Chapter 2
Patient monitoring and clinical measurement
Left atrial pressure (pulmonary artery wedge pressure)
Rate monitors and apnoea alarms
Tidal and minute volume monitors
Blood gas and acid base values
Introduction
From the earliest days of anaesthesia, the anaesthetist has monitored the patient’s pulse rate, pattern of breathing and general condition. Advances in electronic technology have made reasonably reliable, easily attached, non-invasive monitoring devices available for clinical practice. Observations and measurements of certain parameters before, during, and after anaesthesia provide important data to support the clinical assessment of the animal’s condition and improve the chances of survival of the very ill by indicating what treatment is needed, as well as the response to treatment already given.
It is necessary to know what to measure as well as how to measure it and not all anaesthetists may agree on the priority ranking of the monitoring devices available. However, for major surgery, for anaesthesia and surgery of poor-risk patients, and for equine anaesthesia, it would be difficult to defend the failure to use monitoring equipment, especially if it were available. Recommendations for monitoring of anaesthetized patients are available on the web sites for the Association of Veterinary Anaesthetists, American College of Veterinary Anesthesia and Analgesia, and the American Animal Hospital Association.
General considerations relating to monitoring
Complications, including death, may occur in healthy patients at all stages of anaesthesia and monitors provide early warning of life-threatening developments. Anaesthetic mishaps may be caused by mechanical malfunction, disconnection of equipment, or human error. Judgemental error frequently occurs when the anaesthetist is in a hurry and circumvents basic practices and procedures, or when a decision must be made in an emergency. The prevalence of complications may also be associated with inadequate training or experience of the anaesthetist. Knowledge and experience are a function of the nature of the training received and the years of practice, but proper vigilance at all times can only be generated by self-motivation.
Routines should be developed to ensure that each aspect of apparatus function is checked before use. Failure to follow a simple checklist in every case features high on the list of causes of anaesthetic disasters. All anaesthetic equipment, including monitoring devices, should be maintained in good functioning order. It should be a matter of course to maintain monitors with a battery back up fully charged in case of need in an area without a convenient electricity outlet nearby, failure of electricity supply, or the need to disconnect from the main supply to minimize electrical interference with other monitoring equipment.
Proficiency with methods of electronic surveillance must be acquired during minor procedures so that they can be applied properly in circumstances where their use is mandatory (e.g. during major surgery or a cardiovascular crisis). Routine use ensures that probes, sensors, electrodes, etc. can be applied quickly to the animal and increases the likelihood that the information obtained is reliable.
Although current practice is to establish monitoring only after the animal has been anaesthetized, it must be recognized that many complications occur during induction of anaesthesia. Ideally, especially for poor-risk patients, monitoring should begin when the drugs for premedication are administered. Dogs and cats may vomit after administration of an opioid and the quantity and content of the vomit may warn that the animal was fed recently and so may be at risk for regurgitation and pulmonary aspiration of gastric material. Brachycephalic breeds and animals with respiratory problems should always be observed after administration of preanaesthetic drugs because sedation may cause partial or complete airway obstruction or serious respiratory depression and hypoxaemia. In any animal, evaluation of the degree of sedation produced by premedicant drugs may indicate that the anaesthetic plan should be reassessed and either drug doses reduced or additional agents included.
Careful observation of the patient during induction of anaesthesia may allow precise titration of drugs to achieve the desired depth of anaesthesia and ensure early recognition of a complication that requires immediate specific treatment, such as cyanosis, anaphylaxis, or cardiac arrest. Where possible, patients at risk for complications may be attached to specific monitoring equipment before induction of anaesthesia. Appropriate equipment for this would be the electrocardiograph (ECG), a device for measurement of blood pressure, or a pulse oximeter.
Recording drugs, dosages and responses for each patient is essential and provides valuable information for any subsequent time that anaesthesia may be needed. Noting all measurements on an anaesthetic record provides a pictorial description of changes that can be used to predict complications and plan treatment (Fig. 2.1). Retrospective evaluation of difficult cases and of series of records, perhaps of patients with similar surgical procedures, or to compare different anaesthetic protocols, can be used to monitor the anaesthetist’s performance and identify difficult situations that require further thought and improved management. For research purposes, data can be acquired into a computer for accurate data summaries.
Figure 2.1 Anaesthetic record of a 61 kg, 6-year-old female Great Dane anaesthetized for exploratory laparotomy because of torsion of the spleen. Anaesthesia was induced by intravenous administration of ketamine, 200 mg, and diazepam, 10 mg, and maintained with isoflurane. Heart rate, blood pressure and respiratory rate were recorded at regular intervals to facilitate early recognition of adverse trends.
Monitoring animals during anaesthesia must include observation of behaviour and reflexes and measurement of various physiological parameters at regular intervals to accomplish two objectives. The first objective is to ensure that the animal survives anaesthesia and surgery. The second objective is to obtain information that can be used to adjust anaesthetic administration and management to minimize physiological abnormalities, which is especially important for animals that have already compromised organ systems. The goal is to prevent development of preventable adverse consequences 1 hour, 12 hours, or even 3 days after anaesthesia.
Monitoring should continue into the recovery period to determine the need for additional analgesic drugs, adequacy of ventilation, and to record serious deviations in body temperature. Mucous membrane colour should be checked for at least 20 minutes after the animal has been disconnected from oxygen as it may take that long for hypoxaemia to develop in animals that are moderately hypoventilating and breathing air.
A variety of methods using inexpensive or expensive equipment can be used to monitor parameters determined by the species of animal to be anaesthetized and by the abnormalities already present in the patient. Not all monitoring techniques need to be applied to every patient. A recommendation for three levels of monitoring is presented in Table 2.1; level 1 monitoring information should be obtained from all anaesthetized animals, level 2 monitors are affordable and recommended for routine use in some groups of patients, and level 3 monitors individually offer improved monitoring for patients with specific problems.
This chapter will describe the techniques of monitoring using a systems approach, and offer guidelines for interpretation of the information obtained. Further recommendations are given in the chapters devoted to species anaesthesia and the chapter on management of complications.
Clinical assessment of the patient
Monitoring the central nervous system
Monitoring the depth of anaesthesia is not easy (see Chapter 1). An early attempt at definition through observation of changes in reflexes, muscle tone, and respiration with administration of increased concentration of ether resulted in classification of anaesthesia into four stages (Fig. 2.2). The animal was said to make the transition from consciousness to deep anaesthesia by passing sequentially through Stage I (in which voluntary excitement might be observed), Stage II (when the animal appeared to be unconscious but exhibited involuntary muscle movement, such as limb paddling, and vocalization), Stage III (surgical anaesthesia), and Stage IV (anaesthetic overdose immediately prior to death). Stage III was further divided into Plane 1 (light anaesthesia sufficient only for non-painful procedures), Plane 2 (medium depth anaesthesia employed for most surgical procedures), Plane 3 (deep anaesthesia), and Plane 4 (excessively deep anaesthesia). Although the progression of changes described in Fig. 2.2 are generally accurate representations of the transition from light to deep anaesthesia, the rate of changes are altered by concurrent administration of injectable drugs. Thus, in clinical practice, depth of anaesthesia is judged by a number of methods, including altered somatic and autonomic reflexes in response to nociceptive stimulation and by knowledge of the amount of anaesthetic and analgesic agents administered.
Figure 2.2 Changes in ventilation and eye signs follow recognized patterns with different stages of inhalation anaesthesia. The progression of these changes will be influenced by inclusion of injectable anaesthetic agents. Adapted from Soma, 1971.
Eye position and reflexes
Eye movements are similar with thiopental, propofol, etomidate, alfaxalone, and inhalant anaesthetics in that the eyeball rotates rostroventrally during light and moderate depths of surgical anaesthesia, returning to a central position during deep anaesthesia (Fig. 2.3). Muscle tone is retained during ketamine anaesthesia and the eye remains centrally placed in the orbit in dogs and cats (Fig. 2.4), and only slightly rotated in horses and ruminants. Fine nystagmus may be present in horses anaesthetized with ketamine (see Chapter 11). The palpebral reflex, which is partial or complete closure of the eyelids (a blink) elicited by a gentle tap at the lateral canthus of the eye or gentle stroking of the eyelashes, is frequently a useful guide to depth of anaesthesia. At a plane of anaesthesia satisfactory for surgery, the palpebral reflex is present but weak and nystagmus is absent. A brisk palpebral reflex develops when anaesthesia lightens. Ketamine anaesthesia is associated with a brisk palpebral reflex.
Figure 2.3 The rostroventral rotation of the eye in this dog is consistent with light and medium planes of isoflurane or sevoflurane anaesthesia.
Figure 2.4 The central position of the eye, with a brisk palpebral reflex and pupillary dilation, is observed typically during ketamine anaesthesia in cats.
A corneal reflex is a similar lid response elicited by gentle pressure on the cornea. The presence of a corneal reflex is no indicator of depth of anaesthesia and may still be present for a short time after cardiac arrest has occurred.
The pedal reflex is frequently tested in dogs, cats and small laboratory animals to determine if depth of anaesthesia is adequate for the start of surgery. Pinching the web between the toes or firm pressure applied to a nail bed will be followed by withdrawal of the limb if analgesia is inadequate. Other species-specific reflexes that have been found useful include the whisker reflex in cats, where pinching of the pinna elicits a twitch of the whiskers, and tickling the inside of a pig’s ear, where a shake of the head indicates inadequate anaesthesia for surgery. It must be remembered that administration of neuromuscular blocking agents blocks somatic reflexes and respiratory responses, and that the eye position will be central.
Increasing the depth of anaesthesia by increasing administration of an anaesthetic agent in most cases produces increased respiratory and cardiovascular depression. However, more than one anaesthetic or preanaesthetic agent is generally administered and the cardiopulmonary effects are determined by the combination of agents used and their dose rates. Thus measurements of respiratory rates, heart rates, and blood pressure are not reliable guides to depth of anaesthesia. It is not uncommon for an unstimulated dog or horse anaesthetized with an inhalation agent to have a low arterial blood pressure and yet in the next minute start moving its legs in response to a skin incision, all accompanied by a dramatic increase in blood pressure. Nevertheless, significant increases in heart rate and/or arterial blood pressure in response to surgical stimulation are an indication of inadequate analgesia. In certain circumstances, surgical pain can induce a vaso-vagal response resulting in bradycardia, decreased peripheral perfusion and pale mucous membranes. An example is traction on the ovarian ligament during ovariohysterectomy in a bitch. Preanaesthetic administration of an anticholinergic may prevent bradycardia but may also obscure heart rate responses to painful stimuli.
Anaesthetic gas analysers
The anaesthetic gas analyser measures the concentration of inhalation anaesthetic agent in inspired and expired gases (Fig. 2.5). The gases are sampled at the junction of the endotracheal tube and breathing circuit by continuous aspiration of gases at a rate of 150 mL/min to a monitor placed at some distance from the patient. To assess the depth of anaesthesia, the end-tidal concentration of inhalation agent is measured (alveolar concentration is measured at the end of exhalation) and compared with the Minimum Alveolar Concentration (MAC) value for that inhalant anaesthetic and species (Table 2.2). Using MAC for monitoring depth of anaesthesia is not precise as MAC is only an approximation of the predicted response in 50% of a population and MAC is decreased in very young and in old patients. Higher than MAC values will be required to prevent movement in all patients, and to block autonomic responses and increases in cortical electrical activity in response to a painful stimulus (March & Muir, 2003a), usually 1.2–1.5 times MAC, when anaesthesia is maintained almost entirely by inhalation agent. Less than MAC value may be sufficient when the patient is receiving continuous or intermittent administration of an adjunct agent(s) such as an opioid, lidocaine, ketamine, propofol, or medetomidine/dexmedetomidine. For these animals, the anaesthetic administration must be adjusted according to observation of reflexes and lack of significant cardiovascular response to surgical stimulus.
Figure 2.5 This gas analyser (Capnomac UltimaTM, Datex-Engstrom Inc., Tewksbury, Maryland, USA) is monitoring a 27 kg female English Bulldog that was premedicated with glycopyrrolate and butorphanol and anaesthesia induced with propofol. She has been breathing oxygen and isoflurane at a vaporizer setting of 2.5% for 5 minutes. The monitor indicates that the inspired (Fi) isoflurane concentration is less than the vaporizer setting and that the end-tidal (ET) isoflurane concentration is less than MAC value.
Table 2.2
Approximate MAC* values for isoflurane and sevoflurane in several species
* MAC: minimum alveolar concentration of anaesthetic agent required to prevent purposeful movement in 50% of animals in response to a standard painful stimulus; see species chapters for ranges of values.
It should be noted that the gas analyser also accurately measures inspired anaesthetic concentration, which may be substantially lower than the vaporizer setting in rebreathing systems during anaesthesia in large dogs, horses and ruminants (see Fig. 2.5). A situation may arise where administration of an anaesthetic agent may be inadequate despite an apparently adequate vaporizer setting. In a retrospective study of equine anaesthesia, it was found that horses were four times more likely to move during anaesthesia when an anaesthetic agent analyser was not used (Parviainen & Trim, 2000).
Some monitors using the principles of infrared absorption spectrometry cannot be used for horses or ruminants as they will measure exhaled methane and record the concentration as halothane, for example the Datex Capnomac/Normac (Taylor, 1990). There may be minimal effect with isoflurane but negligible with sevoflurane and desflurane. Analysers that use higher wavelengths of infrared light should be unaffected by methane (Moens et al., 1991).
EEG and Bispectral index
The subject of encephalogram (EEG) guidance to depth of anaesthesia has been discussed in Chapter 1 and Murrell and Johnson (2006) have published a review of the techniques using bispectral index and somatosensory evoked potentials for assessment of pain in animals.
Intraoperative awareness occurs when a patient becomes conscious during general anaesthesia. Significant psychological effects have been identified in humans after episodes of awareness that later are associated with changed behaviour (Apfelbaum et al., 2006). The American Society of Anesthesiologists (ASA) task force identified certain procedures and anaesthetic techniques during anaesthesia of human patients that may be associated with intraoperative awareness and these included caesarian section, cardiac surgery, trauma surgery, rapid-sequence induction, total intravenous anaesthesia, and use of reduced anaesthetic drug dosages with or without neuromuscular paralysis. Awareness has been reported in the absence of tachycardia or hypertension. Nonetheless, clinical techniques such as observation for lack of movement, the palpebral reflex, and other monitors are useful to assess anaesthetic unconsciousness.
Monitoring of brain electrical activity has clinical application and a number of monitors have been developed. Various signal processing algorithms can be applied to the frequency, amplitude, and latency of the EEG to generate a number. This number, or ‘index’, is scaled 0 to 100 with a value of 100 associated with wakefulness and 0 referring to an isoelectric EEG. One monitor, the Bispectral Index (BIS) has become the one most used. BIS is a proprietary algorithm based on a large number of EEG recordings taken during human anaesthesia that converts a single channel frontal EEG to an index 0 to 100, of which a range of 40 to 60 is reported to be associated with anaesthesia without awareness or decreased incidence of awareness when compared with anaesthesia monitored by other means. It has also been proposed that targeting a specific BIS index range may avoid excessive depth of anaesthesia such that the patients enter recovery at a lighter plane of anaesthesia with less respiratory depression and experience shorter recovery times. Concerns are that the numeric value may be influenced by other factors such as bolus administration of anaesthetic drugs, electrocautery, or cerebral hypoxia, and may not accurately reflect the depth of anaesthesia and initiate an inappropriate change in anaesthetic administration. Further, that use of a brain function monitor may result in excessively deep anaesthesia to the detriment of organ blood flow and function. Several prospective multicentre controlled trials involving human patients have evaluated the role of BIS monitoring in determining depth of anaesthesia and reliability for prevention of awareness during intravenous or inhalant general anaesthesia (Bruhn et al., 2005; Avidan et al., 2008; Ellerkmann et al., 2010; Kertai et al., 2011). The results thus far have not supported a unified view, however, it appears that BIS monitoring may have an impact on incidence of awareness but not influence the amount of anaesthetic agents administered or influence postoperative mortality.
The use of BIS monitoring is being investigated in animals. Electrodes manufactured for human patients may require modification and reconfiguration to adjust to the differences in shape and nature of animals’ heads. Decreasing BIS values have been recorded with increasing concentrations of isoflurane and sevoflurane in dogs and cats (March & Muir, 2003b; Lamont et al., 2004; Campagnol et al., 2007). Burst suppression of the EEG, characterized by periods of low amplitude waves interspersed with bursts of high amplitude waves, occurs during deep anaesthesia (2.0 MAC) resulting in paradoxical increases in BIS values (Lamont et al., 2004; Henao-Guerrero et al., 2009). Concern has been expressed that the BIS range for animals may not be the same as the accepted range for anaesthesia in humans. A study in experimental cats found that pedal and palpebral reflexes were lost at BIS values 66 to 67, however, these values may not be associated with a surgical depth of anaesthesia (March & Muir, 2003b). BIS values increased significantly in anaesthetized cats in response to a noxious stimulus (March & Muir, 2003a). The relationship between BIS and clinically evaluated anaesthetic depth in dogs was found to be unsatisfactory (Bleijenberg et al., 2011). Although BIS decreased significantly between sedation and anaesthesia, BIS values overlapped significantly between light and deep anaesthesia. Similarly, in horses anaesthetized with isoflurane, BIS monitoring was not found to be a useful predictor of depth of anaesthesia or warning of patient movement (Haga & Dolvik, 2002). In another investigation in horses anaesthetized with sevoflurane and undergoing a variety of surgical procedures, the mean BIS value was >60 and changes in BIS values were not detected in all horses that moved during anaesthesia (Belda et al., 2010). Further investigations are needed to define the recommendations for BIS monitoring in veterinary medicine.
Another monitor used in human anaesthesia is the cerebral state index (CSI) monitor. The CSI is not dependent on algorithms derived from human patients (see Chapter 1) and, consequently, was hoped to be more reliable in domestic species. However, Ribeiro et al. (2009) investigating induction of anaesthesia with propofol in dogs concluded that CSI monitoring was not consistent with the clinical observations observed in different stages of depth of anaesthesia.
Monitoring the circulation
The heart rate, pulse rate and rhythm, tissue perfusion, and blood pressure of all anaesthetized animals should be assessed at frequent regular intervals (Table 2.3).
Pulse rate and rhythm
The peripheral pulse rate and rhythm may be counted by palpation of a peripheral arterial pulse, such as the femoral artery in dogs and cats, lingual artery in dogs (Fig. 2.6), facial, median or metatarsal arteries in horses (Fig. 2.7), and femoral, median, or auricular arteries in ruminants and pigs. The pulse rate and rhythm can also be determined from the audible sound of blood flow from a Doppler ultrasound probe (see later) and as a digital number and waveform generated by a pulse oximeter. The physiological relationship between the heart sounds, ECG, and arterial pulse is depicted in Figure 2.8. Pulse deficits are identified by palpation of a peripheral pulse that has an irregular rhythm, pauses, or a pulse rate that is less than the heart rate determined by auscultation of the heart using a stethoscope. Pulse deficits may be associated with any of several cardiac dysrhythmias, such as sinoatrial heart block, atrioventricular heart block, premature ventricular depolarizations, or atrial fibrillation, and will require an ECG for diagnosis.
Figure 2.6 The lingual artery is easily palpated in dogs midline on the ventral surface of the tongue, adjacent to the nerve and between the lingual veins. The arrow in the photograph points to a line drawn adjacent to the lingual artery.
Figure 2.7 Sites for palpation of arterial pulse or catheter placement for blood pressure measurement in horses.
Heart rate monitors
Heart rates measured before anaesthesia are greatly influenced by the environment. Means (standard deviations, range) of heart rates obtained by palpation from healthy cats at home were 118 mean (SD 11, range 80–160) beats per minute compared with mean 182 (SD 20, range 142–222) when obtained by electrocardiography in the veterinary hospital (Sawyer et al., 1991).
The lowest acceptable heart rates during anaesthesia are controversial, but reasonable guidelines are 55 beats/min for large dogs, 65 beats/min for adult cats, 26 beats/min for horses, and 50 beats/min for cattle. Heart rates should be higher in small breed dogs and much higher in immature animals. Heart rates may be less than these values when medetomidine/dexmedetomidine has been administered. It should be remembered that heart rate is a major determinant of cardiac output, consequently, bradycardia should be treated if blood pressure and peripheral perfusion are also decreased.
The oesophageal stethoscope (Fig. 2.9) is a simple method of monitoring heart rate in dogs and cats. This monitor consists of a tube with a balloon on the end which is passed dorsal to the endotracheal tube and into the oesophagus until the tip is level with the heart. The open end of the tube is connected to an ordinary stethoscope headpiece, to a single earpiece that can be worn by the anaesthetist or surgeon, or connected to an amplifier that makes the heart sounds audible throughout the room. This monitor provides only information about heart rate and rhythm; the intensity of sound is not reliably associated with changes in blood pressure or cardiac output. Other electronic oesophageal probes are available to provide heart rate, and some also may produce an ECG and oesophageal temperature.
Heart rate and rhythm can be obtained from an ECG using standard limb leads in small animals, clip electrodes and ECG paste or gel, and selecting ECG Lead II on the oscilloscope (Fig. 2.10A). Poor contact of the electrodes to the skin from hair bunched in the clips, or close proximity to another electrical apparatus, such as the hot water circulating pad, can result in electrical interference that obscures the ECG. The leads should not be placed over the thorax as breathing will move the electrodes and result in a wandering ECG baseline (Fig. 2.10B).
Figure 2.10 (A) Normal lead II ECG from a Labrador during inhalation anaesthesia. (B) ECG with a wandering baseline induced by movement of leads as the dog breathes. The bottom trace is arterial blood pressure. This dog has a heart rate of 114 beats per minute, systolic arterial pressure of 96 mmHg, diastolic pressure of 46 mmHg, and mean pressure of 61 mmHg. (C) Normal base apex ECG and blood pressure recorded from a horse anaesthetized with isoflurane.
A frequently used monitor lead in equine anaesthesia is the ‘base-apex’ lead. The right arm electrode is clipped on the neck in the right jugular furrow and the left arm electrode is passed between the forelimbs and clipped at the apex of the heart over the left fifth intercostal space several inches from the midline. The left leg electrode is clipped on the neck or on the shoulder. Good electrical contact is achieved with alcohol or electrode paste. Lead I is selected on the electrocardiograph and the normal configuration includes a negative R wave (see Fig. 2.10C). A bifid P wave is frequently observed in the normal equine ECG. Sinus arrhythmia in dogs and cats is abolished when atropine or glycopyrrolate has been administered. Other arrhythmias, for example second degree atrioventricular heart block and ventricular premature depolarizations (VPD) or premature ventricular complexes (PVC), may or may not require specific treatment (see Chapter 21).
There is a high incidence of sinus arrhythmia and first and second degree atrioventricular (AV) heart block in conscious unsedated horses (Robertson, 1990). In contrast, AV block during anaesthesia is uncommon except when the horse has been premedicated with detomidine, or supplemental intravenous injections of xylazine are given during anaesthesia. The appearance of this arrhythmia during anaesthesia on any other occasion is cause for concern as this rhythm may progress within a few minutes to advanced heart block (P waves only, no ventricular complexes) and cardiac arrest. Atrial fibrillation and PVCs occur rarely in horses but may require specific treatment if associated with hypotension.
Tissue perfusion
Evaluation of tissue perfusion can be done by considering gum or lip mucous membrane colour, the capillary refill time, and the blood pressure. High mean arterial pressure does not guarantee adequate tissue perfusion. For example, when blood pressure increases during anaesthesia in response to a surgical stimulus, cardiac output may be decreased due to increased afterload from peripheral vasoconstriction.
Tissue perfusion is usually decreased when the gums are pale, rather than pink, sometimes when very pink, and the capillary refill time (CRT) exceeds 1.5 seconds, or the mean arterial pressure (MAP) is less than 60 mmHg. When MAP is above 60 mmHg, palpation of the strength of the peripheral pulse and observation of oral membrane colour and CRT should be used to assess adequacy of peripheral perfusion and cardiac output. During laparotomy, intestinal colour should be bright pink and intestines that are pale pink, white, or grey may be an indicator of inadequate tissue perfusion. Measurement of central venous O2 saturation may provide further information in sick patients, as described later.
Arterial blood pressure
Systolic (SAP), mean (MAP), and diastolic (DAP) arterial pressures in awake healthy animals are approximately 125–160 mmHg, 90–110 mmHg, and 75–95 mmHg, respectively. Excepting when premedication has included detomidine or medetomidine or when anaesthesia was induced with ketamine or tiletamine, arterial blood pressure is decreased from the awake value during anaesthesia. Arterial blood pressure is lower in paediatric patients than in mature animals. For example, healthy 5- or 6-day-old foals anaesthetized with isoflurane had an average MAP of 58 mmHg. When the same foals were reanaesthetized 4–5 weeks later, the average MAP had increased to 80 mmHg, with a corresponding decrease in cardiac index (Hodgson et al., 1990).
Hypotension may be defined as MAP less than 65 mmHg in mature animals. A MAP as low as 60 mmHg may be allowed in dogs and cats provided that the mucous membrane colour is pink and CRT is 1 second. This combination of values may occur during inhalation anaesthesia at the time of minimal stimulation during preparation of the operative site and before the onset of surgery. MAP is not usually allowed to fall below 65–70 mmHg for any length of time in anaesthetized horses because of the increased risk for postanaesthetic myopathy. When hypotension is documented, appropriate treatment can be instituted, such as decreasing anaesthetic depth or commencing or increasing the intravenous administration of fluids or administration of a vasoactive drug such as dopamine, dobutamine, or ephedrine. The outcome of untreated severe or prolonged hypotension may be unexpected cardiac arrest during anaesthesia or neurological deficits, blindness or renal failure after recovery from anaesthesia.
An approximate estimate of blood pressure can be made from palpation of a peripheral artery. However, strength of a palpated pulse is determined by the pulse pressure (difference between systolic and diastolic pressures) and, in states associated with vasodilatation, a peripheral pulse can be palpated at MAP as low as 40 mmHg so that, in some cases, palpation alone does not suggest the urgency for treatment. The turgidity of the artery will provide further information because pressure is likely to be low if the artery is soft or collapsible rather than solid like a pencil. Bear in mind that it is not uncommon for heart rates to be within an accepted normal range while blood pressure is low or decreasing (Fig. 2.11).
Figure 2.11 Blood pressure (Doppler systolic) decreased without a change in heart rate after this hound was turned over (Arrow 1) during anaesthesia. Blood pressure continued to decrease after isoflurane was discontinued. Infusion of dobutamine (Arrow 2) produced a rise in blood pressure.
Measurement of blood pressure can be made easily; equipment cost varies. The investment in time and money is worthwhile in animals at risk for hypotension, such as small animals and horses anaesthetized with inhalation agents, and in animals with abnormalities likely to give rise to complications during anaesthesia. The least expensive techniques are the Doppler ultrasound technique in dogs and cats, and direct blood pressure measurement using an anaeroid manometer in horses, however, purchase of a unit that monitors more than one parameter, for example, ECG, blood pressure, and temperature, is more economical than acquiring monitors individually.
Hair is first clipped from the skin on the palmar surface of the paw of dogs and cats (Fig. 2.12). A probe covered with contact gel is placed over the artery and taped in place with the electrical lead pointing down towards the toes (Fig. 2.13). Ultrasound waves emitted from one of the two piezoelectric crystals embedded in the probe pass through the skin and deeper tissues. A structure that is stationary will reflect sound back to the second crystal without any frequency change (Stegall et al., 1968). Moving objects, such as erythrocytes and the artery wall, will reflect some of the sound at a different frequency (Doppler-shift). The change in frequency can be heard through a loudspeaker as an audible swooshing sound with each pulse.
Figure 2.12 Sites for application of the Doppler probe for indirect measurement of arterial blood pressure in dogs. 1 & 2: Ulnar artery on the caudal surface of the forelimb, above and below the carpal pad; 3: cranial tibial artery on the craniolateral surface of the hindlimb; 4 & 5: saphenous artery on the medial surface of the flexor tendons and on the plantar surface of the paw proximal to the foot pad; 6: dorsal pedal artery; 7: coccygeal artery on the ventral surface of the tail.
Figure 2.13 Doppler-shift pulse detector. One piezoelectric crystal emits incident ultrasound signal while the other receives the reflected signal from cells in flowing blood. The frequency shift between the incident and reflected sound is converted to audible sound.
A cuff is wrapped snugly around the extremity proximal to the probe, in dogs and cats with the centre of the inflatable part of the cuff, or an arrow or dot, on the medial aspect of the limb and the air tube positioned on the distal end of the cuff. The cuff is connected to an anaeroid manometer and a bulb for manual inflation of the cuff with air (Fig. 2.14). In horses, the cuff is wrapped around the base of the tail with the cuff air bladder centred over the ventral surface of the tail. The probe is taped distal to the cuff over the coccygeal artery in the ventral midline groove. The coccygeal artery can be used for this technique in adult cattle but the results are not reliable. In foals, the probe and cuff are commonly attached to the tail. In foals and small ruminants in lateral recumbency, the probe can be taped over the metatarsal artery on the lateral surface of the hind limb or the common digital artery on the medial side of the forelimb distal to the carpus. The cuff is secured around the limb above the hock or carpus and positioned level with the sternum. In pigs, the probe is most reliable when taped over the common digital artery on the caudomedial aspect of the forelimb. The cuff should be placed between the carpus and the elbow but, because of the triangular shape of the forearm, it may be unable to occlude blood flow when inflation of the cuff causes it to slip down over the carpus. For all animals, the width of the air bladder within the cuff is important for accuracy; a bladder that is too narrow will overestimate blood pressure and one that is too wide will underestimate it. The cuff (air bladder) width should be 40% of the circumference of the extremity and the leg positioned so that the cuff is level with the manubrium. Furthermore, a cuff that is attached too loosely or slips down the extremity and becomes loose, or situated over a joint such as the carpus will result in an erroneously high value.
Figure 2.14 Measurement of arterial pressure in a dog by taping a Doppler probe over an artery distal to the carpal pad so that audible sounds of arterial pulses are emitted from the box. A blood pressure cuff is applied higher up the limb and the anaeroid manometer attached to the cuff is used to identify systolic and diastolic pressures.
To measure blood pressure, the cuff is inflated to above systolic pressure to occlude the artery and no sound is heard. The pressure in the cuff is gradually released until the first sounds of blood flow are detected at systolic pressure. As additional pressure is released from the cuff, diastolic pressure is heard as a change in character of sound from a one or two beat sound to a multiple beat sound, to a muffling of sound, or to a growl. This will occur 15–40 mmHg below systolic pressure. The sounds associated with diastolic pressure are well defined in some animals but not at all clear in others. In some animals, a first muffling of beat signals may occur 10–15 mmHg above the true diastolic pressure. In this event, the second change in beat signal will be more abrupt or distinct. Mean pressure can be calculated as one third of the pulse pressure (systolic−diastolic) plus diastolic pressure.
A decrease in intensity of the pulsing sound, when the attachment and setting have been unchanged, is a reliable indication of decreased blood flow. Furthermore, changes in cardiac rhythm are easily detected by listening to this monitor.
The Doppler ultrasonic method was observed to provide both overestimated and underestimated values over a wide range of systolic pressures in conscious and anaesthetized dogs, with a tendency for overestimation when MAP <80 mmHg and underestimation at high pressures (Haberman et al., 2006; Bosiack et al., 2010). An investigation in cats comparing the Doppler ultrasonic method, using a cuff placed halfway between the carpus and the elbow, with measurements obtained from a femoral artery catheter revealed that the indirect method consistently underestimated systolic pressure by an average of 14 mmHg (Grandy et al., 1992). Caulkett et al. (1998) also determined that the Doppler method both overestimated and underestimated systolic blood pressure in anaesthetized cats but that it proved to be an accurate predictor of MAP. Using this technique of measuring blood pressure in mature horses using a cuff width 48% of the circumference of the tail (bladder width 10.4 cm) systolic pressure was underestimated and diastolic pressure overestimated by approximately 9% (Parry et al., 1982). In another investigation, measurements of systolic arterial pressure in horses anaesthetized in dorsal recumbency with halothane using a cuff 41% of the tail circumference was reasonably accurate but, in 5% of the horses, this technique had an error range of ±20 mmHg (Bailey et al., 1994).
Devices that non-invasively measure peripheral blood pressure using the oscillometric method operate by automatically inflating a cuff placed around an extremity, either above or below the carpus, above or below the hock, or the tail (Fig 2.15). The monitor measures and records the amplitude of pressure changes in the cuff caused by pulses in underlying arteries. The cuff is inflated to above systolic pressure and deflated slowly. The pressure oscillations in the cuff start small and increase to a maximum value (corresponding with MAP) and then decrease. Each monitor uses fixed or variable parameter identification points based on the MAP value to calculate electronically systolic and diastolic pressures. The algorithm determining systolic and diastolic pressures varies between models and thus measurement results are not the same for all models. There is a also small difference in the recorded MAP and MAP calculated from the systolic and diastolic pressures using the formula MAP = diastolic pressure + 0.33 (systolic − diastolic) and this value is dependent on the measurement device (Kiers et al., 2008). Values for SAP, DAP, MAP, and heart rate are digitally displayed and the monitor can be programmed to measure automatically at a specified time interval. The cuff should be situated approximately level with the heart (manubrium or sternum if in lateral recumbency) for accurate measurement. Artefactual pressure changes induced in the cuff by movement of the extremity, for example, during preparation of the surgical site, will either induce abnormal readings or prevent the monitor from obtaining a measurement (Haberman et al., 2006; Bosiack et al., 2010). Closest correlations between direct and indirect measurements have been measured using cuff widths of 40% of the circumference of the animal’s leg or tail.
Figure 2.15 Non-invasive measurement of blood pressure by oscillometry. The cuff should be positioned level with the manubrium or sternum, and for big dogs it may be necessary to hold the limb elevated during measurement to achieve alignment. A Doppler ultrasound probe is also attached to the limb of this dog for audible recognition of blood flow.
By far the highest number of published articles comparing NIBP and IBP measurements have been in dogs. Not all investigations have produced similar results, in part influenced by use of different models from several manufacturers, measurement in awake versus anaesthetized animals, increased variability attributed to haemodynamic alterations imposed by disease, differences in size and gender, sites of measurement, patient body position, pharmacological methods used to alter blood pressure, and the investigators varied definitions of hypotension or hypertension. The ‘take home’ message is that NIBP does not accurately predict IBP all of the time and that no treatment decision should be made based solely on one measurement. Investigations of specific models have employed the average of three to six consecutive readings taken 30–60 seconds apart to increase accuracy. Frequently during anaesthesia, the site of surgery and accessibility dictates the site for application of the cuff. Different sites have been investigated with the result that the forelimb in dogs was determined to be more accurate when compared with lingual artery pressure and the hind limb more accurate when compared with dorsal pedal artery pressure (McMurphy et al., 2006), no difference between measurements was recorded between the fore and hind limbs (Sawyer et al., 1991), or that the tibial or coccygeal arteries provided better correlation than the metacarpal artery when compared with aortic or anterior tibial artery pressure (Sawyer et al., 2004; Haberman et al., 2006). Most oscillometric monitors provide a reasonable approximation of MAP in normotensive animals.
The greatest concern during most anaesthetic episodes is the accurate identification of hypotension to avoid cardiac arrest or postoperative organ malfunction. The Surgivet V60046, with the cuff placed distal to the hock, provided accurate information for MAP in normotensive and hypotensive dogs, with 90.6% of the dogs with MAP <70 mmHg being accurately diagnosed as hypotensive (Deflandre & Hellebrekers, 2007). The monitor was less accurate at high pressures. The Cardell 9401, DINAMAP (Device for Indirect Noninvasive Automatic Mean Arterial Pressure) 8100, DINAMAP 8300 and PetMAP overestimated pressures during hypotension (Sawyer et al., 1991; Meurs et al., 1996; Bosiack et al., 2010; Shih et al., 2010), and did not reliably predict the severity of hypotension in animals with acute haemorrhage or in critically-ill patients in the ICU. Wernick et al. (2010) compared measurements in anaesthetized dogs between pressures recorded from the dorsal pedal artery and from a cuff around the antebrachium using the DINAMAP 8300. The DINAMAP slightly underestimated SAP and DAP and overestimated MAP; comparisons that fell just short of the validation requirement proposed by the American College of Veterinary Internal Medicine (Brown et al., 2007). In contrast, the DINAMAP 8300 underestimated all BP parameters in awake experimental Beagles, and to a greater extent at high pressures generated by administration of phenylephrine (Haberman et al., 2006). Comparisons with the Cardiocap II in clinical canine patients using premedication to alter blood pressure (acepromazine or medetomidine) generated a smaller bias than previously reported (Sawyer et al., 2004; McMurphy et al., 2006) but greater limits of agreement (variability) that was attributed to variation in clinical population (MacFarlane et al., 2010).
In clinical practice, it may be necessary to identify hypertension before anaesthesia and to measure accurately high pressures during anaesthesia in patients at risk, such as phaeochromocytoma, renal or endocrine disease. Oscillometric methods tend to underestimate systolic blood pressures during hypertension (Haberman et al., 2006; McMurphy et al., 2006; Deflandre & Hellebrekers, 2007; Bosiack et al., 2010), consistently underestimate MAP or DAP (Haberman et al., 2006), overestimated MAP or DAP (Bosiack et al., 2010), or underestimated SAP and DAP with close agreement with MAP (McMurphy et al., 2006).
The Cardell 9301V with 2.5 cm-wide neonatal pressure cuff placed around the forelimb above the carpus, with the monitor set at ‘small cuff’ mode has been evaluated in cats (Pedersen et al., 2002). Low pressure MAP <60 mmHg was achieved by increasing depth of anaesthesia with isoflurane and high pressures MAP >140 mmHg were created by administration of medetomidine. Mean differences between NIBP and IBP were <5 mmHg for MAP and DAP in all pressure ranges; SAP differences increased as pressure increased from hypotension to hypertension resulting in underestimation of SAP. The DINAMAP had good correlation with IBP systolic pressure in anaesthetized cats (Caulkett et al., 1998), however, the Datascope Passport did not accurately estimate direct blood pressure in cats (Branson et al., 1997).
In horses, the oscillometric method of blood pressure measurement is used when direct measurement of arterial pressure is not possible, for total intravenous anaesthesia, and for awake animals. The cuff is wrapped around the tail of mature horses and foals or around the hind limb near the metatarsal artery in foals. The cuff should not be wrapped tightly. Some investigators have recommended that the tail cuff be placed close to the base of the tail but, in this author’s opinion, more accurate readings are obtained with the cuff applied approximately 10 cm from the base of the tail, where the tail diameter is constant for the length of the cuff. In standing horses, cuff placement may be higher than the atrium, resulting in a measurement that is lower than the actual pressure. Early investigations of the DINAMAP confirmed accurate and clinically useful values for arterial pressure using a cuff width 24% of the tail circumference in ponies (Geddes et al., 1977) and 25–35% in horses (Latshaw et al., 1979; Muir et al., 1983). However, measurements were inaccurate at heart rates of less than 25 beats/minute. Our experience using a Model 8300 DINAMAP and a cuff width 35–40% of the tail circumference ratio (child or small adult cuff for a mature horse depending on tail thickness and the amount of hair) has been that MAP value obtained from this monitor is usually the same as that obtained by direct blood pressure measurement. Occasionally, the DINAMAP recorded pressures 10–20 mmHg higher than the true MAP. An evaluation of the Cardell Model 9402 and the DINAMAP Pro 100 in anaesthetized foals of less than 7 days of age using cuff sizes recommended by the manufacturers revealed that both monitors had similar performance (Giguère et al., 2005b). Analysis of MAP values confirmed acceptable accuracy with the cuffs on the tail or metatarsus for conditions of normotension and hypotension, although the Cardell was most accurate over the coccygeal artery and the DINAMAP over the metatarsal artery. Cuffs placed over the median artery produced less accurate values and wider variability. This study also confirmed that blood pressure did not correlate well with cardiac output, i.e. a good MAP does not guarantee that cardiac output is also acceptable. In summary, indirect method of measurement of blood pressure provides useful information in most horses, but may produce erroneous values in a small number. Consequently, blood pressure should be measured by direct means whenever possible in horses at risk of developing low blood pressure, for example, during inhalation anaesthesia.
Standards have been set for automated sphygmomanometers in human medicine by several organizations, including the British Hypertension Society, the Association for the Advancement of Medical Instrumentation (AAMI) and the American National Standards Institute, Inc. (ANSI). Devices validated for use in humans must have a correlation of ≥0.9 across a range of measurements with specific % of readings to be within 5, 10, or 15 mmHg of the standard measurement. Requirements for validation were tightened in 2011. These standards have been met for MAP at low and normal pressures in dogs with the Surgivet V60046 (Deflandre & Hellebrekers, 2007), for MAP and DAP in cats with the Cardell 9301V (Pedersen et al., 2002), and for MAP in foals using the Cardell 9402 and DINAMAP Pro 100 (Giguère et al., 2005b). For the most part, oscillometric blood pressure monitors provide useful information for management of the clinical patient. Trends in pressures can be used to direct management of anaesthesia and, although hypotension may not be accurately diagnosed, a decrease in measurements to low values should be a warning that action should be taken. A single abnormal measurement should be confirmed by taking two more readings. Failure of the monitor to achieve a reading (‘timed out’) when it had just previously been recording may indicate a change in peripheral blood flow. It must be remembered that although on average there is reasonable correlation between direct and indirect pressure measurements, in individual patients the difference in readings can be up to 20 mmHg and a patient may not be identified as having a MAP outside an accepted range. Therefore, NIBP readings must be assessed together with other observations of cardiovascular performance and depth of anaesthesia.
In contrast to previously described oscillometric techniques, high-definition oscillometric (HDO) technology discriminates between pressure waveform changes that are characteristic for systolic, mean, and diastolic pressures. The technology allows much more rapid assessments that minimize the impact of outside factors, and is able to accommodate fast heart rates and weak pulse signals. The expectation is for increased accuracy at high and low blood pressures and increased reliability for use in ill patients. In anaesthetized dogs, comparison between IBP measured at the dorsal pedal artery and a high-definition oscillometric device, Memodiagnostic MD_15/90 Pro, revealed that values for SAP, MAP, and DAP were all overestimated by HDO and the overestimation increased for SAP and DAP with increasing pressures (Wernick et al., 2010). The precision exceeded the 15 mmHg limit required for validation and only 50–60% of readings were within 10 mmHg. In a group of conscious experimental Beagles with implanted aortic devices for telemetry of blood pressures, measurement of pressures from a cuff around the base of the tail using HDO revealed that MAP most closely paralleled the telemetered pressures (Meyer et al., 2010). The standards set by AAMI were not met in this study. The authors noted that the mean standard deviations were substantially decreased by use of six averaged measurements at any time point compared with a single measurement.
Measurement of arterial blood pressure directly is accomplished by insertion of a 20 gauge, 22 gauge or, in very small animals, a 24 gauge catheter aseptically into a peripheral artery; the dorsal pedal, anterior tibial, femoral, coccygeal, metacarpal, auricular or lingual artery in dogs (Fig. 2.16), the dorsal pedal, femoral or coccygeal artery in cats, the lateral nasal, facial, transverse facial, or metatarsal artery in horses (see Fig. 2.7), or an auricular artery in ruminants, pigs and rabbits (Fig. 2.17). Placement of a catheter in an artery in most animals is not difficult with experience. Complications are rare provided there is strict attention to asepsis during insertion, wrapping to maintain cleanliness, use of sterile solutions for flushing, and appropriate pressure on the artery when the catheter is removed to avoid a haematoma. The cap or extension must be secured firmly to the arterial catheter to prevent accidental disconnection and blood loss. The catheter is connected by saline-filled tubing to either an anaeroid manometer (Fig. 2.18) or an electrical pressure transducer (Fig. 2.19) for measurement of arterial pressure. Either an air gap or a connector containing a latex diaphragm should be maintained next to the anaeroid manometer to prevent saline entering the manometer and to maintain sterility. An optional addition to the electrical transducer is a continuous flushing device (Fig. 2.20) that can be inserted between the transducer and the artery. This device is connected to a bag of saline that has been pressurized to 200 mmHg and will deliver 2–4 mL saline/hour to help prevent clotting of blood in the catheter. Extension tubing sold specifically for arterial pressure measurement is non-distensible to preserve an accurate waveform.
Figure 2.16 Sites for insertion of arterial catheters on the cranial aspect of the right hind limb of a dog.
Figure 2.19 Direct measurement of blood pressure in a horse using a catheter in the facial artery connected by saline-filled tubing to an electrical transducer.
Figure 2.20 Continuous infusion valve (c) for attachment to a pressure transducer (b). A pressurized bag of intravenous fluid (d) is connected to the device to give a continuous infusion of 3 mL per hour. With this particular version, rapid flushing of the saline-filled tubing (a) to the arterial catheter is achieved by squeezing the tabs (arrow) around the valve.
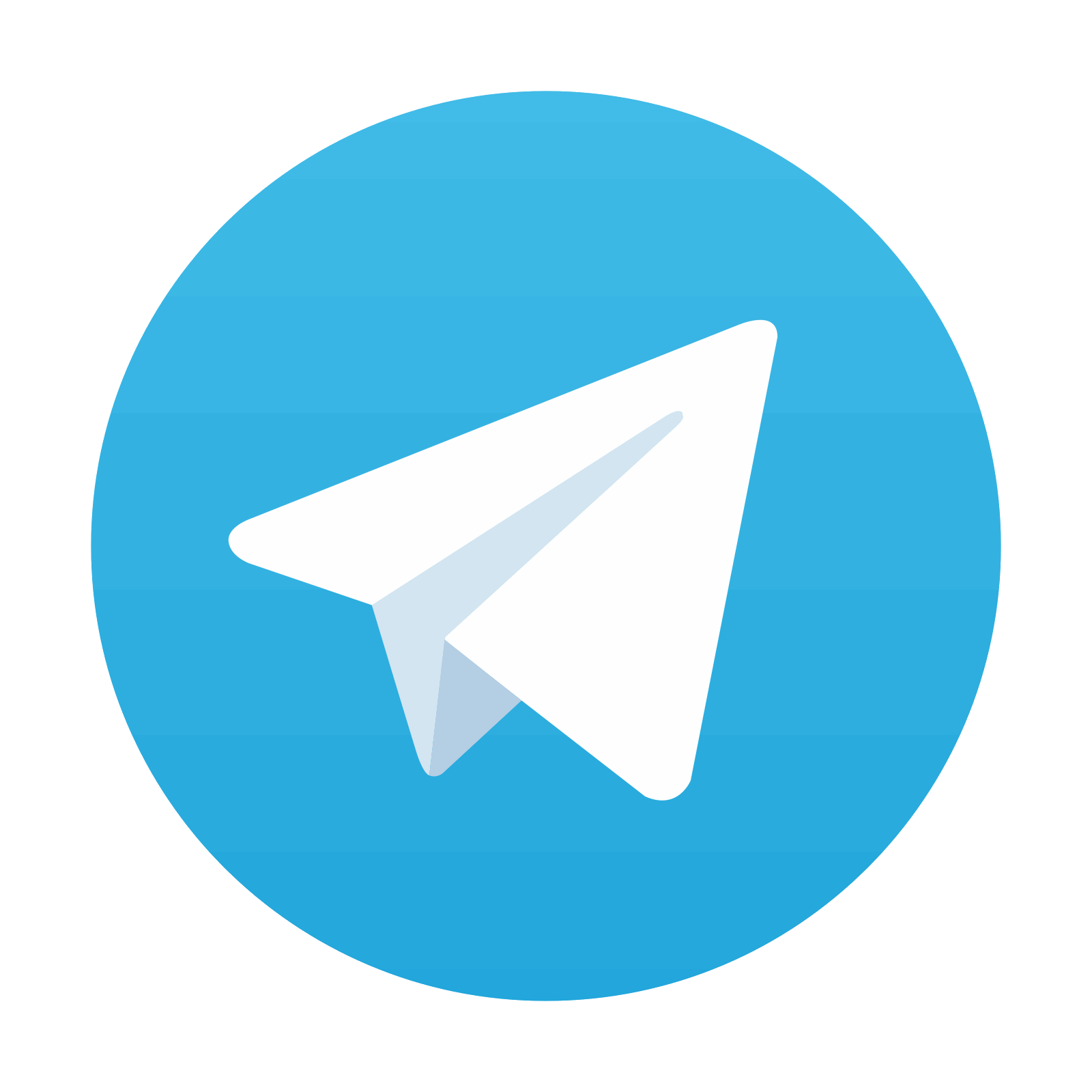
Stay updated, free articles. Join our Telegram channel

Full access? Get Clinical Tree
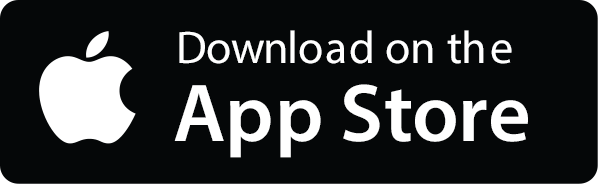
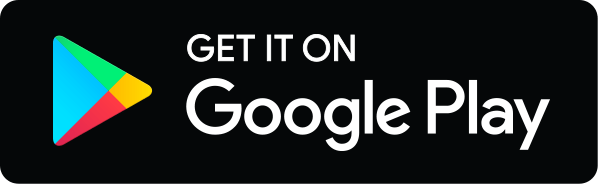
