Radiation for the treatment of veterinary patients is a limited but invaluable resource. It has many applications in veterinary medicine and can be used as sole treatment or more commonly in combination with surgery or chemotherapy. It is a localized treatment and therefore has limited application for patients with disseminated tumours.
How does radiation kill cells?
In the energy ranges used in veterinary oncology the major effect is an indirect one due to the production of free radicals. Living cells consist of approximately 85% water, so the major target of ionizing radiation are water molecules resulting in the production of hydroxyl radicals that in turn damage the DNA. The effects of radiation are not specific for cancer cells and rapidly proliferating normal tissues are susceptible to damage and are known as acutely or early-responding tissues. More slowly dividing normal cells (e.g. bone) are affected by radiation but the changes may take months to years to become apparent; such tissues are known as late-responding tissues.
Cell death
Most cells die what is known as a reproductive death and at standard clinical doses the effect of radiation is limited to dividing cells. Clinical effect is dependent on the growth fraction and doubling time of the tumour cells.
Cell death not requiring mitosis can be achieved at high doses when resistant cells become susceptible. A special case is lymphoid tissue that undergoes both mitotic and interphase death at low radiation doses.
The oxygen effect
Hypoxia protects cells from radiation damage; conversely, high oxygen levels fix the free radical damage. Most cells within a tumour are to some degree hypoxic, as a distance of 100 µm from a capillary bed results in hypoxia.
Delivery of radiation
Ionizing radiation can be delivered by an external source (teletherapy), by application of radioactive sources interstitially (brachytherapy) or systemically by radioactive isotopes, e.g. iodine-131 (131I).
External beam radiotherapy
Orthovoltage
• Produces x-rays of low to medium energy (150–400 kVp).
• Penetration is low with maximum dose delivered to the skin.
• Increased absorption of radiation in bone relative to soft tissue.
• Not suitable for treating deep-seated tumours.
There are still a few centres worldwide that use orthovoltage machines for veterinary patients but the wider availability of megavoltage machines continues to make these machines obsolete.
Cobalt-60
• First of the megavoltage machines that allowed treatment of deep-seated tumours.
• Skin-sparing effect means the maximum dose of radiation is not delivered to the skin.
• Cheaper to install than a linear accelerator, but has potential radiation considerations due to the presence of a radioactive source.
A few centres worldwide do have cobalt units for the treatment of veterinary patients but newer facilities are installing linear accelerators.
Linear accelerator (Linac)
These megavoltage machines have a number of energy ranges; the most commonly used machines are those with energy of 6 MV (6 million volts) (Figure 7.1).
![]() |
Figure 7.1 |
The advantage over orthovoltage machines is the skin-sparing effect. The skin is spared because the maximum dose is delivered at some distance below the skin surface, resulting in fewer side effects to the skin and the ability to treat deep-seated tumours. The point at which maximum dose is delivered (Dmax) depends on the energy of the machine. The absorption of megavoltage radiation is not dependent on the density of the tissue and so permits an even distribution of radiation throughout all tissues in the field, i.e. bone does not preferentially absorb radiation and therefore reduces the risk of complications involving bone.
Megavoltage radiation results in less scatter and therefore less radiation sickness.
The drawback to installation of a Linac is the cost of the installation, machine and running costs.
Brachytherapy
• Iridium-192 (192Ir) wires
• Strontium-90 (90Sr)
Brachytherapy is rarely used in veterinary oncology because of the difficulties in using implants in veterinary patients (reviewed by Walker 1997). Isolation wards are required and, depending on the implant, the patient may require isolation for days to weeks. However, 192Ir implants have been used in the treatment of nasal tumours (Thompson et al 1992). Strontium-90 has limited application in the treatment of very small, superficial nasal planum squamous cell carcinoma in the cat or small superficial mast cell tumours in the cat (Turrel et al 2006). Brachytherapy units are commercially available that will undoubtedly find increasing application for veterinary patients.
What are the goals of radiotherapy in the veterinary patient?
Curing the veterinary patient
Radiotherapy as the definitive treatment is rare in veterinary patients and is usually seen as an adjunctive treatment, e.g. in the management of incompletely resected mast cell tumours and soft tissue sarcomas. The most common situation where radiotherapy may be used as the sole treatment with curative intent is in patients with localized lymphoma, e.g. it is the treatment of choice for feline patients with nasal lymphoma (Figure 7.2A,B; see Chapter 22).
Adjunctive therapy for incompletely resected tumours
Adjunctive therapy is the most common application of radiotherapy in veterinary medicine. The typical patient has had either a mast cell tumour or a soft tissue sarcoma removed with incomplete margins and radiotherapy is used to clean up the ‘dirty’ margins when a further surgery is not possible because of anatomical considerations or client preference (Figure 7.2C). This means that the tumours are predominantly located on the distal extremities or facial area. See the relevant chapters for a more in-depth discussion.
Neoadjuvant therapy for large inoperable tumours
In cases where a tumour is too large to be surgically excised, or too deeply attached to underlying structures, radiotherapy can be used to shrink the tumour to make it operable (Figure 7.2D).
Palliative therapy
Radiotherapy can be used to control pain in patients with inoperable bone tumours (Thrall & LaRue 1995) or in instances of metastasis to bones, e.g. mammary or prostatic tumours. It can be used to relieve physical obstruction by shrinking down tumours to alleviate pain and distress, e.g. metastatic anal sac adenocarcinoma to regional lymph nodes or large inoperable primaries (Figure 7.3). In many cases it is the only treatment modality for large inoperable brain tumours and for patients with granulomatous meningoencephalomyelitis (GME) that is non-responsive to medical management.
The majority of patients receiving radiotherapy are treated with megavoltage Linacs and are given a number of treatments over a period of weeks. The principle of fractionated radiation dose depends on a number of considerations that include the principles of radiation biology, the goal of treatment for the veterinary patient (this may be different from the goal in the human patient) and the access, cost, etc. of treatment. However, clients need to be informed of the various options for, and the benefits and risks of, these treatments.
The 4 ‘R’s’ of radiobiology
Not all tumours are equally radio-responsive, and this difference is primarily due to differences in tumour biology, i.e. the heterogeneity of tumours that includes growth fraction, hypoxic fraction, cell kinetics and the rate of cell loss. The principles are therefore designed to promote the death of tumour cells whilst minimizing the consequences to normal cells (Hall 2000).
Repair
Sublethal damage can be repaired. Fractionation allows normal cells to repair, but neoplastic cells do not have sufficient time to repair.
Repopulation
The regeneration of tissues depends on the recruitment of stem cells and this is slower in neoplastic tissue. Fractionated doses allow acutely responding normal tissues to regenerate and repopulate; however, if the treatment time is prolonged, then neoplastic cells will also repopulate.
Redistribution
Cells redistribute through the cell cycle and cells surviving a dose of radiation tend to synchronize in the resistant S phase of the cycle. Redistribution is particularly important in tumours with a low growth fraction.
Reoxygenation
Hypoxic cells are resistant to radiation. Reduction in the number of oxic cells after a cycle results in previously hypoxic cells receiving better oxygen supply and therefore becoming susceptible to radiation.
The question remains as to what is the optimal protocol to minimize damage to normal tissues and to maximize the ability to kill tumour cells. The answer to that question is unknown but over the past 20 years veterinary radiation oncology has made a number of advances along with human radiation oncology in achieving this goal. In human radiation oncology what is known as fine fractionated protocols have been developed that rely on small doses of radiation (200 cGy/treatment) given daily; in some cases multiple doses of radiation will be given the same day. These protocols are designed to maximize delivery of radiation to the tumour and minimize side effects to normal tissue. These protocols are still evolving.
In the 1920s the first veterinary radiotherapy protocols were developed by an Austrian veterinary surgeon, Alois Pommer, and his protocol of a few large fractions given over a short period of time was widely used for many years. This protocol is now known as accelerated hypofractionation. The main reason for developing such a protocol back then was concern about multiple anaesthesias and the cost. Almost 90 years later these protocols are still used and still have some application in veterinary oncology. In many cases, however, fine fractionation protocols are better suited to the overall control of neoplasia; smaller doses per fraction spare the late-responding tissues such as bone and allow larger total doses to be given (see Table 7.1). This was illustrated in one study that examined the late complications of irradiating the pelvic canal in dogs. Dogs that received lower dose/fraction 2.7 Gy instead of 3.3 Gy/fraction had fewer late side effects, in this case colitis, but overall the complication rate was low (Anderson et al 2002).
MWF, Monday, Wednesday and Friday; M–F, Monday to Friday. | |
Dose/fraction and interval between fractions | Total dose (Gy) |
---|---|
4 × 9 Gy weekly | 36 |
12 × 4 Gy MWF | 48 |
16 × 3 Gy MWF | 48 |
30 × 2 Gy M–F | 60 |
Fraction size limits total dose and, as can be seen from Table 7.1 and Table 7.2, many tumours require a total dose of around 50 Gy. To illustrate how fraction size impacts on normal tissue toxicity, heart failure can be induced by irradiating the whole heart to a total dose of 62–68 Gy using 2 Gy fractions, 60 Gy in 3 Gy fractions and 52 Gy in 4 Gy fractions.
++++, exquisitely sensitive (total dose <3 Gy); +++, sensitive (total dose up to 40 Gy); ++, moderately sensitive (total dose up to 50 Gy); +, relatively resistant (total dose up to 75 Gy). | ||
Tissue | Sensitivity to ionizing radiation | Injury |
---|---|---|
Bone marrow (whole body) | ++++ | Aplasia |
Bone marrow | +++ | Pancytopenia |
Liver | +++ | Hepatitis |
Lung | +++ | Acute respiratory distress syndrome |
Kidney | +++ | Acute/chronic renal failure |
Gastrointestinal tract | ++ | Ulcer |
Brain/spinal cord | ++ | Infarct |
Heart | + | Pancarditis |
Skeletal muscle | + | Myositis, fibrosis |
Bone | + | Necrosis, osteoporosis |
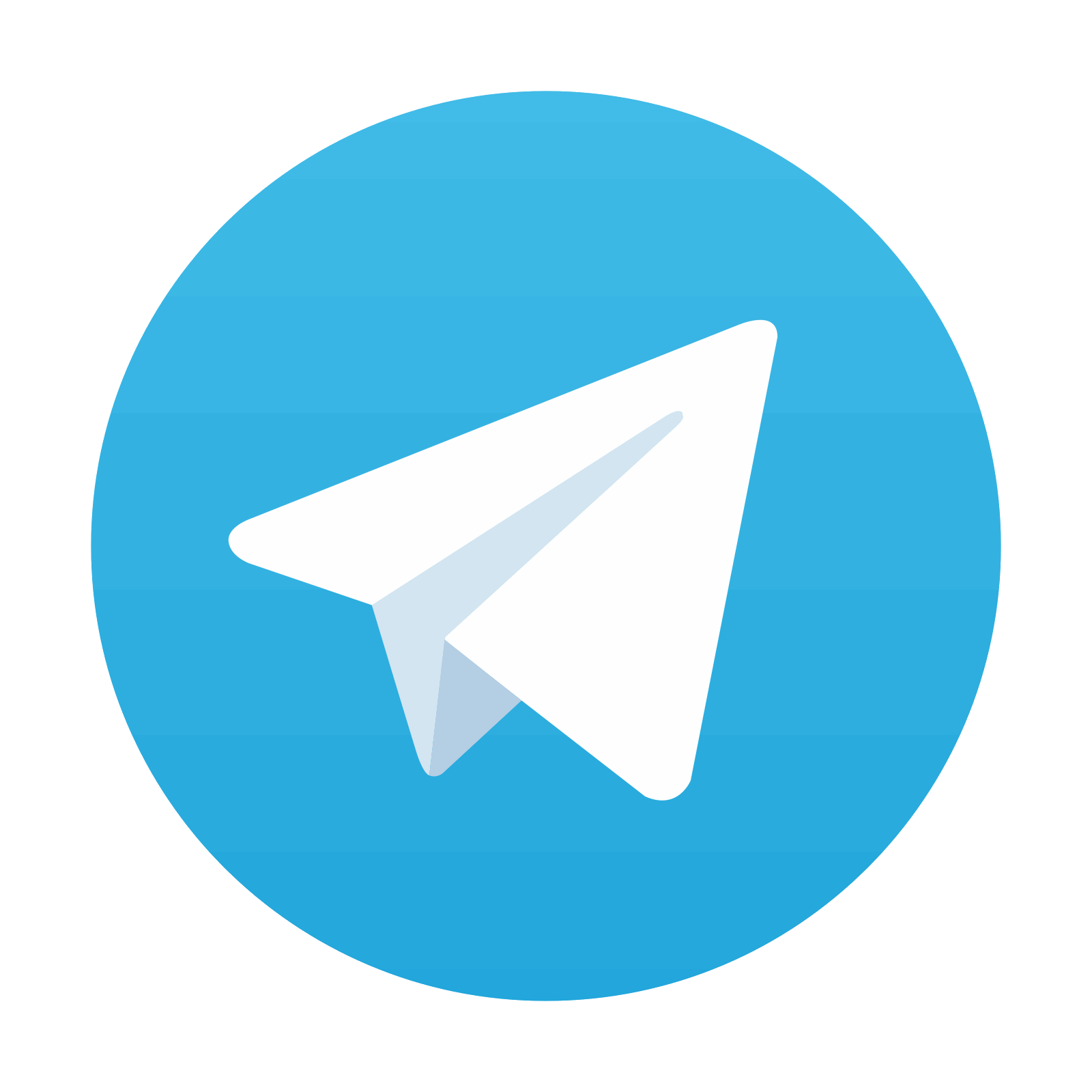
Stay updated, free articles. Join our Telegram channel

Full access? Get Clinical Tree
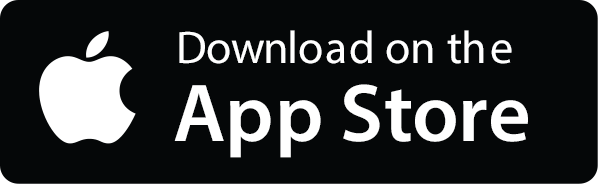
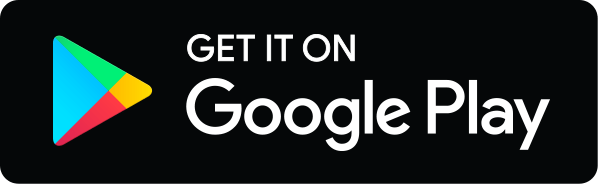