may be observed when a larger dose has been administered, for example, by repeating paravertebral nerve blocks following initial failure. Cardiovascular collapse will occur when a tourniquet dislodges soon after IV injection of a smaller dose of lidocaine for intravenous regional analgesia. Metabolism of ester-linked local anaesthetics, such as procaine, begins in the blood before the distribution phase and therefore relatively higher doses may be used. The site of injection significantly influences the plasma concentrations, and a hyperdynamic circulation and local vasodilation increase speed of uptake. Epinephrine (1 : 200 000) may be added to local anaesthetics to cause local vasoconstriction and reduce the peak plasma concentrations, such that in many countries the maximum official recommended doses of local anaesthetics in human patients are higher when epinephrine is added (Rosenberg et al., 2004). Rarely, tissue and skin necrosis has been observed in cattle following local infiltration of tissues with lidocaine with epinephrine. Epinephrine included with lidocaine or mepivacaine prolongs the duration of epidural block in the more caudal segments of the spinal cord, but is not of added benefit to the duration of bupivacaine or ropivacaine (Bernards, 2006). The mechanism by which epinephrine increases the duration of epidural lidocaine is not clear but is thought to be partly due to dural vasoconstriction, without an effect on spinal cord blood flow, slowing lidocaine elimination and partly due to an inhibitory effect (hyperpolarization) of sensory and motor neurons.
Neurological injury is rare but might occur following direct trauma from the needle or from introduction of bacteria into the perineural space. Case reports and studies of human patients developing persistent paraesthesias and motor weakness following spinal anaesthesia have occurred more commonly with lidocaine than with other anaesthetics. Lidocaine is known to be neurotoxic and nerve injury after intrathecal or epidural injection may result from pooling of undiluted lidocaine resulting in a prolonged neural contact. Maldistribution of lidocaine after injection may be observed in the patient by detection of failed or uneven analgesia. Efforts at redistributing the lidocaine by altering patient position rather than repeating local anaesthetic administration have been suggested to decrease the risk of neural toxicity (Bernards, 2006). Adjuncts to local anaesthetics may contribute to nerve damage but evidence is conflicting. Addition of epinephrine to preservative-free lidocaine delivered intrathecally (subarachnoid) in rats increased the extent of sensory block over lidocaine alone (Hashimoto et al., 2001). Sensation in the rats’ tails was decreased at 4 and 7 days after injection of lidocaine or lidocaine–epinephrine. Subsequent histological examination revealed nerve injury in the rats that received lidocaine that was significantly worse in rats given lidocaine–epinephrine compared with no functional or morphological effects of epinephrine alone. These data raise concern for adverse effects from intrathecal use of lidocaine with epinephrine. In an in vitro model of lidocaine-induced toxicity on neuronal cells and astrocytes, epinephrine did not influence the neurotoxicity of lidocaine indicating that epinephrine may act through its vasoconstrictive effects in vivo and not by a direct toxic effect (Werdehausen et al., 2011). However, this study evaluated several commonly used adjuncts and determined that the addition of ketamine to lidocaine increased cell toxicity in an additive manner and addition of midazolam increased toxicity to a lesser extent. The mechanism of toxicity of lidocaine and ketamine is the same, namely activation of the mitochondrial pathway of apoptosis (a programmed cell death process).
The techniques described below will describe doses of lidocaine as this, with or without epinephrine, is the agent most commonly employed in cattle. However, for various legislative reasons (see Chapter 1), procaine is still often used in Europe and, although requiring a slightly longer time for onset of action, appears to be equally satisfactory as lidocaine for procedures where the addition of epinephrine is acceptable.
Most clinical surgery in cattle is carried out under local anaesthesia with or without sedation, frequently in the standing animal.
Techniques
Auriculopalpebral nerve block
The auriculopalpebral nerve is a branch of the facial nerve (VIIth cranial nerve) supplying motor fibres to the orbicularis oculi muscle. The nerve runs from the base of the ear along the facial crest, past and ventral to the eye, giving off its branches on the way. This block is used to prevent eyelid closure during examination or surgery of the eye. It does not provide analgesia to the eye or eyelids and should be used in conjunction with topical analgesia or other nerve blocks for painful procedures.
The needle is inserted in front of the base of the ear at the end of the zygomatic arch until its point lies at the dorsal border of the arch (Fig. 12.2). About 10 mL of 2% lidocaine is injected beneath the fascia at this point.
Cornual nerve block
The horn corium and the skin around its base derive their sensory nerve supply from a branch of the zygomaticotemporal branch of the Vth cranial nerve. The nerve emerges from the orbit and ascends just behind the lateral ridge of the frontal bone (Fig. 12.3). This latter structure can be readily palpated with the fingers. In the upper third of the ridge, the nerve is relatively superficial, being covered only by skin and a thin layer of the frontalis muscle.
The legislation relating to dehorning and disbudding of calves differs between countries, for example, in the UK and Sweden, disbudding is not permitted without the use of local anaesthesia ± sedation, whereas in North America, local anaesthesia is not always provided to all calves for dehorning; with the conundrum that fewer calves <6 months of age receive analgesia than calves >6 months of age and beef calves are less likely to receive analgesia than dairy calves (Stafford & Mellor, 2005; Hewson et al., 2007; Fajt et al., 2011). In one survey, cattle producers were less likely than veterinary practitioners to include analgesia when dehorning their calves, indicating a need for client education (Misch et al., 2007).
In the last 15 years, the impact of dehorning and disbudding on cattle has been investigated extensively (Stafford & Mellor, 2005). Local anaesthesia significantly attenuates cortisol response during and immediately after the procedure. Cortisol levels increase some hours after the effects of local anaesthesia have dissipated and, although the plasma cortisol concentrations decrease by 9 hours after dehorning, the calves graze and ruminate less between 24 and 48 hours after dehorning than they do between 48 and 72 hours or compared with animals that were not dehorned. Extension of the duration of analgesia by following the lidocaine injection with bupivacaine may eliminate the cortisol response (delay onset of pain). Administration of an NSAID (e.g. ketoprofen or meloxicam, but not phenylbutazone) with a lidocaine nerve block was documented to provide more effective analgesia and continue pain relief beyond the 2–3 hours provided by lidocaine (Stafford & Mellor, 2005; Stewart et al., 2009; Duffield et al., 2010). Xylazine alone was not effective in eliminating the cortisol response to dehorning.
Petersen eye block
A 22 gauge, 2.5 cm needle is used to infiltrate local analgesic solution subcutaneously, about 5 mL of 2% lidocaine, within the notch formed by the joining of the zygomatic process of the frontal bone and the frontal process of the zygomatic bone cranially, the zygomatic arch ventrally and the coronoid process of the mandible caudally (Fig. 12.4). A 12 or 14 gauge, 2.5 cm needle placed as far rostral and ventral as possible in the desensitized skin of the notch serves as a cannula and an 18 gauge, 10 or 12 cm needle is introduced through it. The long needle is directed in a horizontal and caudal direction until it strikes the coronoid process of the mandible. It is then redirected into the orbit just rostral to the orbitorotundum foramen at a depth of about 8–10 cm from the skin and 10–15 mL of 2% lidocaine solution injected. This blocks the oculomotor, trochlear, abducens nerves and two branches of the trigeminal nerve as they emerge from the foramen orbitorotundum. The needle is withdrawn to the subcutaneous tissue and redirected caudally and laterally to block the zygomatic branch of the auriculopalpebral nerve on the zygomatic arch by the injection of 5–10 mL of the local analgesic solution. The Petersen technique requires more skill to perform than a retrobulbar block but it may be safer.
Peribulbar and retrobulbar block
Retrobulbar injection of the eye is achieved by introduction of a curved needle through the skin about 1 cm lateral to the lateral canthus, or through the conjunctiva. The needle is first directed straight back and away from the eyeball until the point is beyond the globe and then turned inward to penetrate the muscle cone. When no blood is obtained after aspiration, lidocaine is deposited behind the eye.
Peribulbar anaesthesia is produced by inserting the needle in 2 to 4 quadrants of the eye and injecting lidocaine within the orbit but outside the ocular muscles. Injection of a total of 20–30 mL of 2% lidocaine (or its equivalent) for an adult cow or bull will produce corneal analgesia, mydriasis, and proptosis and paralysis of the eyeball. Anaesthesia is produced after spread of the anaesthetic agent; thus a larger volume of lidocaine is required for peribulbar anaesthesia, the onset of block is longer, and the larger volume of solution causes a greater increase in ocular pressure.
Recommendations vary over the use of sharp hypodermic or blunt (e.g. spinal needle) needles for ocular blocks, but penetration of the globe has been reported in human patients with both sharp and blunt needles (Hay, 1991; Wong, 1993). Visual outcome is not a factor when penetration of the globe occurs during nerve block for enucleation. However, bacterial contamination of the orbit is possible.
Potentially adverse effects of both the Petersen block and the retrobulbar block include bradycardia, hypotension, asystole, respiratory depression, apnoea, perforation of the globe and intraorbital or retrobulbar haemorrhage. Symptoms of local anaesthetic spread to the central nervous system vary but respiratory arrest is a usual sign of brainstem anaesthesia. When the block is used for other purposes, care must be taken to ensure that the corneal surface does not become dry because of loss of tear formation for several hours.
Inverted L block
Infiltration of the skin, subcutaneous and deeper tissues in an inverted L shape with 60 mL of 2% lidocaine solution is a commonly used technique to provide analgesia for a flank laparotomy in a standing cow (Fig. 12.5). It is also used in recumbent animals to block the site for a paramedian incision, or repeated in a mirror image to form an inverted U shape and analgesia for a midline incision. Injections must be made both subcutaneously and down to the peritoneum to produce a total block. The block can be achieved by making isolated injections at intervals of about 1 cm, which relies on lateral diffusion of the analgesic solution. Alternatively, a wall of local analgesic solution can be created by inserting a long needle to its depth, and injecting anaesthetic solution in a steady stream as the needle is withdrawn.
Paravertebral nerve block
Paravertebral block involves the perineural injection of local analgesic solution about the spinal nerves as they emerge from the vertebral canal through the intervertebral foramina. This technique is commonly used to provide analgesia for laparotomy. It offers a major advantage over use of local infiltration in that the abdominal wall including the peritoneum is more likely to be uniformly desensitized. Additionally, the abdominal wall is relaxed.
The area of the flank bounded cranially by the last rib, caudally by the angle of the ilium and dorsally by the lumbar transverse processes, is innervated by the thirteenth thoracic and first and second lumbar nerves. The third lumbar nerve, although it does not supply the flank, gives off a cutaneous branch that passes obliquely backwards in front of the ilium. Operations involving the abdominal ventral midline will require additional desensitization of the dorsal nerves cranial to the thirteenth. The last thoracic and first lumbar intervertebral foramina in cattle are occasionally double. The last thoracic foramen lies immediately caudal to the head of the last rib and on a level with the base of the transverse process of the first lumbar vertebra (Fig. 12.6). The lumbar foramina are large and are situated between the base of the transverse processes and approximately on the same level. The spinal nerves, after emerging from the foramina, immediately divide into a smaller dorsal and a larger ventral branch. The dorsal branch supplies chiefly the skin and muscles of the loins, but some of its cutaneous branches pass a considerable distance down the flank. The ventral branch passes obliquely ventrally and caudally between the muscles and comprises the main nerve supply to the skin, muscles, and peritoneum of the flank. The ventral branch is also connected with the sympathetic system by a ramus communicans. Paralysis of the nerves at their points of emergence from the intervertebral foramina will provoke desensitization of the whole depth of the flank wall and complete muscular relaxation. Block of the rami communicantes will result in splanchnic vasodilation and potential for hypotension.
Figure 12.6 Location of the last two thoracic and first three lumbar segmental nerves. The needle indicates position for paravertebral injection of local anaesthetic to block T13.
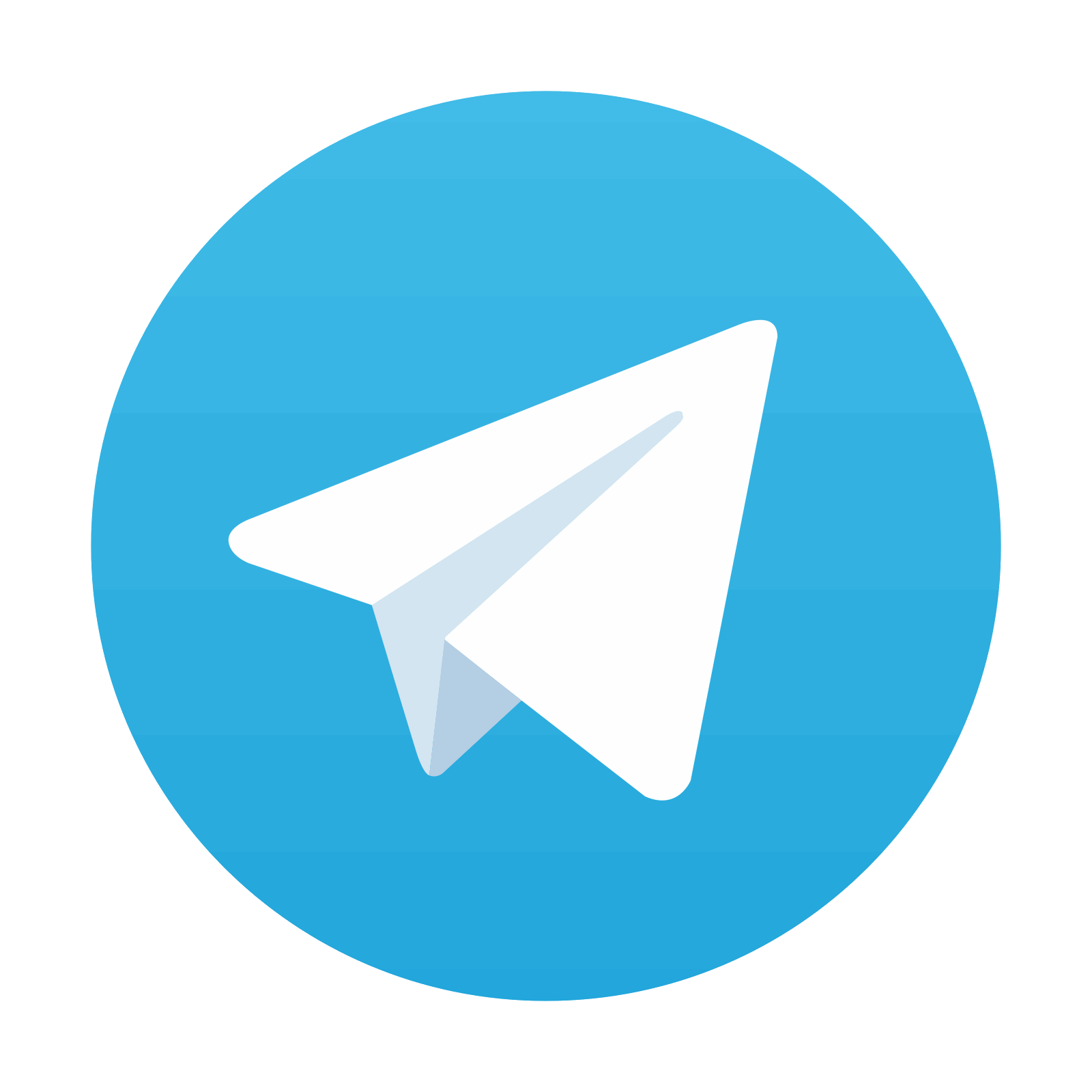
Stay updated, free articles. Join our Telegram channel

Full access? Get Clinical Tree
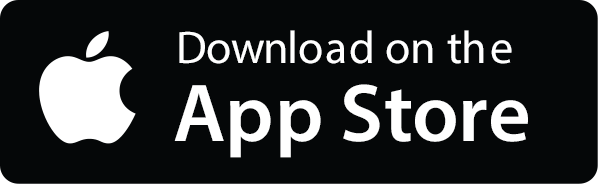
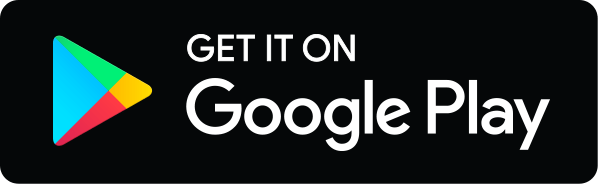
