Clinically important abnormalities have been observed by microscopy of urinary sediment in human medicine for over 400 years[1, 2]. Microscopic examination of the urine sediment should always be performed as part of the complete urinalysis, especially in animals that are sick and those that have abnormal color or clarity, abnormalities detected on chemical dipstrip testing (see Chapter 5), lower than expected urine specific gravity (USG) (Chapter 4), or on physical examination findings. It is preferable to perform the complete urinalysis in‐house, though urine microscopy was considered optional by one veterinary author if the urinary chemistry reagent pads were all normal and there were no abnormalities in color or clarity of the urine specimen [3]. Unfortunately, urinary microscopy is often omitted in veterinary practice, which can result in failure to identify important diagnostic information. White blood cells (WBC), bacteria, fungal organisms, epithelial cells, crystals, and casts are not detected using dry‐reagent strip pad analysis. Failure to perform urine sediment microscopy can be attributed to the perception that it takes too much time (approximately 20 minutes to perform a complete urinalysis) and concern about the skill level and experience necessary for in‐clinic technicians to proficiently perform a urinalysis [4]. In veterinary medicine, standard manual urine microscopy has a within‐run coefficient of variation (CV) of 30–40% for red blood cells (RBC) and WBC [5]. How the urine is mixed, what volume is centrifuged, the force of centrifugation, and the volume of sediment examined all should be standardized to obtain repeatable and accurate results. Urine sediment examination has been referred to as the “liquid kidney biopsy” [6], but it also often reflects disorders of the lower urinary tract, so the moniker “liquid urinary tract biopsy” may be more apt. Macroscopic screening of urine based on its physical and chemical properties is sometimes used in human medicine to decide if urine sediment examination will be cost‐effective [7]. Abnormal elements in urine sediment are more likely to be found during microscopy when urine has an abnormal color (colorless, very dark yellow, red, brown), if the urine is cloudy, if the USG is lower than normal, if USG is extremely high, or if the reagent pads on the dry reagent strip pad are positive for abnormalities (e.g. blood, protein, glucose). In human medicine, the leukocyte esterase test pad is valuable to prompt microscopic sediment evaluation, but this reaction is not useful in veterinary medicine (see Chapter 5). In human medicine, the need for trained specialists to perform urine sediment examination as a biomarker for kidney disease continues to be emphasized, especially in patients with acute kidney injury (AKI) [8]. Nephrologists examining urine sediment are more likely to identify clinically important findings as compared to laboratory technicians in some disease settings [9]. Some believe that the best doctors examine fresh urine sediment themselves during evaluation of sick patients and, thus, gain immediate information that can be clinically useful [9, 10]. If the urine is of normal color and clear, and the chemical reagent dipstrip chemistry reactions are all normal, results of microscopic examination of the urine sediment will be normal in most instances [3, 11]. The likelihood that urine sediment examination will disclose abnormalities in urine samples from apparently healthy dogs and cats (i.e. normal history and physical examination) is low. Approximately 3% of dogs without a history of genitourinary disease had no macroscopic urine abnormalities but had abnormal urine sediment findings (i.e. were falsely negative on macroscopic screening) [12]. In another study, failure to examine the urine sediment of macroscopically normal urine samples would have yielded false‐negative results in 16.5% of the dogs and 5.7% of the cats [13]. All animals with clinical signs or physical examination findings compatible with disease of the genitourinary tract should have a complete urinalysis that includes urine sediment examination even if macroscopic screening is normal. Animals that are sick at initial presentation in general practice, at referral to specialty practice, or during examination at emergency presentation are more likely to have abnormal findings on urine sediment examination, even in the absence of abnormal color, clarity, or urine concentration. It is our impression that patients that have received intravenous fluids and drugs during hospitalization are more likely to have abnormal findings in their urine sediment after suspected and unsuspected renal damage (casts and renal tubular epithelium [RTE]). Abnormal urine sediment from sick animals can include increased numbers of RBC, WBC, epithelial cells, casts, infectious agents (bacterial and fungal), parasite ova or larvae (Capillaria plica, Dioctophyma renale, Dirofilaria immitis), and crystals. Reagent pads do not detect epithelial cells, WBC, casts, infectious agents, parasites, or crystals. Procedures for processing and evaluating urine sediment vary by laboratory, which likely contributes to differences in numbers and types of elements reported. A surprising lack of standardization in the evaluation of urine sediment also exists in human medicine [14]. An outline for the preparation of urinary sediment for microscopy is presented in Table 6.1. Reasons for errors in reporting elements in urinary sediment are outlined in Table 6.2. Prompt examination of fresh urine (at body temperature) is recommended to maximize the ability to properly identify abnormal elements in the urine and to minimize artifacts that develop over time in some samples. Ideally, urine sediment should be examined at room temperature within 30–60 minutes after collection [15, 16]. Microscopic examination of very fresh urine favors preservation of fragile cellular elements and minimizes chances for cellular degeneration, which enhances identification of abnormal elements in the sediment. Up to 12 hours of refrigeration before analysis has traditionally been considered acceptable, but we recommend less than four hours of refrigeration before urine sediment examination if possible. Refrigeration of urine for up to eight hours before performing microscopy likely compromises the ability to accurately identify RBC casts, dysmorphic RBC, and other formed elements because they may be degraded with time and refrigeration [9]. In sick or critically ill patients, it is best to examine the urine sediment within 15 minutes of collection to increase the likelihood of identifying and enumerating cellular casts and RTE. Cooling and storage of urine favor crystal precipitation, cellular degeneration, and dissolution or disintegration of casts. The degradation of urinary elements is more likely in alkaline and dilute urine samples [17], a process that may be particularly important for casts [18]. Warming a stored urine sample to room temperature does not necessarily result in the dissolution of all crystals that formed as artifacts during cooling of the sample. A first morning sample, collected before emptying the bladder by voiding, may contain more formed elements as a consequence of the longer time of urine formation overnight, but cellular detail may be lacking because of longer residence time in the bladder that could allow degeneration of elements before urine sediment examination. In some situations, it may be advantageous to examine a urine sample that has been collected after the first voiding in the morning so as to allow better cellular detail to be observed. In human medicine, more accurate identification and classification of urinary elements (including casts) is made possible by analysis of the second morning specimen. This approach decreases the likelihood of lysis or degeneration of urinary elements that might otherwise occur in urine that has remained in the bladder overnight [18]. Table 6.1 Preparing urinary sediment for microscopy. This is one standardized protocol for urinary microscopy using 1 mL of well‐mixed urine that undergoes manual centrifugation and microscopy. This protocol is used by some veterinary laboratories. Alternatively, a larger volume (e.g. 3 mL or 5 mL) of well‐mixed urine could be used in which 80% of the supernatant is removed, and the sediment is then resuspended in the remaining 20% of the initial volume. Standardization of all aspects of setting up the slide for urinary microscopy will improve the quality and accuracy of the results that are generated and available for interpretation. This includes the volume of urine analyzed, the volume of supernatant used to resuspend the sediment, centrifuge tube size and shape, plastic bulb pipettes, microscope glass slides and coverslips, and the same high‐quality microscope. Preservatives do not need to be added to urine samples when urine sediment examination can be performed within a few hours of collection. When urine cannot be examined soon after collection, the sample should be refrigerated to minimize degeneration of elements in the urine sediment and to inhibit bacterial multiplication. WBC, casts, epithelial cells, and bacteria are well preserved when boric acid alone or boric acid in combination with formic acid has been added to the urine sample container, but RBC may become smaller and unstable. One drop of formalin may be added per 30 mL of urine to preserve urine sediment [19]. Preservatives can also alter the chemical dry reagent strip pad reactions. When used, lyophilized preservatives in containers are preferred so that urine dilution does not occur [17, 20]. The beneficial or damaging effects of routinely adding preservatives to urine samples before analysis have been recently evaluated in veterinary medicine (see later). Table 6.2 Reasons for inaccurate enumeration or failure to replicate reported numbers of elements per HPF, LPF, and μL. When using preservatives to send urine samples to a reference laboratory, we recommend dividing the initial urine sample into two aliquots. The aliquot with preservative is used for urine sediment examination, and the second aliquot without preservative is used for urine dry reagent strip pad chemical analysis. Alternatively, for confusing or complicated cases, an unstained dry‐mount preparation of urine sediment can be sent along with a urine sample in ethylenediaminetetraacetic acid to the reference laboratory [21]. The dry mount is prepared in the usual manner as for cytology; it does not involve allowing the wet mount to dry. The dry mount preparation using fresh urine sediment will allow identification of RBC, WBC, epithelial cells, and bacteria (cocci, cocci in chains, short rods, coccobacilli) that have not undergone degeneration, but crystals and casts usually do not adhere to the slide and, thus, cannot be evaluated using routine techniques. A technique that employs cytocentrifugation of fresh urine followed by Papanicolaou staining has been described and allows permanent dry mount slides to be prepared for evaluation of urine casts. The evaluation of such slides can allow more precise identification and enumeration of casts, especially cellular casts [22]. Thorough mixing of urine before centrifugation is essential in order to avoid a preanalytical error, in which important elements are not included in the sample transferred to the centrifuge tube, as a consequence of elements falling by gravity to the bottom of the container. The effect on the recovery of various urinary elements varies by time for urine mixing, time elapsed for aspiration after mixing, and the location in the tube from which the sample is of aspirated (top, middle, or bottom), but these aspects of sample handling have not been standardized in veterinary medicine [23]. One study determined that the lowest crystal counts were observed when more settling time was allowed before aspiration of the urine sample and when urine was aspirated from a higher position in the tube. Sinking of urinary elements by gravity apparently occurs more rapidly than generally appreciated. A mathematical model was developed that predicted noncrystalline elements to settle at 1 μm per second and crystals to settle at 10–60 μm per second, depending on crystal size and density. Based on these observations, sample aspiration immediately after mixing is necessary to ensure consistent and accurate reporting of urinary elements [23]. After mixing, the sample should be aspirated from a position near the bottom of the container to ensure that a representative urine sample is obtained. Traditionally, a standardized volume of urine is gently centrifuged in a conical tube (Figure 6.1). Glass centrifuge tubes were not recommended for urine sedimentation in one report, since cells and casts were noted to readily adhere to glass [24]. The accepted rationale for the use of centrifugation is that this procedure concentrates or amplifies the detection of small numbers of elements in urine that would otherwise not be observed. The examined volume usually is 10 or 15 mL in human medicine [7], but this volume is not consistently available for urine samples collected from dogs and cats. Although a minimum of 5 mL is recommended in veterinary medicine [25, 26], a sample size of 1–3 mL of urine is routinely examined by some veterinary laboratories. Although it seems intuitive that a larger volume of urine would allow more elements in urinary sediment to be concentrated, retrieved, and identified, this assumption has recently been challenged (see below). It has been considered important to centrifuge the same sample volume in all patients so as to compare quantitative results in a consistent manner. Corrected enumeration of urine sediment elements (based on multiplication factors) are reported by some human medical laboratories depending on the volume that is centrifuged and the volume of sediment examined [7], but such corrections are not made by veterinary laboratories. FIGURE 6.1 A standard volume of urine (often 1–3 mL) is placed into a conical centrifuge tube. Following centrifugation, the tube is inverted, and the supernatant is drained into a second tube and saved for further evaluation if needed (e.g. urinary protein‐to‐creatinine ratio or electrolyte chemistry). There may or may not be anything visible at the bottom of the tube following centrifugation. Sediment can still be present in the bottom of the tube even when there is no visible evidence to the naked eye. Following decanting and repositioning of the tube to a vertical position, sediment will be resuspended in a small volume of urine lining the side of the tube. Excessive force can disrupt fragile elements during centrifugation, especially casts. Debris and free RBC, WBC, or nonsquamous epithelial cells that are identified may appear following disintegration of casts. The hemolysis of RBC in urine will cause under‐reporting of RBC numbers. Traumatic handling of the sample can cause rupture of nucleated cells that also results in under‐reporting of WBC and epithelial cells. Too much force can also cause crystals to aggregate and appear less numerous than when nonaggregated. Similarly, too much force can cause clumps of WBC and RBC that can be difficult to evaluate and may be confused with casts. A relative centrifugal force (RCF) of less than 400 appears to be optimal to generate sediment without damaging urinary elements [7]. The use of RCF for a specific centrifuge is preferred to revolutions per minute (rpm) because the radius of the centrifuge rotor also contributes to force. Some centrifuge manuals provide both rpm and RCF. RCF is used interchangeably with gravity force (g). The following formula can be used to convert rpm to RCF: RCF = 1.118 × 10−5 × rotor radius (cm) × rpm2 [7]. The use of centrifuges at settings used to separate serum and plasma exert too high an RCF to be useful for urine sediment. Braking of the centrifuge should not be used since doing so can cause turbulence and resuspension of lighter elements into the supernatant, which will result in less enumeration of urinary elements during sediment microscopy. The force from braking also has the potential to disrupt fragile elements in the urine such as casts (Figure 6.2) [27]. Commercial veterinary laboratories vary in the techniques they use to evaluate urine sediment. At one commercial veterinary reference laboratory (IDEXX), a small volume (60 μL) of mixed urine is placed into one well of a multiple well plate so that a technician using an inverted microscope can easily read urine sediments from different patients (Figure 6.3). Neither centrifugation nor coverslips are used with this technique, because the urine sample sits in the well at 1 × g for at least 10 minutes to allow sedimentation before microscopy [28]. Another commercial laboratory (ANTECH Diagnostics) centrifuges 2–3 mL of urine at 400 × g, after which the supernatant is decanted and the sediment resuspended for wet mount microscopy. Further discussion of microscopy results using different methods to prepare urine for microscopy is provided below. FIGURE 6.2 A centrifuge capable of lower force (radius × RPM) is selected for urine sediment preparation so that delicate elements in urine are not damaged. The brake function on the centrifuge should not be used; otherwise, fragile elements may be disrupted or resuspended in the supernatant. FIGURE 6.3 Multiple well plate on the microscopic stage of the inverted microscope. Sediment accumulates by gravity and not centrifugation. Light is shining through each individual well of urine sediment sequentially for evaluation under high‐ and low‐powered lens objectives below. Source: Courtesy of IDEXX Laboratories. Copyright © 2022, IDEXX Laboratories, Inc. All rights reserved. Used with permission. More WBC, RBC, squamous epithelial cells, and nonhyaline casts were identified and enumerated using a modified technique for analysis of urinary sediment compared to a “standard” technique in one study from human medicine. The modified technique employed centrifugation of 10 mL urine in round bottom tubes at 1358 × g, and decanting of the supernatant, compared to centrifugation of 5 mL of urine in conical tubes at 400 × g, with aspiration of the supernatant [29]. In an early report using urine samples from dogs, three methods of urine handling were used to compare the enumeration of urinary elements during urine sediment examination after centrifugation. Results were compared among large volume samples handled with low force (5 mL at 400 × g for 5 minutes), small volume samples handled with low force (1.5 mL at 250 × g for 5 minutes), and low volume samples handled with high force (1.5 mL at 3900 × g for 45 seconds; Statspin®). Similar results for numbers of cells, casts, and crystals were noted, but the samples used did not contain sufficient numbers of casts or crystals to perform a meaningful statistical evaluation. Similar studies of this type using urine samples with larger numbers of casts should be performed [30]. In a more recent study, four different methods of preparing canine urine for microscopy were evaluated using urine with added RBC and compared centrifuge force and time of centrifugation. High volume low force (5 mL at 390 × g for 5 minutes), low volume high force (1.5 mL at 3900 × g for 45 seconds), 60 μL in a microtiter plate well with no centrifugation, and 30 μL on a microscope slide with coverslip were examined and compared. The microtiter plate performed best with a CV of 14%, and the 1.5 mL 3900 × g centrifugation method performed worst with a CV of 71% for RBC. No so‐called amplification benefit of centrifugation was identified, whereas substantial variability in results for all of these methods used for microscopic examination was found [28]. A standard volume of urine is chosen for centrifugation based on the laboratory standard operating procedure (SOP). Textbooks often mention to add 5–10 mL of well‐mixed urine to the centrifuge tube, but far smaller volumes of urine are often provided to the laboratory from dogs and cats. Following urine sedimentation by gravity or centrifugation during manual analysis, the supernatant is poured off (decanted) or aspirated by dropper or pipette, and the supernatant can be submitted for other required analyses (e.g. urine protein/creatinine ratio) if necessary. The sediment must be resuspended before microscopy to ensure equal distribution of elements in the sediment. The sediment is diluted by a variable fluid volume lining the tube (estimated at 0.2–0.4 mL) following decanting, which contributes to variations in reports of the number of elements per low‐power field (LPF) or high‐power field (HPF). Similarly, the urine sediment is also diluted to a variable degree when liquid stain is added to the tube. Resuspension can be accomplished by gentle aspiration into a pipette or by tapping gently on the tube using the index finger. Vigorous agitation should be avoided because it can damage some elements in the sediment. It is recommended to remove 80% of the supernatant following centrifugation, followed by resuspension of the sediment in the remaining 20% of the supernatant in an effort to standardize the degree of sediment dilution (Dr. Jessica Hokamp, personal communication, 2020). Manual microscopy has been the gold standard to which automated microscopy is compared, but there can be considerable operator variability in the identification of findings in urine sediment. The amount of interobserver variability (imprecision) in the evaluation of urinary sediment raises concern about the reliability of this method as the gold standard [31]. The level of agreement in the identification of casts among human nephrologists was 59% in one study; an estimated level of agreement for other findings in urinary sediment was 69% [32]. Casts were not accurately identified by community laboratories in one study; there was minor variation in casts detected by nephrologists in the same study [33]. In another study, only slight‐to‐moderate agreement for the identification of urinary sediment elements was found by nephrologists during evaluation of digital images; agreement was not associated with seniority [34]. An excellent core curriculum on urinalysis that emphasizes urinary sediment examination in human medicine is available for the interested reader [35, 36]. A detailed technical review of morphological findings from human urine microscopy is also available with many high‐quality images of urinary elements [37]. Automated methods for urine chemistry and urine microscopy have become common at centralized laboratories in human medicine; a trend has resulted in a decreased number of urinary sediment evaluations by nephrologists. There is still value in nephrologist‐performed urine microscopy, especially in those with AKI in which valuable information is provided that is not typically generated from automated urinalysis by laboratory technicians. The gold standard in the evaluation of human renal patients is still regarded by some to be the performance of manual microscopy by a highly trained and motivated examiner [35, 36]. Routine microscopy of urine sediment employs bright‐field microscopy, but phase microscopy can be helpful to facilitate the identification of cells, casts, and bacteria [18, 38]. Polarizing light microscopy can further aid in the identification of crystals [39]. Elements in the urine sediment can be reported as number per mL or number per HPF (40× objective) or LPF (10× objective). Most veterinary laboratories report using the number of elements per high‐ or low‐power microscopic field. To report as numbers per milliliter, the number of milliliters centrifuged divided by the milliliter of sediment volume evaluated equals the concentration factor [7]. It is best to record all the observations on a standard form for inclusion in the medical record (Figure 6.4). Many experienced laboratory technicians prefer to evaluate unstained urinary sediment, but the added cellular detail obtained using supravital stains (e.g. Sternheimer‐Malbin, Sedi‐Stain™, KOVA®, new methylene blue) can facilitate proper identification of cells in a wet mount preparation [40–45], especially for the less experienced observer. There are advantages of examining urine sediment with and without stain (Table 6.3). The use of supravital stain for urine sediment examination enhances the examiner’s ability to properly identify RTE, casts, and WBC [45]. Stains are designed to change the refractive index of cells and provide nuclear contrast. Urine sediment that has been stained currently cannot be used in automated systems for sediment examination because stain disrupts the recognition algorithms that allow the identification of cell borders. For conventional microscopy, stain is added at a volume equal to the volume of sediment remaining in the bottom of the tube after centrifugation (usually one to two drops) (Figure 6.5), with gentle mixing (Figure 6.6). Doing so adds a dilutional factor that ideally should be considered in how many elements per microscopic field are reported. When stain is used, it is recommended that an unstained portion of the initial sediment be examined at the same time. Both stained and unstained sediment can be examined side by side on the same slide using two coverslips [15]. Enumeration of elements should be determined on the unstained preparation, whereas definitive identification of elements can be made on the stained preparation. Precipitates of stain sometimes can be observed during sediment examination if outdated stain is used. Bacterial and fungal elements sometimes are present as contaminants in the urinary stain [3]. Sedi‐Stain and KOVA contain stabilizing agents to prevent precipitation that occurred with the original Sternheimer‐Malbin stain. Staining of mucus sometimes can result in confusion with casts. Punctate forms that resemble cocci sometimes are seen as an artifact when stain is used for examination of feline urine sediment. Special stains that can be used include 0.5% toluidine blue to enhance nuclear detail (metachromatic) and Oil Red O or Sudan stain to identify triglycerides or neutral fats. The combination of negative lipid staining and positive polarization (Maltese cross appearance) provides supporting evidence to identify cholesterol in urine sediment [7, 46]. FIGURE 6.4 Form for recording observations from microscopic sediment evaluation and expected results from normal dogs and cats. Table 6.3 Advantages and disadvantages for staining or not staining urine for microscopy. FIGURE 6.5 One to two drops of supravital stain (Sternheimer‐Malbin; Sedi‐Stain) are added to match the volume of the sediment in the bottom of the tube (optional). A drop of unstained sediment can be removed at this time instead of adding stain, or this can be used in addition to the stained sediment. The unstained sediment can be examined as described further below or can be submitted for dry‐mount cytology if needed. A consistent volume (one drop or 50 μL) of resuspended urine sediment (Figure 6.7) is transferred to a microscope slide using a pipette and a coverslip is applied (22 × 22 mm) [31] (Figure 6.8). A single view during LPF microscopy (100× total magnification) evaluates 3.14 mm2 under the coverslip and 0.196 mm2 during HPF microscopy (400× total magnification). This corresponds to 7.27 μL of uncentrifuged urine during LPF and 0.45 μL during HPF microscopy [37]. An excessively large drop that spreads beyond the coverslip has the potential to carry heavier elements beyond the cover slip and prevent their identification [7] and also promotes distracting Brownian motion of urinary elements. Care should also be taken to avoid including air bubbles during the placement of the coverslip. Likewise, care is also taken to ensure that immersion oil does not contaminate the slide and create oil droplets. We recommend waiting for approximately 90 seconds before starting microscopy to allow urinary sediment and artifacts to cease Brownian motion and achieve the proper plane for examination (Figure 6.9). FIGURE 6.6 The sediment and stain are mixed by gentle tapping or agitation of the tube. FIGURE 6.7 Urinary sediment, stained or unstained, is gently aspirated by glass or plastic pipette. In this example, a glass pipette is used to collect urine sediment by capillary action. Wet mount microscopy necessitates the use of different techniques for placement of the microscope condenser and the intensity of the lighting compared to dry mount bright‐field microscopy used for blood films and cytology where the light is in focus with the plane of focus for the microscopic specimen. Because the optical density of urine is very close to that of some of the elements in the urine sediment, the microscope lighting should be out of focus to render the formed elements as refractile as possible, which, in turn, will optimize their identification. Adjusting or “rocking” the fine focus of the microscope up and down can facilitate the examination of cellular elements in the sediment. Several other techniques can be used to improve microscopic examination of the urine sediment: The light intensity can be decreased, the iris diaphragm slightly closed, and the condenser slightly lowered. These adjustments should be made while microscopically viewing the urine sediment to identify the optimal settings for the specimen being evaluated. FIGURE 6.8 One drop of aspirated urine sediment is added to a glass microscope slide and a coverslip applied. Care is taken to not overfill the area under the coverslip and to avoid the introduction of air bubbles. FIGURE 6.9 The coverslip has been applied. We recommend allowing the sediment to settle to the same level on the slide for about 90 seconds before starting microscopy. Smaller coverslips are used by some laboratories. Larger cover slips can allow for more fields to be examined when there is suspicion for small number of abnormal elements. The numbers of elements reported during urine sediment examination of normal dogs and cats are affected by the extent of trauma to the urinary tract and the amount of contamination from the environment or genitourinary tract that occur depending on the method of urine collection that is used (e.g. initial stream voided, midstream voided, endstream voided, catheterized, cystocentesis, from the floor or table top (see Chapter 3)). Urinary elements can be retained on items from which the urine sample is collected (e.g. cage pads, diapers), which potentially can lead to under‐reporting of some elements (e.g. casts). Such collection methods also may add artifacts such as fibers to the sediment [47]. The average number of elements observed is counted in 10 HPF (400×) for RBC, WBC, epithelial cells, crystals, and bacteria. Casts are counted per LPF (100×) but further evaluated and the type characterized at HPF (as described below). Clumps of WBC and epithelial cells should be specifically reported (as described below). Bacteria should be reported as cocci, rods, or mixed populations, and bacteria in chains or those engulfed by WBC should be noted. The margins of the cover slip should be examined carefully because heavier elements such as casts often are found there. Sometimes, a large number of RBC (designated “too numerous to count”) dominate the urine sediment, making it impossible to identify other elements. In such instances, 2% acetic acid can be added to the sediment to lyse RBC and allow other elements to be observed more easily [7]. The addition of acetic acid, however, has a dilutional effect that makes it difficult to accurately estimate the number of elements that originally were present. Addition of dilute acetic acid can also be used to dissolve the large numbers of calcium carbonate crystals in the urine of horses that can obscure other elements in the sediment [48, 49]. Enumeration of elements in urinary sediment per HPF or LPF has traditionally been utilized in clinical practice and in the veterinary literature. Unfortunately, what constitutes an LPF or HPF has not been standardized. In this method, the number of observed elements is counted within the surface area (mm2) and depth (mm) of the wet mount (between the coverslip and the glass microscope slide) examined. Most elements under the coverslip fall into the same plane of focus within a short time. Consequently, the use of LPF and HPF enumeration is a crude estimate of elements per volume of urine examined. Historically using the most commonly available equipment at the time, it was assumed that an LPF was that area observed using a 10× ocular (eye piece) and a 10× objective (100‐fold magnification); an HPF was that area observed with the use of a 10× ocular and a 40× objective (400‐fold magnification). The higher the total magnification, the smaller the area (mm2) examined in that field. The traditional 100× objective is designed to be used with oil and the 10× ocular to achieve 1000‐fold magnification, but this is not part of a routine examination of urinary sediment. Oil immersion microscopy can be used at times to gain greater detail and magnification of wet mount preparations, but the mechanics of oil interacting with the coverslip over liquid and the resulting movement within the field of view (FOV) is challenging to achieve an optimal view as the slide position is changed. Dry mount cytology from urine sediment is recommended in these instances. Alternatively, the use of newer generation oil‐free 100× objectives (1000× total magnification) can be used to examine details of organisms or intracellular features; oil should not be used with these objectives. This facilitates shifting the objectives readily between higher and lower magnification fields without blurring from oil on the coverslip. Focusing collars on the 40×, 60×, and 100× objectives in some microscopes allows greater resolution and clarity to be achieved, as the operator coordinates the fine focus with one hand and rotating the focusing collar on the objective with the other hand. Numerous advances in the manufacturing of microscopes now allow access to microscopes with several different magnifications for the ocular (7×, 10×, and 15×) and for the objectives (4×, 10×, 25×, 40×, 45×, 50×, 65×, and 100×). The total magnification (ocular × objective) is loosely associated with the area (mm2) examined, but field area can vary by 33–200% depending on the field number (FN) of the ocular, even with the same objective magnification [3,50–53]. The diameter of the FOV is calculated by the formula: FN ocular/objective magnification (e.g. 20 mm FN/40× objective = 0.5 mm) [54]. If the same objective is used, then the limiting factor of the size of the area viewed is the diameter (mm) of the opening in the field diaphragm of the ocular. Early microscopes had an ocular FN of 18 mm, but modern scopes now use oculars with FN up to 28 mm to widen the field viewed. If a tube lens was present between the ocular and the objective, that would need to be considered to determine the final FOV; however, most modern day scopes do not have a tube lens and have infinity objective lens such that the tube length is constant as well [50, 51]. The FN is often engraved on the side of the ocular indicating how many millimeters of diameter are under view (approximately 18–26.5 mm). If a number is engraved on the side of the objective, it is the numerical aperture, which improves resolution but is not needed to calculate the FOV. The higher the FN of the oculus, the greater the diameter (mm) that will be examined and, thus, more opportunity to observe and report an element per “field.” Small changes in the diameter (d) of the field lead to relatively large changes in area (mm2) according to the formula Area = π(d/2)2 [55]. Precise denotation of the field size examined is pivotally important during mitotic counts and evaluation of suspected neoplastic tissue and in histomorphometry for the enumeration of osteoclasts or osteoblasts per unit examined during the evaluation of bone [50, 56]. Such precise definition of the area of the field to be examined from a wet mount is not likely to be of such great importance, since the number of elements from urine sediment is often near zero or there are many elements. It is unlikely that there will be much variation between the FOV encountered with microscopes commonly used in veterinary private practices when the same magnification objective is used. When the number of elements is near the upper cutoff for the reference range, elements could be reported to be increased or in the reference range when LPF or HPF areas are examined by different microscopes. In such instances, it is advisable to examine more than the usual 10 microscopic fields in order to increase the accurate classification as increased or not by LPF or HPF methods. Since reporting the average number of elements per LPF and HPF is potentially misleading, it has been suggested to instead report the number of elements by total magnification of 100× for LPF and 400× for HPF [56]. For precise work, the number of elements reported per area (mm2) or diameter of field (mm) avoids confusion as to the field evaluated. Periodic calibration of your microscope system by measuring the FOV diameter (mm) with a stage micrometer that is within 0.02 μm is recommended (Meuten personal communication 2020). A paradigm shift from the well‐entrenched reporting as per LPF and per HPF for urine sediment in veterinary medicine to the more accurate method of per μl of well‐mixed urine before sedimentation is advocated. This change would then align with how elements are generally enumerated quantitatively in other veterinary body fluids as in a CBC or cytological evaluation of body fluids [27]. The decislide method to examine and quantify urine sediment is preferred by some veterinary clinical pathologists for generation of the most accurate results for enumeration (Dr. Mary Nabity and Dr. Jessica Hokamp, personal communication, 2020). The decislide contains 10 individual acrylic chambers that standardize the volume per area examined and prevents cross contamination between the multiple samples. This method allows greater certainty that the volume of urine examined will be known and the same each time urine microscopy is performed. In one method, a standardized volume of well‐mixed whole urine is added to the chamber and allowed to sediment to the bottom by gravity and then examined. In another method more commonly used in veterinary medicine when precise enumeration of elements is desired, the urine is centrifuged first, the supernatant is resuspended in 20% of the original volume of the processed urine, and then 25 μL is added to the chamber (Dr. Jessica Hokamp, personal communication). More cells are usually identified with the use of this system compared to the microscopy of glass slides with coverslips (Mary Nabity, personal communication). Considerable variability in the volume of urine examined can occur with the use of glass slides and coverslips associated with the use of small or large coverslips, as the same volume applied will spread across the coverslip. Additional variables that effect the enumeration of elements include the volume of sediment applied to the glass slide and the dilutional effect of urine added to the sediment for resuspension, if any. Resuspension of sediment with supernatant is sometimes accomplished by using a volume of urine using 10–20% of the total urine volume that was processed. In some laboratories, the volume of urine is preset at 1 mL, but this is not optimal (Jessica Hokamp, personal communication). Another recommendation has been to add 0.5 mL of supernatant to resuspend the sediment regardless of the original urine volume processed [27]. Due to all of these variables, converting the numbers of elements reported per HPF or per LPF to per μL is problematic, as there can be substantial variation in the volume of urine under the coverslip that will vary by how the slides are prepared. Urine microscopy results reported as per HPF or per LPF are inherently semiquantitative and can lead to false‐negative or false‐positive bins that result in both overdiagnosis and underdiagnosis of upper and lower urinary tract diseases compared to the per μL method of reporting [27]. Increased WBC in urine sediment reported at >40/μL using volumetric chamber counting compared well to the finding of >5 WBC/HPF in one report. In the same report, much greater variability for the enumeration of WBC per HPF was noted compared to chamber counts, an effect that was attributed to differences in the volume of supernatant used to resuspend sediment and the size of the drop applied to the microscope slide [57]. Dramatic differences in the number of elements reported per HPF from the same urine sample were illustrated in one white paper depending on the volume of urine processed and the volume of supernatant used for resuspension of sediment [58]. The initial urine volume that was processed in one example was either 5, 2.5, or 1 mL, and then, all sediments were resuspended in 500 μL of supernatant. Elements were reported at 12, 6, and 2–3 per HPF, respectively, with the lowest numbers reported due to greater dilution in the analysis of the smallest urine volume analyzed with a preset amount of supernatant used for resuspension. In a second example, 2.5 mL of urine was analyzed using 500‐μL supernatant to resuspend the sediment generating a report of six cells per HPF. When 5 mL from the same sample were analyzed but resuspended in 250 μL of supernatant, 24 cells per HPF were reported. Results from these two examples could be considered normal or abnormal, depending on the methods of preparation for microscopy. It is not always possible to accurately identify all the elements in a urine sediment sample because the morphology of cellular elements can be altered as a consequence of the relatively wide pH and osmolality range of normal and abnormal urine, as well as the storage conditions employed before microscopy. Inexperienced laboratory technicians may have more difficulty identifying elements in the sediment, but experienced laboratory personnel and veterinary nephrologists can also encounter difficulty at times. When the definitive identification of elements in the urine sediment is in doubt, consultation with more experienced personnel is warranted. Urine sediment examination by an experienced nephrologist often yields clinically important information that is not reported by routine hospital laboratory personnel in human medicine [9, 39, 59, 60]. A nephrologist is more likely to recognize the presence of RTE, granular casts, renal tubular epithelial cellular casts, and dysmorphic RBC in urine than are certified medical technologists in human medicine [9]. Cells may enter the urine either by desquamation of epithelial cells along the course of the genitourinary tract or by entry from the circulation through the urinary tissues into urine (WBC and RBC). Cells that enter the urine are exposed to a potentially hostile environment of variable osmolality and pH that also may contain enzymes, toxins, and sometimes bacteria. Changes in cell size, morphology, and staining characteristics may occur when cells undergo permeability changes and degenerate [61]. Clinical experience and science must often be integrated to accurately identify elements observed in the urine sediment [39]. Adding a drop of formalin to urine sediment (unstained or stained) can preserve elements until another observer can offer an opinion. A digital picture of a confusing or interesting urine sediment finding taken through the microscope eyepiece using a smart phone or other digital camera can provide excellent images that can be sent to specialists for further assessment. Digital images of abnormal urinary sediment can also be included as part of the patient’s medical record. The camera is placed approximately 0.5–1.0 cm above the microscope eyepiece, and automated focus and exposure are used to capture the image [62]. The use of smart phone or camera adapters that mount the digital device to the microscope eyepiece can make this process easier, because the stable attachment no longer requires fine eye–hand motor coordination. Several brands of adapters are made for this purpose and can be found during a routine web search. Digital cameras for permanent attachment to the microscope as well as digital microscopes themselves are becoming more affordable and, therefore, more common in the average veterinary practice. Multiple digital images of urinary sediment are acquired by automated sediment analyzers that allow the sharing of images for further review. Dry mount slides can be prepared by spreading a drop of urine sediment on a microscope slide and allowing the film to air dry, a technique similar to that used for preparing samples for cytologic evaluation. The prepared slide can then be stained using Diff‐Quik® on site or submitted to a reference laboratory for Wright‐Giemsa (Romanowsky) staining to allow a more detailed evaluation of cells and bacteria. Casts and crystals usually are not preserved by this technique [61], but crystals may be faintly visible. Finding intracellular bacteria indicates that the bacteria are not merely contaminants. Slides prepared for cytology should not be heat fixed, frozen, or exposed to temperature extremes, condensation, or formalin fumes [63]. Dry mount films can also be made from sediment that previously has been stained using a supravital stain. Such a preparation can be examined as is or can undergo additional staining with Wright‐Giemsa (method of Dr. Gary Kociba). One veterinary author recommends submission of urine sediment in a small Eppendorf tube® that is tightly sealed, rather than whole urine, in addition to films on glass slides [63]. Semipermanent wet mounts of urinary sediment provide another method to keep urine sediment elements available for further on‐site evaluation or for training of laboratory personnel. Coverslipped glass slides of urine sediment can be kept for several hours in a covered Petri dish humidified by moist filter paper on the bottom [64]; the glass slide is separated from the bottom of the Petri dish by wooden applicator sticks while being refrigerated [11]. Permanent wet mounts of urine sediment may be made on glass slides if a portion of the sediment is mixed with gelatin, glycerin, and phenol [65] or with formaldehyde and gelatin [66]. The sediment can be placed on the microscope slide first, but placement on the cover slip is preferred because doing so minimizes the entry of air bubbles and is more likely to establish an airtight seal when lowered onto the microscope slide. The coverslip is then sealed with Vaseline, balsam, or methacrylate polymer (Shandon‐Mount®; Shandon, PA, USA). Refrigeration of these wet mounts also may enhance long‐term preservation of the sample. Excellent cellular detail and integrity of casts were maintained for over six months when a drop of formaldehyde was added to the urine sediment after centrifugation. The preparation was then allowed to sit overnight at room temperature to kill microorganisms and preserve elements in the sediment. 2–3 mL of 15% liquid gelatin is then applied, and a few drops of supravital stain are added to this mixture (optional). A drop of this sediment mixture is placed on a coverslip, applied to a glass slide, and sealed [65]. This method was adapted from a similar one designed to preserve urine sediment stored in tubes for future teaching of students and technicians [64]. With the tube technique, formalin is added to the sediment in a small tube and supravital stain is added (optional). Adding serum to the sediment is also an option, as described in the original report. The tube is capped or sealed and stored in the refrigerator. We have used this tube method to store interesting urine sediments for teaching purposes for up to one year. Automated microscopy has been used increasingly in human medicine to improve laboratory efficiency as well as accuracy and reproducibility in identifying and enumerating elements in urine sediment [18] and is now considered economically feasible for routine use. In human medicine, these methods are reproducible, faster, and more accurate than results provided by manual microscopy [67, 68]. Use of automated in‐house microscopy is likely to provide more standardization than is possible using manual microscopy in veterinary practices. Automated microscopy in veterinary medicine offers a method to standardize how urine is processed (e.g. volume analyzed, speed of centrifugation), how the sediment is examined, and how the elements are counted. Increased accuracy for identification of elements in sediment can be provided in veterinary practices where highly trained and experienced laboratory personnel are not available. An additional advantage of automated microscopy in veterinary medicine, where the amount of urine available a dog or cat is often small, is that a very small volume of urine is required for analysis. An automated microscopy system for veterinary use (IDEXX SediVue®) has been adapted from a human medicine using veterinary‐specific algorithms to recognize elements in urine from dogs and cats (Figures 6.10 and 6.11) [69]. A urine sediment examination report is generated in approximately three minutes after urine is added to the cartridge and the analysis started. This system has been used in private veterinary practices since 2015. The algorithms employed by a convolutional neural network in this analyzer have continued to evolve to allow a more accurate identification of elements in urine sediment. In this proprietary system, an automatically loaded cartridge is filled with 165 μL of urine using a pipette to aspirate from the bottom of the sample tube immediately after thorough mixing. SediVue® captures 70 digital images that correspond to about 45 HPF. Each digital image is nearly two‐third of an HPF at 400× total magnification. Approximately 10 μL of the 165 μL inserted into the cartridge is analyzed [31]. FIGURE 6.10 Front view, closed door of IDEXX SediVue Dx® Urine Sediment Analyzer. Source: Courtesy of Dr. Ronald Lyman, Ft. Pierce, FL. FIGURE 6.11 Open‐door SediVue®. Overview of urine cartridge handling system, light source, microscope lens, and centrifuge. Source: Courtesy of IDEXX Laboratories. Copyright © 2022, IDEXX Laboratories, Inc. All rights reserved. Used with permission. FIGURE 6.12 It is essential that urine be well mixed just prior to placing the urine sample into the urine cartridge. Picture is showing the urine tube being gently inverted and back to its original position a few times before the urine sample is aspirated. Source: Courtesy of Dr. Ronald Lyman, Ft. Pierce, FL. It is crucial to mix the urine sample immediately before pipetting it into the cartridge, [70] because settling may occur within 15 seconds after mixing (Figure 6.12) [23]. If the urine sample has not been well mixed, aspiration from the top of the tube will underestimate the number of elements, whereas the overestimation of cells will occur if the sample is aspirated from the bottom of the tube. This factor is important during both manual and automated microscopy. Alternatively, urine can be added to the cartridge using a specialized syringe adapter. Overfilling of the cuvette results in an analysis error, and another cartridge must be properly filled in order to start the analyzer. The filled cartridge is delivered to an onboard gentle centrifugation system using a perpendicular arm at 260 RCF for 30 seconds (Figure 6.13). This centrifugation is well below the maximal 400 RCF that is recommended, providing a technique that provides less acceleration and force that otherwise could contribute to breakage of sensitive elements. No pellet of urine sediment is achieved using this method because the sediment rapidly settles to the floor of a 1‐mm‐deep horizontal chamber in the cartridge. Also, no coverslip margin is present to examine for preferential delivery of sediment elements. In most cases, lipids accumulate above this level and are out of the field of microscopy, eliminating confusion between RBC and lipid droplets. Light is directed through the top of the cartridge to the inverted microscope below, and 70 high‐resolution, grayscale images are captured for analysis. Urine sediment samples that are highly cellular result in an error notice so that the element numbers per field are not reported until the sample has been diluted and the analysis rerun. This technology uses face recognition algorithms to accurately identify and enumerate elements in urinary sediment (Figure 6.14). Each image captured represents two‐thirds of a typical HPF (400×) examined during standard manual microscopy; a total of 46 HPFs are analyzed. The number of elements per HPF is averaged from evaluation of all 70 images. This instrument measures the number of elements per μL and converts this number to elements per HPF (400×) or per LPF (100×) and provides options to report elements per μL, per HPF or LPF, or both. A report is generated that details the numbers per HPF of RBC, WBC, squamous epithelial cells, nonsquamous epithelial cells, and crystals (struvite, calcium oxalate dihydrate, or nonclassified crystals) identified during the analysis. Casts are reported per LPF as hyaline and nonhyaline. Additionally, bacteria are reported as rods or cocci and as none seen or rare, as well as suspected presence or present. Laboratory technicians and the supervising veterinarian still must have the necessary skills to identify specific types of urine sediment elements that are not completely characterized by this automated system. The 70 high‐resolution digital images are captured and available for manual review for a limited time, after which only the best six images selected by the system will be archived [69, 71]. Each image is scored relative to the clinical relevance of the formed elements present. For example, an image with a nonhyaline cast that is present in only a few of the 70 images will have a higher score than an image with RBC or WBC, which should be present in most of the images. The best three images based on clinically relevant score are incorporated into the final report for review and permanently stored on IDEXX servers. All 70 images are initially available for review or for distribution to other individuals for review; an additional three images can be selected and added to the permanent patient record. To maintain quality control, it is recommended that a laboratory technician routinely checks at least the three selected images to further ensure the accuracy of what the analyzer has reported. Further clarification of reported items can include clumps of WBC if they are seen on the images, the specific type of nonhyaline cast (i.e. granular, cellular, waxy), the type of nonsquamous epithelium (transitional or RTE) if these cells are found in clumps, and a specification of “other” for nonspecified crystal types. FIGURE 6.13 Long arm of low force centrifuge in back. Note chamber that accepts the urine cartridge to be spun and then delivered to the stage for sediment viewing. Source: Courtesy of Dr. Ronald Lyman, Ft. Pierce, FL. FIGURE 6.14 Screen shot capture showing representative identification and labeling of elements in urine sediment identified by SediVue® (IDEXX). The labels can be turned off and on. For quality control, it is a good practice to examine several fields to see how well the face recognition technology matches up the labeled element with what you see in the captured image. Not all elements are labeled. RBC = red blood cells; WBC = white blood cells; nsEpi = nonsquamous epithelial cell. Source: Courtesy of Dr. Ronald Lyman, Ft. Pierce, FL. In some instances, automated microscopy outperforms manual microscopy, possibly due to the higher number of microscopic fields evaluated and method of centrifugation (less time and lower g forces). In other instances, automated microscopy is outperformed by manual microscopy possibly related to larger volumes of urine that are evaluated and failure of algorithms to accurately identify urinary elements. Initial studies of the SediVue® for RBC and WBC using Kova® commercial controls displayed excellent precision [5]. Kova controls are well established for microscopic evaluation of RBC and WBC, and they have excellent stability for more than 12 months. Using the original software, automated microscopy of dog and cat urine had moderate sensitivity and high specificity for detecting >5 WBC or RBC/HPF when compared to manual microscopy, accurately identifying clinically relevant increases in these elements [4]. Positive and negative agreement between manual and automated methods was slightly higher for WBC than RBC in another study. Occasional false‐positive and false‐negative results for RBC and WBC were reported during automated microscopy in this study, but many of these occurred as a result of differences of only a few cells on either side of the cutoff threshold [72]. In another study by the same group, results from SediVue® were compared to those of manual microscopy as the gold standard for determination of elements in urine from dogs and cats. Positive results were defined when ≥6 cells per HPF were identified as WBC or RBC. Moderate‐to‐high sensitivity and specificity for the detection of urine RBC and WBC numbers were possible using this automated method of urine sediment microscopy (dog and cat urine combined) [73]. Updated software for this analyzer resulted in an increase in sensitivity for the detection of RBC and WBC with a slight decrease in specificity [74]. Using the current software, sensitivity and specificity for detection of RBC in dog urine or cat urine is good to excellent. For detection of WBC, the sensitivity and specificity in dog urine is good, but the apparent sensitivity in cat urine was less. The specificity of WBC in cat urine is excellent [74]. Automated microscopy by SediVue® categorizes crystals at ≥1 crystal/HPF into struvite, calcium oxalate dihydrate, or unclassified. Positive and negative agreement between manual and automated methods was very high for crystal identification in this study. False‐negative and false‐positive classification of crystals was uncommon. Struvite and calcium oxalate dihydrate crystals occasionally were categorized as unclassified [75]. In another study by the same group, urine sediment samples were considered positive for struvite or for calcium oxalate dihydrate crystalluria when ≥1 per HPF of either crystal was detected. Use of the updated software greatly improved the sensitivity (>97%) for recognition of struvite, but a mild decrease in specificity (81%) was observed. Sensitivity for detection of calcium oxalate dihydrate was close to 80% and specificity was >98% using either the old or new software [76]. Another study that examined urine from dogs (80%) and cats (20%) defined positive as >1 crystal per HPF for either struvite or calcium oxalate dihydrate by either method. Using manual microscopy, reported crystals were divided into semiquantitative categories: none‐rare, 1–5/HPF, 6–20/HPF, 21–50/HPF, and >50/HPF. Using the updated software, automated microscopy had a sensitivity of 91% and a specificity of 84% for detection of struvite. Sensitivity for detection of calcium oxalate dihydrate was 75% and specificity was 99% using the updated software compared to manual microscopy [74, 77]. Clinical interpretation of epithelial cells was similar between automated SediVue® and manual methods of urine sediment examination in one study [78]. In another study, ≥1 squamous epithelial cell per HPF or ≥1 nonsquamous epithelial cell per HPF was defined as positive by either manual or automated microscopy. Manual microscopy detected squamous epithelial cells in 5% of the samples and nonsquamous epithelial cells in 11% of the samples [79]. Using updated software, automated microscopy had a sensitivity of 33.3% for the detection of squamous epithelial cells and 99.4% specificity [74]. False negatives for detection of squamous epithelial cells by the automated method seemed to occur when the number was just above the minimal definition of ≥1.0 per HPF. Sensitivity was 71% and specificity was 87% for the detection of nonsquamous epithelial cells using automated microscopy. Categorization of nonsquamous epithelial cells requires the technician to review the digital images to further classify these cells. Bias for the identification of RBC and WBC in urine was compared between manual and automated microscopy in another study. High bias was identified with the automated analyzer at 21.2% for RBC and 15.1% for WBC. Bias was considerably reduced following a manual review of the digital images acquired by the automated analyzer to 2.5% for RBC and 3.1% for WBC [80]. The detection of casts during manual microscopy was compared to detection by automated microscopy using SediVue® in urine from dogs at a veterinary teaching hospital. Casts were identified by manual microscopy as the gold standard comparator in 112 of 455 samples (24.6% prevalence). The automated sediment reader had a sensitivity of 72% and a specificity of 94% in this study. A review of digital images allowed for a correction in the number of false‐positive and false‐negative results reported by automated microscopy. It is anticipated that improvements in the neural net used in the algorithm for cast identification will improve over time [81]. A review of digital images is needed to confirm the accuracy for the reporting of hyaline and nonhyaline casts, serving as a quality control for results generated by automated urine microscopy. Additionally, further categorization of casts as granular, waxy, and or cellular is needed when nonhyaline casts are reported by automated methods. Results from one automated urinary sediment analyzer (SediVue®) were compared to manual microscopy on the same sample as the gold standard for the identification of casts in urine from dogs. The identification of any cast (>0/LPF) by either method was considered positive. This automated method showed poor‐to‐moderate sensitivity (53.7%; 43.85–63.35%) in the detection of casts, as there were many false negatives [31]. Failure to identify casts might be attributed to low cast numbers, small sample volume, and an inability of the automated method to focus through different planes of urinary sediment. Moderate‐to‐high specificity (86.0%; 81.78–89.51%) was demonstrated by the automated method, indicating few false positives. Mucous strands, squamous epithelial cells, debris, and environmental contamination were occasionally identified as casts. Excellent specificity (>99%) for the analyzer compared to the manual method was found when the threshold for casts was modified to ≥1/LPF instead of >0/LPF, but the sensitivity did not improve. The automated analyzer generated 47 false‐positive and 50 false‐negative results prior to digital image review. Interestingly, a review of digital images collected by the analyzer identified casts in 36.2% (17 of 47) of cases originally classified as “false positive” that were not detected during manual microscopy (6 hyaline and 14 nonhyaline casts), so these were reclassified as true positives. In these instances, the analyzer performed better than the human operator, challenging the concept of manual microscopy as the gold standard reference for all urinary elements. This study concluded that there was moderate agreement between the automated and manual methods for the identification of casts [31]. Whenever the analyzer reports casts, a visual review of the captured digital images should be undertaken to confirm their presence and type. Additionally, the digital images should be reviewed in cases with suspected AKI, in which casts were not reported. Manual urine microscopy is also an alternative to confirm the presence or absence of casts in these instances. Another automated urinary sediment analyzer became available for use in veterinary medicine in 2018 (Zoetis VETSCAN® SA Sediment Analyzer) (Figure 6.15). This analyzer uses similar technology to identify elements in urine sediment as described above for SediVue® but does not use centrifugation before the enumeration of the elements. A probe outside the analyzer aspirates 650 μL of urine into a counting chamber. Urine then sits in this chamber for three minutes to allow sediment to fall by gravity before elements are counted. Elements are identified and then counted as the average occurring per μL from 192 fields and 96 high‐resolution digital images. Ninety‐six images are pulled forward and are available for review at the time of analysis if desired and are stored in the cloud for later review if needed. The urine sediment report displays six images with the most rare or important elements (such as casts) displayed first. The displayed images are approximately the size of what would be viewed during HPF manual microscopy (Figure 6.16). Urine sediment results are reported as the number per μl instead of per HPF or LPF. Reporting the number of elements per μL is considered a more accurate method, but this has not yet become widely accepted in private general practice. Reference ranges using per μL have been developed for use with this analyzer. Comparisons of the urinary sediment results from this analyzer to that of manual microscopy or to SediVue® automated microscopy have yet to be published. FIGURE 6.15 Front view of Zoetis VETSCAN® SA Sediment Analyzer. Automated urine sediment analyzer aspirates urine from probe on the outside of the analyzer (below shelf on right). Source: Courtesy of Zoetis. FIGURE 6.16 WBC and RBC. Note that RBC are about one‐third to one‐half the size of the WBC. WBC are identified by their nucleus, most of which appear polymorphonuclear. Some bacteria that are out of focus are in the background. Image acquired by VETSCAN® SA Sediment Analyzer. Source: Reproduced with permission of Zoetis. In general, automated microscopy agrees well with manual microscopy for the identification of RBC and WBC in human [82–84] and veterinary medicine (dogs and cats). Automated urine sediment microscopy appears to be well suited for screening healthy individuals with minimally active urine sediment and less accurate for those with more unusual pathology [83, 84]. Crystals and squamous epithelial cells are identified with moderate accuracy, but the accurate identification of nonsquamous epithelial cells, bacteria, and casts was poor at times [83]. Enumeration of RBC, WBC, and epithelial cells was very similar when comparing results from manual to automated urine microscopy in one report from human medicine. In contrast, there was no concordance in these methods for the identification and reporting of casts. Manual microscopic examination by trained personnel was recommended to improve the accuracy of reporting for dysmorphic RBC, bacteria, yeast, crystals, and casts. Automated urine microscopy was still advocated to save time and to provide the standardization of methods [84]. Review of the digital images of urine sediment harvested by the automated analyzer may be of high enough quality for laboratory personnel to assess in lieu of manual microscopy in these instances. The accuracy of automated methods of urine sediment evaluation has continued to improve, but still needs further improvement [18]. Lack of agreement between manual and automated methods for identification of casts was particularly problematic in one study of humans [84]. A review of results flagged during automated microscopy requires a review of the digitally captured pictures or manual microscopy of the urine sediment to assign definitive identification for the final report [83, 84]. The quality of the digital images is usually sufficient to identify questionable elements without the need to perform manual microscopy. For elements still in question, digital images can be sent to consultants for further review and rendering of an opinion. Recent advances in recognition software can allow for the identification of malignant or atypical epithelium by some analyzers in human medicine. One of the weakest points in automated microscopy remains accurate recognition for small cocci. Some analyzers now include phase contrast in addition to routine bright‐field microscopy to improve the identification of some urinary elements [85]. The accurate detection of bacteriuria remains difficult using automated analyzers [86]. Of 191 feline urine samples analyzed for bacteriuria, 36% were determined to be free of bacteria by both an observer and an automated analyzer, and no bacterial growth was detected in these samples. Of the samples, 14% were determined to have bacteriuria, and 24 of these 27 samples were culture positive. In 50% of the samples, a clear identification was not possible; 85% of those that did not have a clear identification were culture negative. SediVue® reports bacteria as rods or cocci and enumerates them as none to rare, suspect present, or present [87]. Concordant negative findings were found in 36% (69 of 191) of samples from cats as no bacteria were reported by an automated sediment analyzer (SediVue®), none were observed following human review of digital images from the analyzer, and urine culture was negative; WBC count was low in most samples. Bacteria were identified by the analyzer and review of digital images along with positive urine culture (>10 colony‐forming units (cfu)/mL [3]) in 24 of 27 cases. In the review of digital images, bacteria were placed into the same category as cocci or rods by both the analyzer and a human observer. In the remaining 95 cases, there was discordance between the report of bacteria by the analyzer and the observer in 50% of the samples; 81 samples were culture negative. Disagreement was more frequent in categorization of bacteria as cocci (91 samples) compared to rods (41 samples). Cocci are more difficult to identify with certainty and were frequently noted as suspected to be present by the analyzer and the human observer. WBC counts were >5/HPF in 82% of those samples with positive urine culture results. Dry mount cytological evaluation of urine sediment or urine culture is recommended to confirm bacteriuria when there is discordance between bacteria identified by the analyzer and the human observer [87]. Automated urine sediment examination in veterinary medicine has the potential to increase the number of complete urinalyses performed in private practice, as has been observed in human medicine due to an environment of improved workflow and decreased turnaround time [18, 82, 84]. Increased performance of complete urinalyses in‐house provides both a means to increase the quality of diagnostic information that is generated and an appropriate source of income for the practice. Automated methods standardize the method of analysis (volume of urine, centrifugation force and time) while decreasing the time required to generate a report. Captured high‐resolution images still should be reviewed by the veterinarian and technician to assure the accuracy of results reported by the analyzer before providing final approval for entry into the medical record. Review of images can also provide an opportunity for ongoing technician training. In general, the review of digitally acquired urine sediment images can replace standard microscopy. However, manual microscopy using LPF and HPF magnification still remains the gold standard for evaluation of urine sediment despite increased availability and accuracy of automated urine sediment analyzers [18]. Small numbers of RBC, WBC, and epithelial cells are often identified during the urine sediment examination of healthy animals (i.e. inactive urine sediment), but casts (hyaline and granular) are rare in normal animals. The number of these elements (per HPF or per LPF) identified during urine sediment examination of sick animals (i.e. active sediment) is higher and associated with various upper and lower urinary tract disease processes. Generalized reference ranges for urinary sediment evaluation are not easily provided because of methodological differences among laboratories. These differences include the sample volume processed, time and force of centrifugation, amount of resuspended sediment used to make the coverslip preparation, and whether or not the sediment is stained. The average numbers of RBC, WBC, and epithelial cells are reported per HPF, whereas the numbers of casts are reported per LPF. The presence or absence of bacteria, crystals, amorphous material, lipid droplets and sperm (in males) are also noted. Positive findings should be further characterized as few, moderate, or many. Results from an average of 10 microscopic fields are reported. Adherence to a standardized method of urine sediment examination allowed the quantification of numbers of RBC and WBC per HPF that correlated well with numbers of WBC and RBC excreted per day as the gold standard in human medicine [88]. The WBC and RBC estimates on examination of wet mount preparations also agreed well with hemocytometer counts performed using urine from dogs and cats [89]. Detailed causes and the suggested diagnostic approaches for symptomatic and asymptomatic hematuria in human medicine have been reviewed [90–94]. Despite intense investigation, the cause of isolated microscopic hematuria frequently remains unidentified in human medicine [90]. Table 6.4 lists the causes for excessive numbers of intact RBC in urinary sediment in dogs and cats. Some of the differentials overlap with that for the causes of hemoglobinuria shown in Table 5.11. RBC measure approximately 5–7 μM in diameter and are smaller than WBC (Figure 6.17) [63]. RBC appear as nonnucleated pale yellow discs in unstained urine sediment preparations (Figure 6.18). If RBC have been present in urine for a relatively long time, they may appear colorless because hemoglobin is leached from the cells over time (Figure 6.19) [95]. RBC are variably stained by Sedi‐Stain® and may vary in appearance from light pink to dark red (Figure 6.20). Feline RBC often stain darker than do canine RBC (Figure 6.21). Staining can vary among RBC in a single urine sediment sample or among different urine sediment samples. RBC in highly concentrated urine are smaller than normal as a result of crenation [96] caused by water movement out of the RBC as a consequence of the osmotic gradient (Figure 6.22). Table 6.4 Causes for excessive number of intact RBC in urinary sediment. Some of the causes for increased RBC will also be associated with variably increased numbers of WBC in the urinary sediment. FIGURE 6.17 Urine sediment from a dog. Numerous RBC, all of which are light orange in color. The concave nature of these RBC is apparent based on the central darkened area. A few RBC are seen on‐end, further demonstrating this concavity. Three WBC in the central field are easy to identify due to the distinct lobulation of the neutrophil; these stain red and are slightly larger than the RBC next to them. There are numerous degenerating WBC that are considerably larger than the RBC, have a bluish stain, and have less recognizable nuclei. No obvious bacteria are identified. FIGURE 6.18 Note three types and sizes of round cells in this unstained urine sediment. The most numerous cell is the RBC; it is the smallest and has some inherent color without stain. Next most common are moderate numbers of neutrophils, which are defined by their being about 2–2.5 times the size of the RBC and having a lobulated nucleus. The largest of the round cells are transitional epithelial cells (nonsquamous epithelium). Sporadically throughout the field are needle‐like struvite (amorphous) crystals. FIGURE 6.19 Urine sediment from the cystocentesis syringe as shown in Figure 6.26. This sample was from a young Great Dane with botryoid rhabdomyosarcoma. Note a large number of pale staining RBC, many of which display a concave shape. There are moderate numbers of neutrophils. Most of the WBC display red staining to the nucleus, while same show a blue stain. No tumor cells or bacteria were identified. FIGURE 6.20 Urine was obtained by free catch from a middle aged intact male dog. Urine sediment at 104×. Too numerous to count (TNTC) RBC with rare WBC, and no bacteria identified. Hematuria was from bleeding associated with benign prostatic hyperplasia (BPH). Very dilute urine will result in swelling of RBC, [96], and some RBC may rupture, leaving “ghost” membranes behind if the cell membranes have not completely disintegrated. Ghost RBC are not readily detected by routine light microscopy, but can often be observed using phase microscopy. In a study of dogs, RBC survival in urine was correlated with osmotic stress. RBC survival markedly decreased when urine was highly concentrated and decreased to a lesser extent when urine was dilute [97]. Survival time of 51Cr‐labeled RBC was determined in water‐restricted dogs excreting concentrated urine and again in the same dogs after a six‐week period of ad libitum water consumption. During water restriction, RBC survival was decreased to 14% of normal. In rats with untreated diabetes insipidus, 51Cr‐RBC survival was decreased 30% as compared to the same rats treated with antidiuretic hormone. FIGURE 6.21 Numerous RBC in urine sediment from a cat with idiopathic cystitis (100×). Note light and dark staining RBC and the complete absence of WBC. There are some struvite crystals that are of questionable significance in this disease. It is important to know how the urine was handled as crystals often appear following storage that were not there when freshly collected. FIGURE 6.22 Struvite crystals. Many crenated RBC and rare WBC in the background. Unrefrigerated urine causes a relatively rapid lysis of erythrocytes and leukocytes [98]. Leukocytes persisted without significant decrease in numbers or altered morphology for at least 45 days in some refrigerated urine samples that were acidic of one study of human urine [98]. In contrast to WBC, the enumeration of RBC and squamous epithelial cells was better maintained in tubes at room temperature containing preservatives [99]. Alkaline urine also contributes to the lysis of RBC [98]. Crenated RBC are sometimes confused with WBC because the spicules on crenated RBC can look like granules [63]. Lipid droplets are occasionally confused with RBC (especially in cats), but lipid droplets vary markedly in size, are highly refractile, and are in a plane of focus just below the coverslip (Figure 6.23) [100]. If in doubt, a drop of 10% acetic acid can be added to the edge of the coverslip to lyse the RBC. Lipid droplets also take up Sudan stain (Figure 6.24). Precipitated hemoglobin (in patients with intravascular hemolysis and hemoglobinuria) may appear as distinctive orange–brown globules that initially resemble RBC (Figure 6.25). Careful examination will disclose wide variation in particle size, whereas RBC are of more uniform size. FIGURE 6.23 Stained urine sediment from a cat. RBC are all the same size and stain orange‐red. Note a few refractile droplets (lipid), one of which is about the same size as RBC. Lipiduria is normal in cats. Source: Reproduced by permission Dr. Richard Scott, Animal Medical Center, NY. FIGURE 6.24 Lipid droplets in urinary sediment from a cat. Sudan stain has resulted in strong uptake of red color into droplets. The amorphous material in the background is possibly stain precipitate. FIGURE 6.25 The report on this dog initially returned with TNTC RBC as the major finding. More careful inspection of this microscopic field reveals multiple size spherical structures that have their own peculiar color. These are the precipitates of hemoglobin that entered urine from this animal following a splenic torsion and hemolysis of systemic RBC. The finding of occasional RBC is considered normal in the urine sediment of healthy dogs and cats. The origin of these RBC in healthy individuals is not known. Healthy dogs and cats may have 0–8 RBC/HPF in voided urine, 0–5 RBC/HPF in urine obtained by catheterization, and 0–3 RBC/HPF in urine obtained by cystocentesis [70, 100, 101]. Hematuria is the common term used to describe increased numbers of RBC in urine [95]. Hematuria can be macroscopic (or “gross”) when easily identified by the naked eye (Figure 6.26) or may be microscopic (invisible to the naked eye). The number of RBC/HPF needed to impart red color to a urine sample has been described in dogs [102], as discussed in Chapter 4. One group of investigators considered even 1 RBC/HPF indicative of hematuria in the second midstream voided urine sample of the morning in people [95, 103]. The extent of trauma that occurs during urine collection must always be considered when evaluating possible causes for hematuria (see Chapter 3). Although 0–3 RBC/HPF originally was defined as the normal range for RBC in urine collected by cystocentesis from normal dogs and cats [100], 0–10 RBC/HPF is often considered normal, and up to 50 RBC/HPF [104] may be encountered in some urine samples as a result of the largely unavoidable trauma associated with this method of urine collection, regardless of operator expertise. An alternative consideration is that the trauma of cystocentesis more readily allows RBC to enter the sample when the bladder is diseased. FIGURE 6.26 Dark red urine from a young Great Dane with botryoid rhabdomyosarcoma that presented for signs related to bone pain. It is often advised to evaluate first morning urine samples for the presence of RBC because RBC are better preserved in more acidic and relatively concentrated urine. However, RBC that have remained in the bladder overnight can undergo some lysis as a result of prolonged contact time in urine [1]. Lysis of RBC in the sample should be considered when the occult blood chemistry dipstrip reaction is highly positive for heme pigment despite a paucity of RBC observed microscopically. The finding of hematuria in itself does not identify where in the urogenital tract the excessive entry of RBC has occurred. The kidney is incriminated when free RBC are observed simultaneously with casts that also contain RBC (see section on casts below). In human medicine, free RBC in the presence of granular casts increases the likelihood of loss of RBC through the glomeruli [95]. Renal parenchymal bleeding can result in the presence of erythrophagocytes, which develop when renal tubular cells phagocytize RBC that pass through the nephron. Bleeding from the renal pelvis, ureter, bladder, or urethra was considered more likely when RBC were observed along with larger epithelial cells arising from deeper layers of the uroepithelium [95]. In human medicine, hematuria of renal origin is considered more likely when renal proteinuria, cylindruria, renal tubular epitheliuria, and dysmorphic RBC are also identified [105]. Hematuria has many potential causes (Table 6.4). RBC in urine may arise from the kidney, ureter, bladder, urethra, prostate gland, uterus, vestibule, vagina, vulva, penis, prepuce, or perineum, in part depending on how the urine sample was collected. The causes of hematuria can be grouped in categories such as renal parenchymal disease, renal vascular disease, lower urinary tract disorders (including trauma), and systemic coagulation disorders [88]. Findings from the history, physical examination, and method of urine collection must all be analyzed and integrated in order to properly localize the origin of hematuria. Hematuria is most often identified after trauma (e.g. cystocentesis, urolithiasis, and injury), bacterial infection, and neoplasia in veterinary medicine. Hematuria in lower urinary tract disorders occurs as a consequence of small‐to‐large vessel damage and subsequent bleeding. RBC in the urine sediment that arise from the lower urinary tract have been called “isomorphic” (i.e. a homogeneous population of normochromic oval and biconcave cells) as opposed to “dysmorphic” RBC [106]. The presence of so‐called dysmorphic RBC in the urine sediment indicates renal origin in humans, likely following transglomerular passage. RBC in the urine sediment of human patients with glomerulonephritis sometimes have distorted morphology with characteristic membrane blebs and are referred to as dysmorphic RBC, whereas RBC in the urine sediment of patients with urologic disorders typically have normal morphology and are referred to as isomorphic RBC [90, 91, 95, 106]. It is important to not confuse crenated RBC with spicules that form in concentrated urine [91]. Isomorphic RBC in the urine sediment can have either normal (dark cells) or decreased (clear cells) hemoglobin content [95]. The occurrence of dysmorphic RBC has not yet been reported from urine microscopy of dogs or cats with glomerular disease. Close inspection of urine sediment from dogs with XLHN, however, routinely reveals the presence of RBC that have the characteristics described for human dysmorphic RBC. These dogs always have moderate‐to‐large blood on the dipstrip reagent pad in association with <5 RBC/HPF in urinary sediment from voided samples, suggesting that most RBC lyse as they pass through the diseased glomerulus (Mary Nabity, personal communication 2020). How dysmorphic RBC are formed is not known, but they could arise from some combination of physical trauma during passage through the glomeruli, exposure to osmotic stress in the distal nephron, and alteration after phagocytosis by RTE [105]. In rats with experimental glomerulonephritis and hematuria, RBC traversed the glomerular capillary between gaps in the discontinuous basement membranes as demonstrated by scanning and transmission electron microscopy [107]. In a similar study of rabbits with experimental glomerulonephritis with hematuria and RBC casts, RBC were found in the glomerular urinary space in 50% of the glomeruli evaluated by scanning EM as well as passage of RBC and neutrophils crossing the glomerulus through gaps in the basement membrane [108]. Wide variation in size (anisocytosis) and shape (poikilocytosis) characterized dysmorphic RBC that were recognized during bright‐field microscopy. Dysmorphic RBC are often hypochromic (ghost cells) with a large amount of central pallor. Spherocytes, target cells, schistocytes, acanthocytes, and crenated cells are also sometimes observed [90, 91, 105]. Dysmorphic RBC may be more readily recognized by phase microscopy [95]. RBC morphology should be normal on examination of a peripheral blood smear in a patient with dysmorphic RBC in the urine [105, 106]. Hematuria in humans was considered to be of glomerular origin when >40 or > 80% of the RBC were dysmorphic, and nonglomerular when the RBC were isomorphic [95, 103]. The proteinuria was considered mixed in origin when equal proportions of dysmorphic and isomorphic RBC were identified [95]. This classification system was not always accurate because isomorphic RBC can also be found in patients with glomerular disease [95], and dysmorphic RBC can be found in patients with nonglomerular (renal tubular) bleeding [106]. A less subjective indicator for the presence of glomerular bleeding is the finding of ≥5% acanthocytes during urine sediment examination. These acanthocytes are described as ring formations of RBC with ≥1 protrusions of variable shape and size. RBC that originate from glomerular bleeding (as compared to those originating from urologic disorders) are considerably smaller when measured by Coulter counter methods [95]. Glomerular‐origin RBC have a high predictive value for the presence of glomerular disease, even when they occur as an isolated finding. In humans, three urine specimens from the same patient, each collected at least one week apart, may need to be evaluated before the renal origin of hematuria can be identified [103]. It is not clear if glomerular hematuria is only a consequence of glomerular disease or if transglomerular passage of RBC contributes to the progression of chronic kidney disease (CKD) [90]. We recommend the evaluation of dry mount urine sediment preparations to further evaluate for the presence of dysmorphic RBC in veterinary patients with suspected renal origin hematuria. WBC in urine are spherical and usually 1.5–2.0 times as large as RBC (Figure 6.27). The diameter of a WBC in urine sediment usually is 10–14 μM, depending on the type of WBC (e.g. polymorphonuclear, mononuclear) and conditions in the urine (e.g. osmolality, pH, presence of bacterial toxins) [63]. Neutrophils are the most commonly reported type of WBC in urine sediment, but it is likely that lymphocytes and eosinophils are underreported because of difficulty in making definitive identification using wet mount microscopy. Neutrophils with distinct nuclear lobulation are easily identified. However, cellular degeneration with loss of nuclear detail frequently occurs and makes it difficult to distinguish neutrophils, lymphocytes, small renal tubular epithelial cells, and small transitional epithelial cells from one another. Fusion of the segmented nucleus of the neutrophil is an early degenerative change and is followed by nuclear fragmentation that contributes to the overall granular appearance of these cells. Other degenerative changes in WBC observed during urine microscopy can include the presence of foamy cytoplasm and nuclear dissolution, swelling, necrosis, and decreased stain uptake (karyolysis) [109]. Phagocytosis of bacteria by neutrophils also may contribute to the granular appearance of the cytoplasm (Figure 6.28), but such cells are readily lysed and frequently not detected. This distortion of morphology is most marked in the presence of bacterial infection. Neutrophils in the same urine sediment sample stained with Sedi‐Stain® can exhibit variable nuclear staining, ranging in color from pink to blue or dark purple (Figure 6.29). FIGURE 6.27 Four areas of clumped neutrophils with red staining nuclei. There are increased numbers of pale staining RBC, with small numbers of what appears to be punctate bacteria. In dilute urine, neutrophils may swell (Figure 6.30), stain poorly, have Brownian movement of cytoplasmic granules, and are often referred to as “glitter” cells [61]. Glitter cell forms of WBC were noted to frequently be associated with pyelonephritis, but specificity for this finding is low [37]. The glitter phenomenon may also be observed in patients with USG < 1.015. Leukocytes readily lyse in dilute (hypotonic) urine. Highly concentrated urine specimens may result in shrinkage of WBC with scant visibility of cytoplasm surrounding the nucleus, making definitive identification difficult (Figure 6.31) [35]. FIGURE 6.28 Stained urine sediment from a dog with a bacterial pyelonephritis. Renal involvement cannot be suspected based on the findings of this urine sediment alone. Note small clumps of WBC, which often indicate the presence of an organism causing the WBC to clump. Rod forms of bacteria are seen free and in clumps between WBC and free floating. A few of the WBC appear to have intracellular bacteria. Several of the WBC have swollen cytoplasm. A few RBC in the background allow the microscopist to make a comparison of size. FIGURE 6.29 Stained urine sediment from a cat with Corynebacterium UTI associated with urethral obstruction and a perineal urethrostomy. There is punctate “debris” in the background that could be bacteria or cellular detritus. The nuclei of some WBC stain deep red and others are light blue. The light blue staining WBC are slightly larger than the ones staining red. One WBC to the mid far right shows nuclear fragmentation. Many RBC are in this field, some which stain light red while others stain light orange and some do not take up stain. Encrusting cystitis associated with Corynebacterium was the diagnosis. FIGURE 6.30 Clump of neutrophils with blue staining nuclei and swollen cytoplasm. The cytoplasm can swell when the USG is less than 1.015 or from toxic effects on the neutrophil. One WBC with red staining has shrunken cytoplasm near top of image. Particulate matter that is not identified is seen at the top of this clump. FIGURE 6.31 Urine sediment showing numerous WBC with some in clumps. Notice the lack of cytoplasm around most of the neutrophils – this can happen in highly concentrated urine that has been in contact with the WBC for some time. Clumping of WBC often indicates an underlying inciting bacterial organism. There are many punctate particles in the background that could be bacteria. It would be best to convert the urine sediment to a dry mount cytology slide for further evaluation to more definitively determine if the bacteria are real or not. A few amorphous struvite crystals appear in the center of the field. Phase microscopy may be useful to more definitively identify the nature of the WBC nucleus. Adding a drop of dilute acetic acid to the edge of the coverslip may enhance the nuclear detail of neutrophils [96]. In some instances, staining may change the shape of WBC so that they are more easily recognized [45]. Peroxidase staining of urine sediment from humans turns neutrophils greenish‐blue and allows positive identification because peroxidase is abundant in mature neutrophils and absent in other cells [110]. Cytological evaluation of dry mount slides may also be helpful in the further evaluation of morphology, either using unstained sediment or processing previously stained wet mount sediment preparations. In a study of dogs and cats, differential WBC counts could not be performed because of poor preservation of WBC in air‐dried urine sediment preparations [89]. Small numbers of WBC may be found in urine from healthy dogs and cats, but their anatomical origin is not known. Neutrophils are the predominant type of WBC reported on urine sediment examination. Lymphocytes and monocytes are not easily differentiated from small epithelial cells and, thus, are likely underreported. The ratio of RBC to WBC (neutrophils) in normal urine is approximately 1:1, much different from the ratio in peripheral blood (approximately 500 : 1). Up to 8 WBC/HPF in voided urine, 5 WBC/HPF in urine obtained by catheterization, and 3 WBC/HPF in urine obtained by cystocentesis are considered normal. Up to 5 WBC/HPF is considered normal by several veterinary laboratories. WBC are not added to urine as contaminants during trauma associated with urine collection, as are RBC. In a study of urine from apparently healthy dogs, enumeration of WBC in urinary sediment measured in fresh urine at time 0 was better maintained in urine samples stored in plain glass tubes in the refrigerator for up to 72 hours compared to urine samples stored at room temperature in tubes with preservatives [99]. Leukocyturia and pyuria are the terms used to describe pathological increases in the number of WBC observed in the urine sediment. Table 6.5 lists the possible causes for detection of pyuria in dogs and cats. Abnormal numbers of WBC in urine sediment indicate urinary tract inflammation or inflammation of (or contamination by) the genital tract (i.e. vulva, vestibule, vagina, uterus, prostate gland, testes, epididymis, vas deferens, penis, prepuce, or perineum). The anatomical origin of excessive numbers of WBC (i.e. kidney, ureter, bladder, urethra, extra‐urinary) cannot conclusively be determined unless WBC are found in casts, which incriminates the kidney. Although not pathognomonic for bacterial infection, urine samples with the most severe pyuria typically are obtained from animals that have bacterial urinary tract infection (UTI). Sterile pyuria may accompany other urinary disorders, including urolithiasis and neoplasia. Because pyuria usually accompanies bacterial infection in patients with clinical signs, a finding of excessive numbers of WBC in the urine sediment should prompt a search for a possible infectious agent. Clumps of WBC often occur with bacterial infection although bacteria may not be readily visible (Figure 6.32). Careful examination of the spaces between clumped WBC often discloses bacterial organisms. Bacteria can often be identified adhering to the surface of WBC whether these cells are clumped or solitary. Bacteria may also be found within the cytoplasm of neutrophils after phagocytosis or may be seen free in the urine sediment (Figures 6.33 and 6.34) WBC can also clump in an alkaline environment [98], and alkaline urine frequently occurs in conjunction with UTI caused by urease‐producing bacteria. Table 6.5 Causes of pyuria. FIGURE 6.32 Image captured through the microscope eye piece using a hand‐held iPhone. The smallest poorly staining cells are RBC. Both blue and red staining nuclei are seen in the numerous neutrophils (larger than the RBC). Note that some large clusters of WBC are seen, often indicating the presence of an infectious organism. Occasional bacteria are near the WBC or are free‐floating. Occasional free nonsquamous epithelial cells are observed and one clump of the same type of epithelial cells can be seen at the bottom of the field. One epithelial cell is seen with a prominent tail, near the lower middle of the field. Epithelial cells are considerably larger than WBC. Eosinophils seemingly occur rarely in urine, but have the potential to be identified in patients with some types of AKI, including acute interstitial nephritis [111]. Eosinophiluria and eosinophilia were described in one dog with azotemic AKI with an underlying diagnosis of acute tubulointerstitial nephritis, likely secondary to an immune‐mediated reaction to ormetoprim‐sulfa. Urine sediment contained >500 WBC per HPF, >50 RBC/HPF, and 15–20 epithelial cells/HPF. The nature of the WBC and epithelial cells were not further characterized during urine microscopy. Cytology of urine sediment revealed that 60% of the WBC were eosinophils and the remainder were neutrophils. The occurrence of eosinophiluria appeared to be uncommon when evaluated by urine cytology in other veterinary urinary disorders associated with pyuria. The authors of this report recommended the increased use of urine cytology in cases with AKI and pyuria in order to further characterize the WBC beyond neutrophils [112]. It is likely that the occurrence of eosinophiluria is under‐reported when AKI patients with pyuria do not undergo cytological evaluation, as it is difficult to definitively identify WBC other than as neutrophils during wet mount urine microscopy. FIGURE 6.33 Stained urine sediment. Notice sheets of WBC clumping together. Most WBC are light blue staining and somewhat larger than those that are red staining. RBC to the left in this field stain light orange. Rare epithelial cells are seen. FIGURE 6.34 Many WBC (neutrophils) with easy to recognize polymorphonuclear nuclei. There is abundant cytoplasm in these neutrophils and the nuclei are well preserved. Bacterial rods are in the background. Image acquired by VETSCAN® SA Sediment Analyzer. Source: Reproduced with permission of Zoetis Bacteria should not be seen in the urine sediment of normal dogs and cats when samples are collected by cystocentesis. Normal bladder urine is sterile (based on conventional bacterial culture techniques), but the distal urethra, genital tract, and skin harbor bacteria that can enter urine when samples are collected by voiding or catheterization. Urine samples collected from the tabletop, cage floor, cage pads, hospital floors, litter box, or containers of urine submitted by owners often have bacteria that are identified in the urine sediment. If allowed to incubate at room temperature, these contaminant bacteria may proliferate, doubling in number after 45 minutes [100, 113, 114], which allows the observation of bacteria in the absence of pyuria (Figure 6.35). Contamination with normal bacterial flora from the urethra in voided or catheterized specimens usually does not result in large enough numbers of bacteria to be visualized microscopically in urine sediment that has been refrigerated. Most often, pyuria accompanies bacteriuria in animals with UTI, but pyuria can be absent in immunosuppressed patients (e.g. exogenous or endogenous corticosteroids, diabetes mellitus, cancer, and chemotherapy) and CKD (Figure 6.36). The absence of visible bacteria in the sediment does not rule out UTI, because approximately 10 000 rods/mL or 100 000 cocci/mL of urine must be present to be seen by the human eye in wet mount preparations of urine sediment [100]. False‐positive results for bacteria may occur because of confusion with amorphous particulate debris (e.g. cellular debris, small crystals, lipid droplets, and stain precipitate), especially when these particles experience Brownian motion. Some coliform organisms assume a filamentous morphology when growing in urine. These bacteria should not be confused with fungal hyphae (Figure 6.37). The gold standard for the diagnosis of bacterial UTI is the isolation of organisms in clinically relevant quantities based on numbers of cfu per mL of urine [115]. Examination of urine sediment that has been prepared as a dry mount and stained with Gram [116] or Wright’s‐Giemsa stain for cytology will facilitate confirmation of suspected bacterial organisms [117–119].
CHAPTER 6
Examination of Urinary Sediment
INTRODUCTION
METHODOLOGY
PREPARING URINE SEDIMENT FOR EXAMINATION
Storage and Preservation
A larger urine volume of well‐mixed urine (e.g. 3 mL) is removed per some veterinary laboratory protocols, but recent evidence suggests that any degree of amplification or concentration of elements in urine sediment is not enhanced when comparing a larger to a smaller urine volume that is processed.
Consult manufacturer booklet or calculate g using RPM and length of centrifuge arm.
Do NOT centrifuge at high speeds or g forces used to process serum or plasma tubes, as this can damage delicate urinary elements.
Decanting of the supernatant as performed in some laboratories, makes it more difficult to achieve an accurate residual volume and provides a greater risk for resuspending some sediment, especially following centrifugation of small volumes.
Centrifugation
EXAMINATION OF URINARY SEDIMENT
Staining
Advantage
Disadvantage
Stained
Unstained
Standardization of Enumeration During Urinary Microscopy
Unidentified Elements in Sediment – What to Do?
Automated Microscopy
ELEMENTS IN URINE SEDIMENT
CELLULAR ELEMENTS
Red Blood Cells
Upper or lower urinary tract
Trauma
Nephritis
Coagulopathy
Genital tract
White Blood Cells
Urinary Tract – kidneys, ureters, bladder, urethra
Inflammation
Trauma
Genital tract– inflammation (infection and sterile), neoplasia, trauma
Organisms
Bacteria
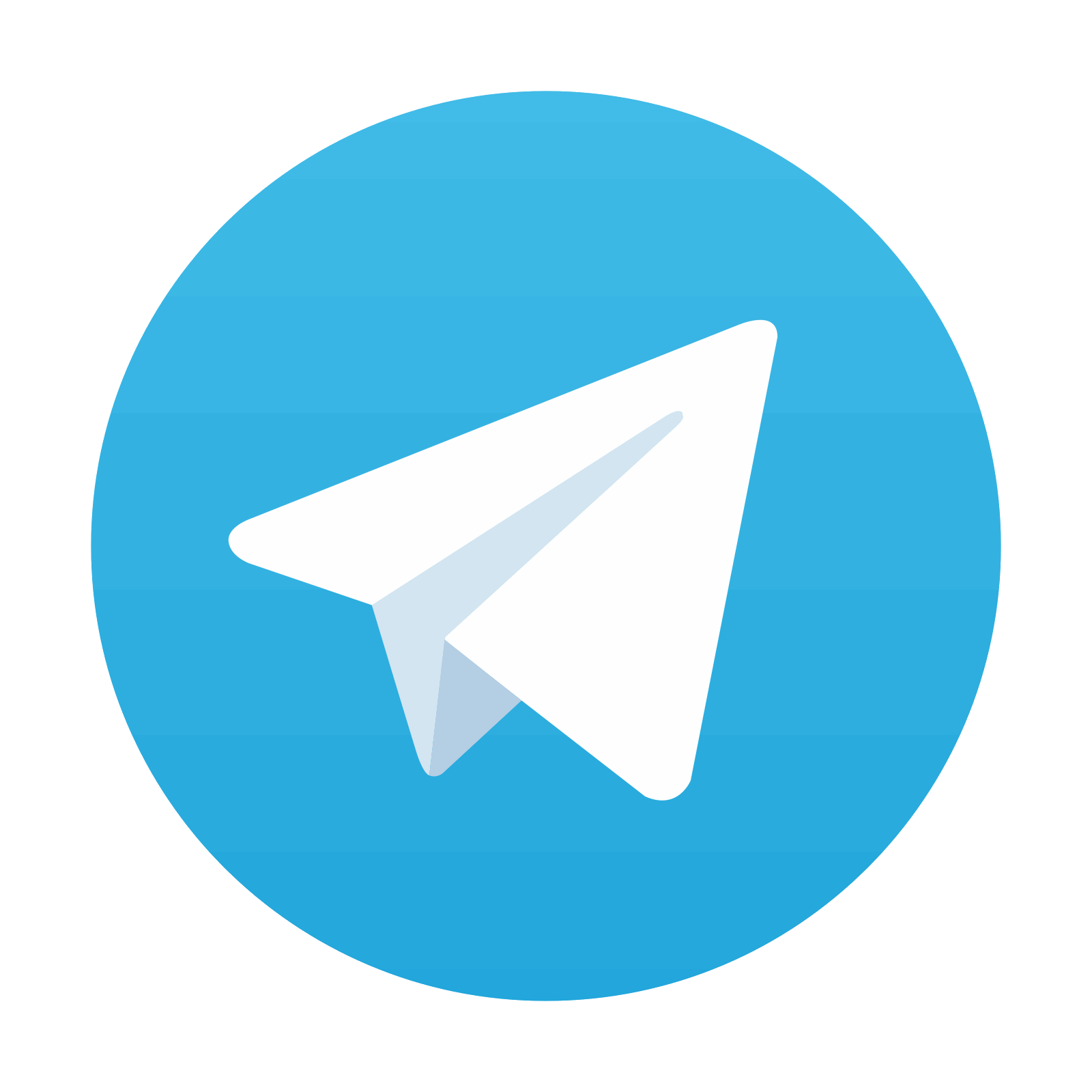
Stay updated, free articles. Join our Telegram channel

Full access? Get Clinical Tree
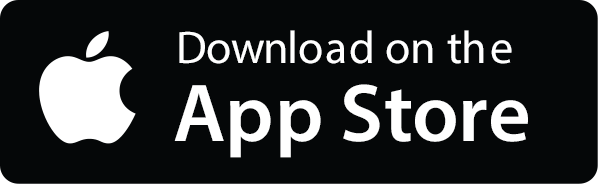
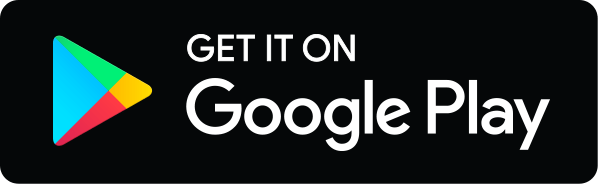