General principles of clinical nutrition
‘Simple’ or uncomplicated starvation
Simple starvation differs from the metabolism of the critically ill, because long periods of food deprivation can be tolerated by the body’s coping mechanisms, using hepatic glycogen, triglycerides within adipocytes and amino acids (skeletal muscle, visceral protein). Hepatic glycogen stores are depleted within 12 hours and glucose and insulin decrease initially. There is increased glucagon to stimulate glycogenolysis, and gluconeogenesis is also increased. As glucagon increases, lipolysis increases. This leads to increased glycerol, ketones and free fatty acids (FFAs). The basal metabolic rate (BMR) is downregulated to protect lean body mass. When glycogen sources are gone, amino acids are used for gluconeogenesis. Fat-derived fuels are used instead of glucose in tissues where this is metabolically possible (Mauldin & Davidson 2003).
‘Complicated/stressed’ starvation (metabolism of the critically ill)
Stressed starvation occurs when the body is in a hypermetabolic state as a result of a traumatic or inflammatory insult. Hypermetabolism compromises the normal metabolic adaptations to starvation that occur in simple starvation. Hormonal changes (increased catecholamine, cortisol, ACTH, glucagon, growth hormone) occur which lead to an increased BMR. An increased BMR, along with an increased need for clotting factors, acute phase proteins, white blood cells and other inflammatory components all increase the demand for glucose and amino acids to such a degree that lean body mass cannot be spared from catabolism, as it would be in simple starvation (Mauldin & Davidson 2003).
Nutritional support is required to prevent malnutrition and a compromised immune function, decreased wound healing, catabolism of lean body mass and likely a negative impact on overall survival (Chan & Freeman 2006). Often energy demands are not met without nutritional intervention, due to anorexia, decreased absorption, vomiting, etc. A high protein intake (up to 50%) is needed to provide the critically ill dog or cat with enough amino acids to stop catabolism of body protein (Donoghue 1989). This may be especially important in the cat, where a lack of amino acids may be responsible for impaired hepatic lipid release, leading to hepatic lipidosis. Fat is the main energy source for most tissues during complicated starvation, whereas carbohydrate may not be used with maximal efficiency due to persistent glucose intolerance and hyperglycaemia (mostly due to peripheral insulin resistance) (Mauldin & Davidson 2003).
In humans, providing nutrition decreases urinary nitrogen loss, stimulates immune function, reverses hypometabolism and provides sustenance during hypermetabolism. This improves recovery, lowers mortality and improves response to trauma and stress. The goal of providing nutrition in animals is to prevent catabolism of tissue proteins (Donoghue 1992). Enteral nutrition (assisted or voluntary) supports the integrity of the gastrointestinal tract (GIT) (Armstrong et al 1990, Robben et al 1999).
When to intervene to ensure adequate nutrition?
Nutritional support should be pre-empted (e.g. anticipated anorexia of more than 3–5 days, trauma, sepsis, peritonitis, pancreatitis, burns, large wounds, major gastrointestinal (GI) surgery, protracted vomiting, diarrhoea). Late signs of malnutrition are weight loss (>10%), poor hair coat, muscle wasting, hypoalbuminaemia, lymphopenia, coagulopathy and inadequate wound healing. Intervention such as placement of a feeding tube or parenteral nutrition should be given prior to these signs (Chan & Freeman 2006). Animals with hypoalbuminaemia are better treated with earlier intervention, as hypoalbuminaemia reflects a prolonged period of malnutrition (Withrow & Vail 2007).
What are the benefits of providing nutritional support to the cancer patient?
The benefits of dietary support in cancer patients include increased tolerance to surgery, chemotherapy and radiation therapy, improved immune function, immunoglobulin and complement levels, and phagocytic function of white blood cells (Ogilvie & Vail 1990).
Metabolic alterations that occur with cancer
Carbohydrate metabolism
Carbohydrate metabolism is significantly different in dogs with cancer; tumour cells preferentially metabolize glucose (carbohydrate) for energy and make lactate (lactic acid) as an end product. Lactate must then be converted back to glucose, which consumes energy and gives a net energy gain to the tumour and a net loss to the animal, contributing to cancer cachexia. Dogs with lymphoma and a wide variety of malignant diseases have elevated resting insulin and lactate levels compared to control animals. Elevated lactate and glucose levels may persist when dogs are rendered free of cancer with chemotherapy and surgery (Ogilvie & Marks 2000).
Protein metabolism
Tumours often use amino acids preferentially for energy via gluconeogenesis. If protein intake/absorption does not keep pace with use, a negative nitrogen balance occurs. This is seen clinically as muscle wasting, decreased immune function and GI function, and poor wound healing. Decreased body muscle mass, decreased skeletal protein synthesis, increased skeletal protein breakdown and increased liver and whole body protein synthesis all occur to support tumour growth (Ogilvie 2001).
Fat metabolism
Tumour cells have a limited ability to use fat for energy, compared to carbohydrates and protein. Dogs with cancer therefore burn their fat reserves as an energy source. Loss of body fat accounts for the majority of weight loss in people and rodents with cancer cachexia. The type of fat in the food is important, and omega-3 fatty acids have many clinical benefits (Ogilvie 2001, Ogilvie & Marks 2000).
Dogs with cancer without weight loss
In the authors’ experience, these dogs form the majority of patients seen in referral veterinary oncology practice, perhaps because many die of their cancer before they become cachectic. Dogs with cancer and no weight loss still show the alterations in protein, carbohydrate and fat metabolism as described above, without any evidence of clinical signs (Ogilvie 2001). Several studies have found no significant differences in resting energy expenditure (and presumably caloric requirements) in dogs with a wide range of malignancies when compared with healthy, client-owned dogs. This finding suggests that, in general, dogs with cancer and no evidence of weight loss do not have energy requirements higher than those of apparently healthy dogs without cancer (Ogilvie 2001). However, the source of their dietary energy may be more critical than their net energy requirements.
Dogs with cancer and weight loss
The first clinical signs of these metabolic alterations are anorexia, mild weight loss, lethargy and an increased susceptibility to side effects of chemotherapy, radiation therapy, surgery, etc. (Ogilvie 2001). A prolonged state of metabolic derangement resulting in accelerated starvation and profound malnourishment is termed ‘cancer cachexia’. Decreased nutritional intake/assimilation, abnormal nutrient losses, treatment-related factors, abnormal metabolic pathways and competition with the tumour for nutrients all contribute. End-stage cancer cachexia is characterized by marked muscle wasting, weight loss, debilitation, weakness, loss of body fat stores and lethargy (Ogilvie & Marks 2000) (Figure 12.1).
![]() |
Figure 12.1 |
In the authors’ experience, advanced cancer cachexia is not seen commonly. When it has been seen, it has tended to affect dogs with a significant ongoing, progressive, uncontrolled and often end-stage tumour burden. Overt cancer cachexia is a poor prognostic sign, as patients have a decreased quality of life, decreased response to therapy and shortened survival time compared to those with similar diseases without cachexia (Ogilvie 2001). Dogs with cancer may develop cachexia despite a nutritional intake considered adequate for a normal dog (Ogilvie & Vail 1990).
What to feed the cancer patient
The finding that tumour cells tend to use carbohydrate and protein preferentially to lipid for fuel has led to the hypothesis that feeding foods relatively high in fat may be more beneficial than feeding carbohydrate-rich foods. This diet is then designed to ‘feed the dog and starve the tumour’. Foods containing less than 30% protein calories and more than 40% carbohydrate calories should be avoided in cancer patients (most commercial foods are suboptimal in this regard) (Ogilvie & Marks 2000).
Carbohydrates
Carbohydrates may be poorly used due to peripheral insulin resistance. Foods high in carbohydrates may increase the total amount of lactate produced and the need for the dog to use energy to convert lactate back to glucose. Soluble carbohydrates should comprise less than 25% of the food’s dry matter (or <20% of the daily energy requirement, DER) (Ogilvie & Marks 2000).
Fat
A large proportion of the daily energy should come from fat in the diet because tumour cells have difficulty using fat compared to carbohydrate and protein, and loss of body fat contributes to weight loss and cachexia. Fat should comprise 25–40% of the food’s dry matter (50–65% of the DER) (Ogilvie & Marks 2000).
Omega-3 (n-3) fatty acids
Increased levels of dietary n-3 fatty acids may benefit the cancer patient, according to studies that link n-3 fatty acids to tumour inhibition and immune enhancement. N-3 fatty acids have this selective tumoricidal action without harming normal cells. As they also decrease protein degradation without altering protein synthesis, the net effect is anti-cachectic. Dietary levels should be in excess of 5% of the food’s dry matter, with an n-6 : n-3 ratio of <3.0 (Ogilvie & Marks 2000).
Omega-6 (n-6) fatty acids
Metastases are enhanced with omega-6 (n-6) fatty acids (Ogilvie & Marks 2000).
Protein
This is used to build body protein (muscles) and for immune function. Dietary protein should be fed at greater levels than those required in a normal dog to help prevent a loss of muscle mass. Protein should comprise 30–45% of the food’s dry matter in dogs and 40–50% in cats (25–40% of the DER in dogs and 35–45% of the DER in cats) (Ogilvie & Marks 2000).
Amino acids
Methionine
If methionine is replaced with its precursor homocysteine, methionine-dependent tumour cells are locked into late S and G2 phases of the cell cycle, which increases the percentage of tumour cells sensitive to chemotherapy (Ogilvie & Marks 2000).
Asparagine
Asparagine is essential for tumour growth in lymphoma. Treatment of dogs and cats with l-asparaginase (which breaks down asparagine) has induced complete (but short-term) remissions in up to 80% of dogs and cats with lymphoma (Ogilvie & Marks 2000).
Tyrosine and phenylalanine
Restriction of these amino acids has been reported to suppress melanoma growth in tissue cultures and rodents (Ogilvie & Marks 2000).
Arginine
Adding arginine to total parenteral nutrition solutions decreases tumour growth and metastatic rate in rodent cancer models. Arginine is an essential amino acid for cats and conditionally essential for dogs. Arginine given in large doses exerts numerous beneficial effects on the immune system, particularly on thymus-dependent and T-cell-dependent immune reactions. Arginine should comprise >2% of the dry matter of the food (Ogilvie & Marks 2000).
Glutamine
Glutamine is an essential precursor to nucleotide biosynthesis and is the most important oxidative fuel for enterocytes (Ogilvie & Marks 2000).
Vitamins
Retinoids
The synthetic retinoids isotretinoin and etretinate (vitamin A derivatives) have been therapeutic in some dogs with intracutaneous cornifying epitheliomas, other benign skin tumours, cutaneous lymphoma, solar-induced squamous cell carcinoma and associated preneoplastic lesions. The retinoids promote cellular differentiation and may enhance the susceptibility of neoplastic cells to chemotherapy and radiation therapy (Ogilvie & Marks 2000).
Vitamin C
Vitamin C may be therapeutic as an antioxidant, as well as an inhibitor of nitrosation reactions (nitrosamines have carcinogenic capabilities), and for overcoming drug resistance (e.g. vincristine) in some cancer cells. However, few direct data exist proving its efficacy (Ogilvie & Marks 2000).
Vitamin E
Vitamin E (α-tocopherol) is an antioxidant (free radical scavenger) with a broad capacity to inhibit mammary tumour carcinogenesis and colon carcinogenesis in rodents. Vitamin E may normalize the immune system by interacting with macrophages and T lymphocytes to inhibit retroviral-induced infections and retroviral-induced tumorigenesis (Ogilvie & Marks 2000).
Minerals
Selenium
Low selenium levels have been observed in humans with GI cancer. In rodents, dietary supplementation with selenium inhibits colon, mammary gland and stomach carcinogenesis (Ogilvie & Marks 2000).
Iron
Mice with low levels of iron have slower tumour growth than those with normal iron levels. Lung, colon, bladder and oesophageal cancer in people have been highly correlated with increased serum iron levels and increased transferrin saturation (Ogilvie & Marks 2000).
Zinc
Low levels of zinc in blood and diseased tissue have been observed in human oesophageal, pancreatic and bronchial cancer. Zinc deficiency enhances carcinogenesis in rodents (Ogilvie & Marks 2000).
Novel foods
Soybean-derived Bowman-Birk inhibitor
This can inhibit carcinogenesis in vivo and in vitro, and is also associated with increased intestinal integrity in cats given methotrexate (Ogilvie & Marks 2000).
Garlic
Epidemiological studies correlate high garlic consumption with reduced cancer risk, although no studies demonstrate the safety or effectiveness of garlic for prevention or treatment of cancer in dogs, cats or people. There is evidence that garlic has activity against cultured human breast cancer and melanoma cells, without harming normal cells (Ogilvie & Marks 2000).
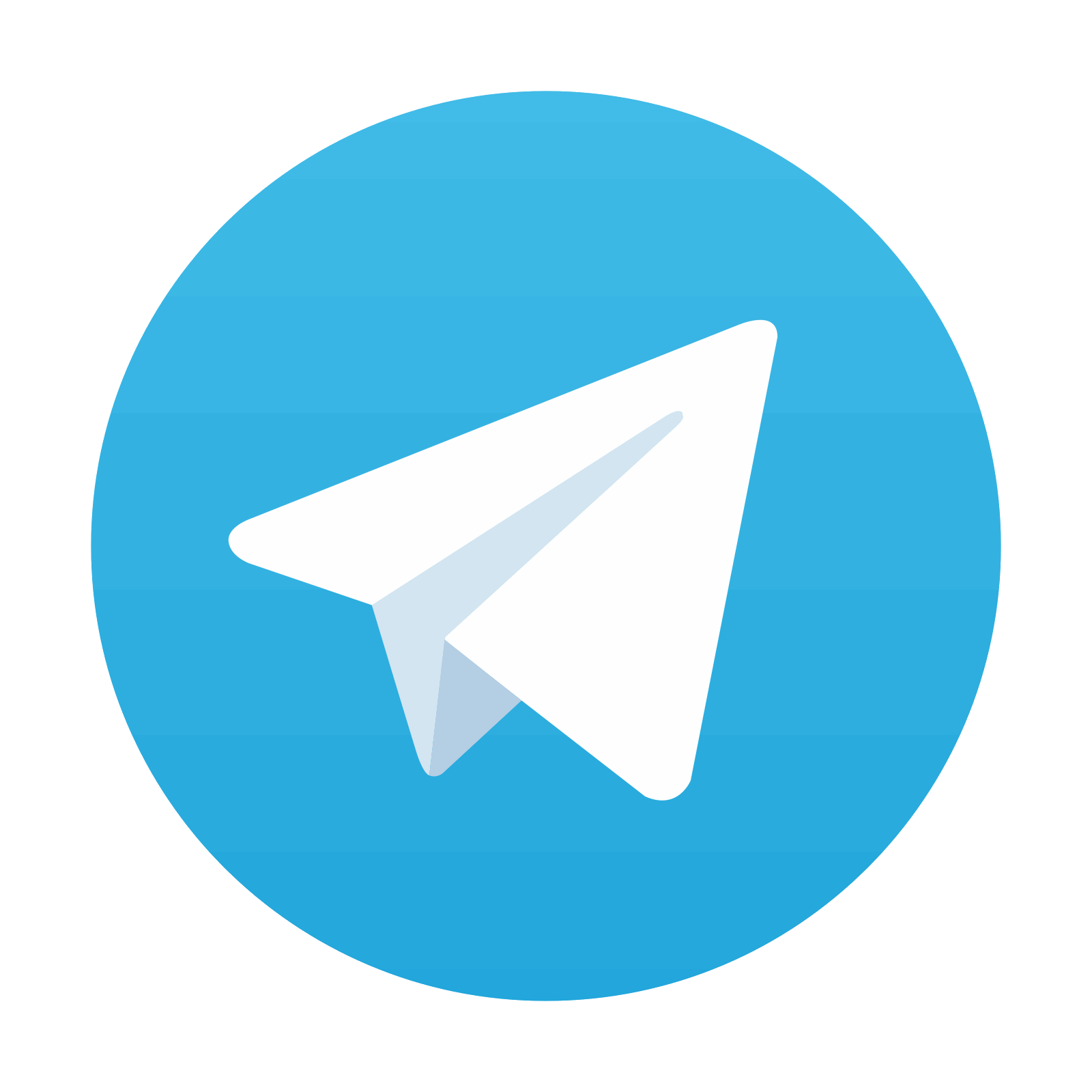
Stay updated, free articles. Join our Telegram channel

Full access? Get Clinical Tree
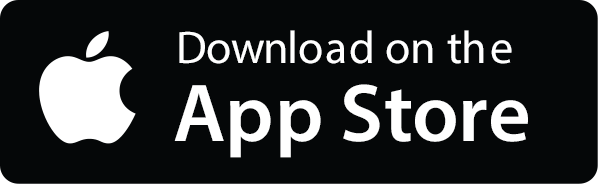
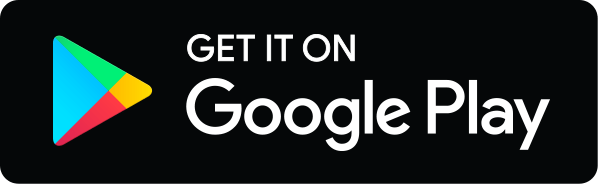