Urine chemistry provides vital information as part of the complete urinalysis, in addition to physical properties (Chapter 4) and urinary sediment findings (Chapter 6). Measurement of analytes in urine may be qualitative, semiquantitative, or fully quantitative. Benchtop analyzers generate the most accurate quantitative results for specific molecules of interest, like protein. Dry reagent strip testing, often referred to as dipstick or dipstrip testing, generates results that can be qualitative (yes or no) and/or semiquantitative. Color reactions on reagent pads can be read either by manual visual inspection or by an automated reader. Semiquantitative results are assigned a score of 0, +1, +2, or +3 within a range of possible absolute values in mg/dL (see bins concept later). For each color reaction measured, there is a range of possible analyte concentrations. Abnormalities identified from urinary dipstick testing sometimes will require confirmation with a quantitative method for some molecules, especially protein, to generate a precise value. Dipstrip testing provides rapid assessment of urine chemistry and is the most commonly used method to determine urine chemistry results in clinical practice. Dipstrip measurements can be performed at cageside or in the exam room for a quick chemical screening assessment for any obvious abnormalities (glucose +, ketones+, occult blood +) when urine becomes available. Dipstrips contain a series of chemically impregnated absorbent pads for each analyte on a cellulose or plastic strip designed to interact with urine with the potential to develop color changes (Figures 5.1 and 5.2). The intensity of the color that develops is roughly proportional to the magnitude of analyte detected, which generate semiquantitative results (Figure 5.3). This concept is different than results generated by glucose meters, in which the analyte concentration is directly proportional to the color generated following the application of blood. Dipstrips vary by manufacturer as to the number of analytes on each strip and the color expected to develop. Some dipstrips designed for human urine chemistry contain reagent pads (e.g. urine specific gravity [USG], white blood cells [WBC] esterase, and nitrites) that are of no or limited value in veterinary patients. Some systems measure and report urobilinogen and others do not. Some urinary dipstrips also contain reagent pads to detect ascorbic acid as a quality control for possible color development interference on some reagent pads (see Section 5.4.11). The same dipstrips are used to determine color reactions during both manual and automated methods. Make sure that the dipstrip matches with the autoanalyzer from the same manufacturer when using automation. The shelf life, depending on manufacturer, for reagent pad integrity on the dipstrip is around 18 months when the container remains unopened. Reagent strips should not be used beyond the expiration date noted by the manufacturer on the container. The shelf life for dipstrips is about three months after the container has been opened depending on the specific manufacturer and if the container has been quickly capped and the lid kept tight. The containers should be stored at room temperature and not refrigerated. Abnormal (positive) and normal (negative) quality controls for the dipstrip should be performed weekly, or at least when a new container of dipstrips is opened. Quality control liquid vials can be purchased that can be used with most brands of dipstrips. Distilled water should not be used as a negative control since its ionic concentration is markedly lower than that of urine, which affects reagent pad reactions. All reagent pad readings must be negative during use of the negative control. Positive reagent pad reactions must match up with the magnitude provided by the positive control [1]. FIGURE 5.1 Structure of a typical urine chemistry dipstrip. The mesh is designed to hold and protect the reagent pad in place and to allow for the rapid and even wetting of the test pad. Note that there is a hydrophobic barrier over the glucose reagent pad that does not allow urine access from the top in some strips; in these instances, urine must enter the test pad from the side. The reagent paper contains the chemical reagents that can develop color. The presence of an inert absorber paper layer between the reagent paper and the carrier strip limits the flow of excess urine and reagents that otherwise could contaminate an adjacent pad. Some pads are thicker than others due to an additional layer that minimizes interference, as might occur with ascorbic acid during measurement of occult blood (not shown). Some pads contain a layer designed to increased sensitivity of a specific analyte (not shown). Illustration by Tim Vojt, reproduced with permission of The Ohio State University. FIGURE 5.2 Side view of urinary chemistry dipstrip (ChemStrip) showing the various reagent pads prior to application of urine. The urine chemistry dipstrip measurement is ideally performed on fresh warm urine before the sample is stored or refrigerated (Table 5.1) [2–4]. Refrigerated urine samples should be brought back to room temperature since the reagent pad enzymatic reactions are temperature dependent. A delay in the analysis of stored urine runs the risk for degradation of some analytes in the urine sample. Additionally, lysis of red blood cells (RBC) during storage will alter the reading of how much of the color reaction is due to intact RBC or from released free hemoglobin. In general, the dipstrip measurements are performed on well‐mixed urine before settling or centrifugation for urinary microscopy. Failure to thoroughly mix the urine right before measurement will result in the underreporting of occult blood reactions due to intact RBC that have settled to the bottom of the urine container before urine can be applied to the dipstrip (true for manual or automated methods). Highly pigmented or turbid urine that interferes with accurate reading of color reactions on the reagent pads may clear upon settling or centrifugation, then allowing more accurate reading of color reactions on the pads when urine is applied from the supernatant in these instances. FIGURE 5.3 Hypothetical example showing the relationship between the depth of color development and its magnitude reported semiquantitatively by light reflectance. The amount of color developed is roughly proportional to the concentration of the analyte in question (see text for more details). The mg/dL noted in this example approximates the bins that are commonly measured and assigned for urinary proteins. Note that the bins overlap to some degree at the margins of the bins. This overlap can result in the assignment to either the upper or lower bin when analyte concentrations are near the margins. A second reading on the same sample using a new dipstrip may result in a different bin assignment. Different analyte concentrations within a bin range will all be reported with the same semiquantitative notation despite measured concentrations at the low end, mid‐range, or high end for that bin. Table 5.1 Performing urinary dipstrip measurement. Select a dipstrip from a container that has not expired. When removing the dipstrip from the container, make sure that the color of the negative blocks for each analyte matches up with the color chart provided by the manufacturer. Otherwise, discard that dipstrip as it is defective. The entire batch of strips in that bottle may be damaged, and it may be necessary to switch to a new bottle of fresh dipstrips [5]. Use only dipstrips that have been stored properly (tight lid and desiccant pad within), as exposure to air and moisture can affect the integrity of the chemicals in the reagent pad within a few hours [4]. Remember to immediately recap the dipstrip container after the dipstrip is removed. The use of only one brand and specific type of urinary dipstrip is recommended throughout the veterinary hospital (community practice, emergency room, specialty services, and in the central laboratory) in order to avoid confusion in results that are generated and to allow more meaningful comparison of serial results on the same patient. It is important to ensure that color charts are matched with the specific manufacturer of the dipstrip when determining the magnitude of color generation on the reagent pads as this is likely to vary. Urine can be applied to the dipstrip following immersion into urine (Figure 5.4) or by using dropper bulbs or pipettes to apply urine individually to each reagent pad. Immersion of the strip into urine should be brief in order to minimize leaching out of reagents from the pads. Insufficient volume for complete immersion into urine will occur frequently with the small urine volumes often submitted for analysis in dogs and cats. Tilting a urine tube sideways will allow the urine strip to contact enough urine that will allow urine to be absorbed into the pads by wicking action as an alternative (Figure 5.5). Following urine application, the dipstrip should be turned on its side horizontally to touch a tissue or other absorbent paper to remove excessive urine volume [6]. The dipstrip should not be held vertically after urine application. This limits the contamination of urine from one pad spilling over to the other, which otherwise could allow the movement of color reacting chemicals from one pad to the other obscuring or altering the color development on the next pad. Urinary chemistry measurement by dipstrip is most often performed in the veterinary clinic by manual visual estimation of color development, though there appears to be a trend for the increased use of partially automated instruments in primary care practices (Figure 5.6a,b). Automated readers are usually employed by referral laboratories. FIGURE 5.4 Immersion of urinary dipstrip in urine. Immersion is possible when a large enough urine volume has been submitted. Immersion should be brief so as to not leach out chemicals from reagent pads. FIGURE 5.5 Insertion of a urinary dipstrip into well mixed urine in a tube before settling or centrifugation. The tube can be tilted in samples with limited urine volume in the tube so that urine that lines the side of the tube can come into contact with the dipstrip. It is important to read the color reaction on the reagent pads at the manufacturer‐recommended times for each pad. There may be three or four different optimal times for reading depending on the reagent pad. The optimal reading times can vary from 30 seconds to 2 minutes for some reagent pad color reactions [4]. Underdevelopment of color could occur when read too early, and overdevelopment of color could occur when read too late. Automated readers are programmed to ensure that the color reaction for each pad is read at the proper time. The degree of color reaction on the chemistry dipstrip is generally proportional to the concentration of the analyte in the urine. A ranking for how much color has developed for each analyte is assigned during the manual visual inspection of the dipstrip. The color on the urine exposed reagent pad is compared to a color chart provided by the specific manufacturer (Figure 5.7). Recording the intensity or depth of color change that develops on the reagent pad by visual analysis is inherently subjective, as humans have limited ability to recognize subtle gradations in color development [7, 8]. The ability of the technician to assign this ranking may vary depending on the individual’s visual acuity and ambient lighting. Turbid or highly colored urine can interfere with the ability to manually read the color reaction on the reagent pad. Visibly pink or red urine from hematuria or hemoglobinuria, high amounts of bilirubin in the urine, drugs with dyes, excreted drugs that express color, or vitamins that can add color when excreted into urine can obscure color reactions on the reagent pad [8, 9]. FIGURE 5.6 Two commonly used veterinary automated chemistry strip readers. (a) Zoetis VetScan ® UA. Source: Zoetis. (b) IDEXX VetLab. Source: Courtesy of IDEXX Laboratories. Copyright © 2022, IDEXX Laboratories, Inc. All rights reserved. Used with permission. The color of each reagent pad is aligned with the corresponding color chart to see which color block it best matches during manual visual inspection (Figure 5.8). These color blocks are also called “bins” and contain a range of values rather than a single number. It is important to ensure that the dipstrip is compared to the color block to match each analyte from the proper end of the strip. A qualitative value is assigned to most pads as 0, trace, 1+, 2+, 3+ along with a corresponding numerical value in mg/dL or μmol/L that represents each bin. Each color block or bin contains a range of values that are reported the same whether the analyte concentration is at the low or high end of that color block, despite slight differences in color generation. For example, using the protein pad, a 2+ reading that is reported in a urine sample will also report 100 mg/dL by convention. Conceptually, the actual protein content could be 80 mg/dL in one sample and 130 mg/dL in another sample, but both will be reported at 2+. The precise upper and lower limits for each bin set of values will vary somewhat by the dipstrip manufacturer. Due to inherent variability using dipstrip methods, the same urine specimen measured twice in a row with a new dipstrip could have different color bins reported when the actual analyte concentration is very near the margin as to how that bin is assigned. A specimen could be 0 or trace, trace or 1+, 1+ or 2+, 2+ or 3+ in these instances. It has been considered acceptable to report results that are within one color block or bin above or below the actual result [8, 10, 11]. For some analytes, the bins that are associated with the color block overlap to some degree depending on the manufacturer. A different color bin assignment can occur for two values very close in concentration to each other when one value is just above and the other value just below the margin of the bin. Analytes that measure at the margins of the bins could be read at the higher or the lower bin. In this instance, a protein reading could be 2+ or 3+ despite the same value for the measured protein in mg/dL due to the inherent variability in this method of measurement [8, 10, 11]. Always consult the manufacturer’s package insert as reagent pad chemical reagents may have changed, which can have implications for clinical interpretation [5]. Factors that also affect the repeatability of reported results include the potential for varying amounts of reactive chemicals in each dipstrip pad and how urine is deposited on the pad (too little, too much, failure to wick excess after urine is applied). The temperature in the room and the temperature of the urine sample are additional considerations as enzymatic reactions within the pads are temperature dependent but most dipstrips are not temperature compensated. FIGURE 5.7 Color chart used to visually match color that develops on the various reagent pads on the dipstrip. Note that there is a notation to help ensure that the strip is held in the proper orientation before matching color reactions (Roche Cobas urinary dipstrip). FIGURE 5.8 Matching up the reagent strip color pads with the appropriate color blocks on the chart. The reagent pads are moved over the color chart blocks to determine the best match to be reported. It is essential that the dipstrip be held in the proper orientation (with the handle to the right). Color development on an individual reagent pad is not always directly proportional to the concentration of the analyte in the urine, as color development is subject to many potential errors (Table 5.2). Technicians vary in their ability to visually match the color reactions on the reagent pads with the standard color chart on the bottle, and this ability may be adversely affected by poor lighting. Turbid urine or urine that is abnormally colored due to the presence of blood, bilirubin, or certain drugs also may affect the interpretation of color reactions on the dipstrip reagent pads. All dipstrip urinary chemistry analytes except glucose were significantly affected by the amount of blood contamination in the urine from both dogs and cats in one study [12]. Measurement of dipstrip chemistry in the supernatant of a centrifuged urine sample is recommended when obvious interference from intact RBC or turbidity prevents accurate reading of reagent pad color. Other factors that may interfere with results of dipstrip testing include the temperature of the sample (e.g. refrigerated sample not allowed to return to room temperature before testing), contamination of urine by disinfectants or preservatives, interference of the color reaction by drugs in the urine sample, outdated reagent strips, contamination of reagent pads, incorrect timing for reading of reagent pad reaction, and inadvertently holding the standard color chart on the bottle of dipstrips upside down relative to the dipstrip itself. Extremes of urine pH should also be considered for possible positive or negative effects on color development of the other chemistry dipstrip pads [10, 11, 13]. This is why dipstrip readings with current technology are semiquantitative and not purely quantitative in nature. Table 5.2 Errors in dipstrip reagent pad results. To evaluate the effect of preservatives in urine, dipstrip chemistry reactions were determined using an autoanalyzer in free catch urine from healthy dogs at time 0, 24, and 72 hours after collection. Results obtained at time 0 from freshly collected urine samples were determined in plain tubes and in tubes with preservatives. Chemistry measurements were repeated at 24 and 72 hours in urine samples stored at room temperature in tubes that contained a preservative and in plain tubes stored in the refrigerator without preservatives. There were very high to high correlations for dipstrip chemistry analytes in all the samples at all time points. All dipstrip chemistry results from refrigerated plain tube samples or from room temperature samples with preservatives were similar to each other and to the time 0 measurements. Since no urine samples contained ketones or glucose, and few had occult blood reactions, conclusions about any effect of preservatives or storage conditions on these urine chemistry results cannot be made. 7–8‐mL urine is recommended to be added to tubes containing preservatives in this system to maintain the recommended ratio of urine to preservative, which could limit its use. The effect, if any, of adding smaller urine volumes to the preservative tube has not been reported [14]. Fully automated dipstrip analyzer systems are available in human medicine [15]. In these systems, urine is inserted into the instrument, and then, urine is applied to the pads on the dipstrip by the machine prior to the automated reflectance readings. Automated readers standardize the method for the reading of the color that is generated for each reagent pad at the standardized times recommended for that particular system. Automated readers objectively detect color development, which reduces interoperator variability and increases the diagnostic accuracy of the results. Partially automated analyzers are currently used in veterinary medicine, in which the technician first applies urine to the dipstrip and then inserts the dipstrip into the analyzer for measurements to commence (Figure 5.6a,b). Automated readers direct an LED beam at the various reagent pads once the dipstrip with applied urine is inserted into the analyzer. A photoelectric cell above the reagent pad determines how much light is reflected from the reagent pad, that signal is converted from analog to digital, and then, the percent reflectance is calculated by the instrument’s microprocessor. Each chemistry pad is illuminated by a specific wavelength of light, and then, the percentage that is reflected is recorded by the analyzer. Different wavelengths of light are directed to the chemistry pad depending on the specific analyte and the maker of the automated analyzer. The amount of light that is absorbed and reflected depends on how dark or light the pads are following interactions with the urine analyte. As mentioned earlier, a color change that is darker corresponds to a higher concentration of the urinary analyte. The higher the reflectance, the lower the concentration of the analyte; the lower the reflectance (greater absorbance), the greater the concentration of the urinary analyte (Figure 5.3). Algorithms within the analyzer convert the reflectance into a numeric semiquantitative value or “bin.” The reciprocal value of reflectance readings is proportional to the concentration of the measured analyte [10, 11,16–18]. Reflectance is determined with high accuracy by these instruments, but they still generate semiquantitative results due to factors other than the concentration of the analyte that influence the development of color, as discussed above. Some automated urinary dipstrip readers used in human medicine have the ability to generate results with a specific number along a continuous scale rather than one within a semiquantitative bin [4, 15]. A color compensation pad is included on some dipstrips designed for automated urine chemistry. This pad is the same material as in the other pads but without added reagents. If there is a strong intrinsic color of the urine, the analyzer includes the compensation pad to calculate a corrected final reflectance for each pad. This correction concept is similar to background subtraction, but the algorithm employed by the analyzer is more complicated. Since this is not possible to do visually, the analyzer reading in these instances is more accurate [18]. Several in‐house automated urine chemistry analyzers are now marketed to veterinarians on the premise that technician operator time will be reduced, and that the accuracy and precision of results will be greater compared to manual methods. Errors in recorded results are lessened as data are electronically captured and entered into the medical record. Variability in the reading of color pad results between different technicians is eliminated during automated chemistry measurement. In one study at a teaching hospital, the manual reading of color reactions from the urine chemistry dipstick by the technician did not differ from those generated by the automated analyzer in any canine urine sample [19]. Similar findings were found in other studies when dipstrip reading was performed by experienced veterinary technicians [20, 21]. Such a high concordance between readings from the automated analyzer and manual reading by less experienced technicians is not as likely. In another study, a high degree of discordance was observed between visual urinary dipstrip readings and those by an automated reader using the same dipstrips in one veterinary study of pooled urine samples from dogs with varying amounts of glucose, protein, and RBC. Precision was higher during automated reading of urinary dipstrips compared to visual reading [22]. Poor precision in this study was attributed to lack of sample mixing (visual and automated), not blotting the dipstrip after dipping into the sample, and not reading the color reactions at the manufacturer‐recommended time when performing visual analysis. A surprising number of operators held the strip horizontally instead of vertically while matching the color blocks [22]. The use of automated analyzers to measure urine chemistry by dipstrips is advocated over manual visual methods in order to increase the accuracy of reported results and to increase work flow efficiency, though results generated can vary by analyzer [10, 11, 21, 23]. There was high agreement between automated results using a proprietary veterinary analyzer and visual inspection results in one white paper [11]. There was good agreement between dipstrip results using a different proprietary veterinary automated reader compared with a high‐end human dipstrip analyzer in another white paper [10]. Accurate reporting and transmission of results is ensured with automated methods, whereas transcription errors sometimes occur during the recording of results following manual reading [4, 11, 20, 21, 24]. There was excellent concordance between results from automated urine chemistry (Cobas u411 analyzer with Roche Chemstrip 10 UA strips) and manual methods, after altering reflectance programming for urine from the dog and cat. This automated method is used in high‐throughput laboratories and is not designed for in‐house analysis [25]. In normal animals, most dipstrip reactions will be negative (Figure 5.9). The detection of glucose and ketones in urine often indicates excessive generation of these molecules in the body from a systemic disease process. Alterations in urinary pH, protein, and occult blood can reflect disorders of the upper and lower urinary tract, as can the presence of glucose during some types of acute kidney injury (AKI) (rarely during chronic kidney disease [CKD]). Details for individual analyte reagent pad reactions are provided in the subsections that follow. Some of the urinary analytes on the dipstrip designed for humans are not accurately measured in the urine of dogs and cats, such as USG, nitrites, and LE, and are best not recorded to avoid confusion. FIGURE 5.9 Expected results from physical properties of normal urinalysis, recorded on a standardized form. The magnitude of color reactions that develop on any reagent pad should be compared to those on all the other reagent pads, as color reactions on one pad could indicate factors that could influence color development on other reagent pads. Urinary physical findings (USG, color, and clarity) and urinary microscopy results should also be integrated into the interpretation of readings on the urinary chemistry dipstrip to maximize interpretation [1, 26]. It is important to remember that clinical decisions from urinary chemistry dipstrip measurements are made in the context of multiple partially flawed results (Wamsley 2020 personal communication). Further value in the assessment of positive dipstick reactions can sometimes be gained when findings are integrated with the degree of urine concentration (USG). For example, a trace finding may be normal in a urine sample with a >1.050 USG, whereas it could assume clinical significance when the USG was 1.020. Smaller quantitative reactions in dilute urine are potentially of more significance than similar reactions in highly concentrated urine. Abnormal results on dipstrip chemistry should be verified by repeat measurement, especially when equivocally positive results were initially identified (e.g. trace protein or trace glucose). Greater assurance for a true abnormality is gained when the second dipstrip generates a result in the same bin (both are trace or both are 1+). Some discrepancy between a dipstrip measurement and that generated from a benchtop instrument is expected due to the inherent differences in the methodologies employed (visual or reflectance color determination on a pad compared to the transmission colorimetry of urine). This discrepancy is most common for the protein value estimated on the dipstrip compared to that generated from the benchtop analyzer. Clinical decisions that require a quantitative value, such as urinary‐protein‐to‐creatinine ratio (UPC) for proteinuria, cannot be made from the urinary dipstrip protein estimate alone. Abnormal urine chemistry results determined by dipstrip should be considered a screening procedure to be confirmed by quantitative testing using another method if precise results for that analyte are considered important. The findings of proteinuria and hematuria on dipstrip chemistry are encountered incidentally at times during wellness screening of seemingly healthy animals that have underlying disorders not yet severe enough to create obvious clinical signs. Abnormal results for urine dipstrip measurements were common in apparently healthy geriatric and senior dogs of one study. Positive reactions for protein were detected in 79%, 84% for bilirubin, 19% for occult blood, 38% for urobilinogen, 1% for nitrite, and 5% for LE in these samples [27]. Trace positive reactions for glucose, ketones, and urobilinogen were detected in 3% of apparently healthy middle‐aged and older cats. In another study of younger dogs (median five years of age), all were negative for glucose and ketones, 5% had a positive reaction for occult blood, 65% had trace (15%) or higher reactions (50%) for protein, and 55% had positive reactions for bilirubin [14].Occult blood reactions were positive in 28%, 99% were positive for LE, while zero of these cats had reactions for bilirubin or nitrites. Based on UPC, borderline proteinuria was common in this population of cats, but comparison to dipstrip reactivity was not provided [28]. Having pet owners monitor dipstrip color reactions at home in these patients may be helpful in these cases (glucose, ketones, pH, blood), but it is also problematic to ensure the accuracy of the reading due to issues with operator training and precise timing for the reading of the dipstrip by the owner. An at‐home kit with an embedded urinary dipstrip is available that allows interaction with voided urine from dogs. A smartphone and an app were used to record and score color reactions, but, unfortunately, this system provided unreliable results in one study [29]. Abnormalities on dipstrip chemistry frequently align with abnormal findings from urine microscopy. For example, with positive reactions for occult blood and protein on the dipstrip, urine microscopy will often detect RBC sometimes in association with WBC and bacteria. Protein positive status as the only abnormality on the dipstrip can occur with no microscopic abnormalities in urinary sediment, as often is the case with glomerular proteinuria. Conversely, normal findings on dipstrip chemistry do not guarantee an inactive sediment on urine microscopy. Casts, tubular epithelial cells, urothelial cells, WBC, fungal and bacterial organisms, and crystals are the findings on microscopy that are not detected by the dipstrip that can be detected during urinary microscopy (see Chapter 6). The integration of findings from physical properties, dipstick urine chemistry, and urine microscopy provides the most meaningful clinical information, more than any of these components of the complete urinalysis alone. Urine pH measures only free hydrogen ions and not hydrogen ions buffered with phosphate or with ammonium in the urine [5] and can provide a crude assessment for systemic acid–base status. The appropriateness of the urinary pH measurement can provide clinically important information when integrated with other findings. An increase in urine pH can develop when urine samples stand in open containers exposed to air at room temperature for long periods of time, allowing loss of CO2. The pH of uncontaminated urine stored at room temperature for several hours in a sterile closed container does not change appreciably. In one study of urine from dogs, pH values determined by dipstrip did not change from time 0 (initial collection) to 24 or 72 hours later whether stored at room temperature in preservative‐containing tubes or in plain glass tubes in the refrigerator. Median pH was 7 with a range of 6–8.5 in these samples [14]. In another study of dogs, there was no significant effect of storage time (0, 6, and 24 hours) or temperature (room or refrigerator) on urinary pH determined by meter in urine [30]. It is likely that increased pH would occur in urine samples that are exposed to air for long periods or that are mixed with air (allowing loss of CO2), compared to storage under anaerobic conditions. Extremely alkaline urine (≥8.5 pH) can also be an artifact when there is contamination of the urine, urinary container, or dipstrip with alkaline detergents (e.g. ammonium or bleach‐containing) and surgical scrubs [1, 26, 31, 32]. Contamination with alcohol, benzalkonium, or betadine surgical scrub material potentially increases urinary pH when urine samples are obtained by expression while under anesthesia, but these effects have not been detailed. Highly alkaline urine may impact findings from urine microscopy due to accelerated degeneration of free cells in the urine and cells within casts [26]. Additionally, the matrix protein within casts is more soluble under conditions of alkaline urine, which can decrease the chances to observe casts that were formed but that broke up quickly. The test pad on the dipstrip is impregnated with various combinations of reagents that produce color gradations depending on the urinary pH of 5–9. Most test pads include a mixture of methyl red and bromothymol blue resulting in shades of orange, yellow, green, and blue. The lowest pH (5.0) generates orange color followed by yellow and green for intermediate pH values, and the highest pH (8.5) generates blue [4, 8, 33]. The color that develops on the reagent pad is compared visually to a color chart provided by the dipstrip manufacturer immediately following the application of urine to the dipstrip. The pH that is recorded is the one that most closely matches the color block on the chart and is estimated to the nearest 0.5 or 1.0 pH unit depending on the manufacturer of the dipstrip. The measurement of pH by dipstrip is considered accurate to within 1 pH unit (±1 unit) when compared to pH meter [8, 26], a high level of discordance that is not generally appreciated in veterinary medicine. Reporting urinary pH to the nearest 0.5 pH unit can lead to the false impression that the dipstrip pH measurement provides a high level of accuracy and precision (Heather Wamsley personal communication 2019). For this reason, some urinary dipstrips are manufactured that provide pH to the nearest whole unit pH value instead (e.g. 6, 7, and 8). Historically, urine pH by dipstrip has been considered useful in providing an approximation of urine pH during routine urinalysis [34, 35]. Unfortunately, the estimation of urine pH by dipstrip shows only poor‐to‐moderate agreement with pH measured by meter. The measurement of urine pH by dipstrip has been described as inherently inaccurate and its continued use is one of convenience instead of accuracy [36]. Consequently, urine pH by dipstrip often is not accurate enough to make informed clinical decisions in many disorders. Urine pH by dipstrip in spot urine samples was compared to pH measured by an electrode in 24‐hour urine samples from humans. Greater inaccuracy was found in urine samples with a spot urine pH > 6.5, but pH by dipstrip was associated with a large range in pH by electrode in this study. Based on these findings, urine pH by dipstrip from spot urine was considered unacceptable in the monitoring and management of human patients with urolithiasis [37]. The inaccurate determination of urine pH by dipstrip could also result in faulty treatment strategies to alter urinary pH to prevent urolithiasis. For example, the dipstick method tended to overestimate pH in one study of dogs suggesting the presence of alkaline urine when the urine was mildly acidic by pH meter [35]. Urinary pH by dipstrip reported acidic urine in cats when readings by pH meter indicated a neutral to mildly alkaline urine in some cats [34]. The clinical interpretation of inaccurate urinary pH readings by dipstrip is most likely to occur when pH values are near neutral [38]. Urine pH values more removed from neutral are likely to align in the same bin when measured by meter (high–high or low–low) despite differing actual values. When measured by dipstrip, visible blood contamination lowered the score assigned to urinary pH in both dogs and cats of one study, though the magnitude of change was considered small. Urinary pH was significantly lower in red urine samples from dogs and cats, and in combined red (light pink, dark pink, and red) samples from cats [12]. Extremes in urinary pH have the potential to alter color development on other reagent pads on the dipstrip, as can occur with a high urine pH and an increased reaction for protein in urine that is highly concentrated. Also, contamination of the pH pad with acid buffer flowing from the protein reagent pad during poor technique is a consideration for a falsely low urinary pH [12, 39]. Automated readers are available to read dipstrip results and may provide more consistent and more accurate readings of urinary pH. Pooled urine samples from dogs that were acidic, neutral, or alkaline were measured by dipstrip for pH by visual and by automated methods to detect color development. Compared to urinary pH by meter as the gold standard, accuracy for pH readings was higher with the use of automated readings compared to that recorded following visual analysis assessed by multiple operators for all the samples [22]. In another study, there was good agreement between urinary pH determined by visual assessment and an automated reader in urine from dogs of one study (a pH meter was not used for comparison) [20]. Similarly, correlation between urinary pH by visual reading and those by an automated reader was considered excellent in another study of urine from dogs and cats [21]. The gold standard for the measurement of urinary pH is provided by either benchtop or portable pH meters that have been properly maintained and calibrated [14, 30,34–36]. If accurate measurement of urine pH is important in clinical decision‐making, a pH meter should be used [26,34–36, 38]. Relatively inexpensive portable pH meters can be used in‐house, or a refrigerated urine sample can be shipped to a reference laboratory within 24 hours of collection to obtain an accurate urinary pH [30, 35]. There can be significant discordance between urinary pH measured by dipstrip and pH meter. A pH meter can be used to confirm or determine more accurate results, particularly when pigmenturia obscures or alters test pad color [32]. The degree of agreement for urinary pH was compared between a benchtop pH meter, two portable pH meters, urinary dipstrip, and pH paper in urine from normal cats. The expiration of the electrodes on one of the portable pH meters accounted for a negative bias on the pH readings. The pH paper had poor agreement, and dipstrip pH readings had intermediate agreement with pH measured by the benchtop analyzer. The pH measured by dipstrip had an average negative bias of −0.12 pH units compared to the benchtop analyzer, but displayed much greater discordance at lower pH values on the dipstrip. The difference in urine pH measured by the benchtop analyzer after 24 hours of refrigeration (mean increase of 0.04) compared to time 0 was not considered clinically relevant. Electrode maintenance and daily calibration of portable pH meters is important to ensure the accuracy of test results [34]. In another study of urine from hospitalized dogs, urinary pH was compared between a benchtop pH meter, four portable pH meters, urinary dipstrip, and pH paper. Three of the four portable pH meters provided nearly identical results to that from the benchtop meter. However, there was moderate‐to‐poor agreement for urinary pH results determined by dipstrip or pH paper compared to the benchtop meter [35]. Mean urinary pH measured by dipstrip was significantly higher (7.11 ± 0.12) than when measured by pH meter (6.83 ± 0.12) in urine from dogs; urinary pH did not change when analyzed before or after centrifugation [40]. In cats, urinary pH by dipstrip was consistently lower compared to measurement by pH meter; a consistent error in direction of pH was not found in dogs of the same study. The authors of this study suggested that pH by dipstrip should be within ±0.25 pH units of that measured by pH meter in order to be clinically useful, but this only occurred in 40% of the samples analyzed. A dipstrip pH of 7 corresponded to a pH meter pH of 6.2–8.2 [36]. In another study in cats, a dipstrip urinary pH of 6 corresponded to a meter pH of 5.81–7.18 [34]. The normal urinary pH of dogs and cats is generally considered to be from 5.0 to 7.5 [41, 42] or from 6.0 to 7.5 [3, 43] depending on age, nutrients in the diet, food intake (ad libitum or meal fed), and timing of the urine sample collection. The mean urinary pH of 5.1 ± 0.3 in one day old Great Dane puppies significantly increased on day 5 of age to 5.3 ± 0.6. The initially low urinary pH was attributed to a combination of respiratory and metabolic acidosis in newborn pups [44]. Bone growth of the young is associated with the production of calcium apatite and protons that are excreted into urine, which decreases urinary pH. When bone growth is finished, urinary pH tends to increase [36, 45]. The possibility for the elaboration of slightly more acid urine following the sleeping hours and mild respiratory acidosis [42] has not been critically evaluated in dogs or cats. The amount and type of protein, acidifying or alkalinizing minerals [46], and organic anions and cations in the diet contribute to urinary pH. Decreased urinary pH is expected when eating higher concentration of proteins, especially those with sulfur‐containing amino acids, which generate acid products following metabolism (Table 5.3). The consumption of organic cations usually increases the urinary pH (Table 5.4). The ingestion of diets based on cereal or vegetables may generate alkaline urine due to the excretion of alkaline end products following metabolism. Urinary pH is lower in young cats compared to adult cats eating the same diet. Urinary pH tends toward neutral to slightly acidic during fasting of normal animals, as demonstrated by the finding of a urinary pH by meter of 6.5–7 regardless of the baseline pH when normal adult cats were fasted for three days [45]. Table 5.3 Causes of acidic urine. Table 5.4 Causes of alkaline urine. An increased urinary pH of varying magnitude following food intake is sometimes observed in dogs and cats. This transient increase in urinary pH has been attributed to the so‐called postprandial alkaline tide as a response following gastric acid secretion [42], but this explanation appears too simplistic [47]. A fall in urinary acid excretion was still seen following food intake despite blockade of gastric acid secretion in one study of humans, so the mechanism for the generation of this alkaline urine remains unclear. Also, a decrease in urinary acid excretion was seen after the feeding of high compared to lower concentrations of dietary protein, fasting, or ingestion of water alone [47]. The postprandial alkaline urine effect can be protracted, and it may require 8–12 hours fasting after feeding for more acidic urine to return. For these reasons, the timing of the urine sample collection in relationship to eating or fasting should be factored into the interpretation of neutral to alkaline urinary pH. An abnormal urine pH (>7.5 pH) was detected in 17.4% of samples from dogs and in 8.2% of samples from cats at a veterinary teaching hospital. Of these abnormal pH samples, 52.3% from the dogs and 74.4% from the cats were subsequently found to be associated with some microscopic abnormality [41]. A wide range of urinary pH was encountered in dogs evaluated at a teaching hospital, from 5.0 to 9.0 as determined by visual inspection of the color reactions. Urinary pH was 8–9 in 40% of these samples and 5.0 in 24%. A pH of 6.5–7.0 was encountered in 22% of the samples [48]. Urinary pH is not a reliable indicator for the precise assessment of acid–base status, as there are many instances where urinary pH is discordant from systemic acidemia or alkalemia. Urinary pH is influenced by acid–base balance, but this is not a simple function of the blood pH, as this can be affected by systemic disease, renal tubular health (e.g. CKD and AKI), effective circulating blood volume, and concurrent electrolyte disorders. In general, metabolic and respiratory acidosis result in aciduria, whereas metabolic and respiratory alkalosis result in alkaline urine following compensatory mechanisms. The optimal interpretation of urinary pH is made when blood gases are available to fully characterize the nature of the acid–base disturbance. Paradoxical aciduria occurs in some dogs and cats that have metabolic alkalosis in association with the contraction of circulating blood volume, along with the depletion of sodium, potassium, and chloride as can occur during gastrointestinal (GI) obstruction. In these instances, alkalinuria develops as a compensatory response to restore normal acid–base balance following fluid therapy to restore circulating volume and chloride and potassium repletion. Paradoxical alkalinuria potentially develops in states characterized predominantly by hyperkalemia in which the translocation of potassium into and hydrogen ions out of cells occurs. Uncommonly, distal renal tubular acidosis may be responsible for alkaline urine or an inability to maximally acidify urine. Because of these variations, evaluation of urinary pH by itself can be misleading and lead to the wrong characterization of an acid–base disturbance. Intermittent alkaline urine was documented in a cat that was fed an acidifying diet, likely due to stress‐induced respiratory alkalosis during visits to the veterinary hospital [49]. Drugs may affect urine pH, and the drug history of the animal should be considered when evaluating urine pH. Urinary acidifiers (e.g. ascorbic acid, d,l‐methionine, and ammonium chloride) result in the production of acidic urine. The administration of alkalinizing agents, such as sodium bicarbonate or citrate, and carbonic anhydrase inhibitor diuretics (e.g. acetazolamide) causes the formation of alkaline urine. Urinary pH often aligns with certain types of crystals in urinary sediment. For example, urate, cystine, and calcium oxalate crystals are more apt to form in acid urine, whereas struvite tends to form in alkaline urine. The prediction of the urolith type in the absence of quantitative stone analysis is facilitated by knowing the urinary pH in addition to breed, age, sex, and radiodensity of the stone. Manipulation of urinary pH is often attempted to try to prevent the recurrence of urolithiasis based on crystal type and solubility characteristics. Urinary pH may not indicate how much acid is being excreted by the kidneys in some instances, if the urine is changed after renal processing [50]. Urinary tract infection (UTI) with urease‐producing bacteria (Proteus spp, Staphylococcus aureus, Mycoplasma spp) can be associated with persistently alkaline urine, often with urinary pH ≥ 7.5 and as high as 9.0 in some dogs. Many bacterial UTIs, however, do not result in alkaline urine and exist in neutral or acid urine [51]. Alkaline urine may also develop if a sample of normal urine is contaminated with urease‐producing bacteria. If this urine is allowed to stand at room temperature, bacteria proliferate and urea can be hydrolyzed, resulting in alkaline urine the same as happens with a real urinary infection. Protein in the urine can be measured by qualitative, semiquantitative, or quantitative methods (see Chapter 7 for details). Reagent dipstrip pads allow qualitative and semiquantitative colorimetric determination if the reaction on the pad is read at the appropriate time interval. Standard reagent strips estimate the protein concentration in urine, the magnitude of which is influenced by the degree of urine concentration (USG, Uosm), which is importantly dependent on the patient’s state of hydration. Consequently, results from urinary reagent pads for protein only provide a rough assessment as to whether or not there is pathological proteinuria [4]. Increasing protein concentrations in the urine cause the chemical indicator in the reagent pad (tetrabromophenol blue or tetrachlorophenol‐tetrabromosulfonphthalein) to change color [1, 4, 5, 32]. A positive reaction on the reagent pad does not identify a particular protein, but the indicator is much more sensitive to albumin than to globulins, Bence Jones protein, mucoproteins, hemoglobin, myoglobin, and other nonalbumin proteins [4, 8, 32, 33, 50, 52]. The color change on the reagent pad is based on the ability of free amino groups of proteins in the urine to bind to the color indicator, which varies by specific protein. This test pad detects albumin most readily since albumin has an abundance of free amino groups compared to other proteins in urine and so develops the strongest color. Nonalbumin urinary proteins can be detected if they have enough free amino groups and the protein concentration is high enough. Consequently, a negative reagent pad reaction for protein does not automatically exclude the presence of these nonalbumin proteins [1, 5, 32, 38, 53]. Specific bins for the degree of color development on the reagent pad and the protein concentration reported vary by manufacturer, especially at the trace and maximal amount of protein that is reported. Albumin in the urine is the protein of greatest interest in most clinical cases, as it is the predominant urinary protein in disease and in health [1, 32, 38,54–58]. The gradations in color intensity for each block/bin of protein reagent pad on the dipstrip are subtle making it difficult at times to assign the proper block during visual inspection. It appears likely that the assignment of the proper color block for urinary protein has the most errors of any pad on the dipstrip when assessed manually using visual inspection. Any abnormal color of the urine (hemorrhage bilirubin) also makes it difficult to impossible to properly read the color that develops on the reagent pad [3]. The lower limit of sensitivity for detection of proteinuria using conventional dipstrips is approximately 10–20 mg/dL for a trace reading on the reagent pad, depending on the specific manufacturer. The ability to detect protein in urine (sensitivity) depends on the type and proportion of urinary proteins [4]. Maximal intensity color reaction occurs at protein concentrations ≥2000 mg/dL, ≥1000 mg/dL, ≥600 mg/dL, 500 mg/dL, or 300 mg/dL depending on the specific dipstrip manufacturer. In general, the protein concentration of urine measured by dipstrip is reported qualitatively as negative (<10 or 15 mg/dL), trace (10–20 mg/dL), 1+ (30 mg/dL), 2+ (100 mg/dL), 3+ (300 mg/dL), or 4+ (1000 mg/dL). 1+ and 2+ reactions are the most consistent reagent pad determinations among different manufacturers. Due to difficulty in the interpretation of trace protein reactions, the reporting of trace values can be suppressed by some laboratories [1]. Visibly bloody urine can result in the reporting of false‐positive protein results from discoloration of the reagent pad [8, 12]. A repeat dipstrip measurement using urine supernatant after RBC have settled by gravity or after centrifugation can lessen the discoloration of the reagent pad. The dipstrip reagent pad for protein did not develop a positive reaction (100 mg/dL) until the hemoglobin concentration was >5 mg/dL in one study from dogs. Less than maximal reactions for hemoglobin on the dipstrip at the same time that the protein dipstrip is positive indicates that the protein being detected on the reagent pad cannot be attributed to hemoglobin [52]. According to one package insert, false‐positive reactions are also noted to occur when reagent pads are exposed to strongly alkaline urine (>8.0 pH), following treatment with some intravenous (IV) blood substitutes, and when the urine sample has been contaminated with disinfectants (quaternary ammonium or chlorhexidine) that activate color development on the reagent pad (Table 5.5) [33]. False‐negative results for reagent pad protein can occur in urine that is highly acidic (pH < 6.0) or urine that is minimally concentrated or dilute (1.001–1.017 USG). Results on the reagent pad do not appear to be affected by turbidity, radiographic contrast agents [59], most medications, and preservatives that are in the urine sample. Small amounts of protein are excreted into urine by the normal dog and cat, which often are read as trace to 1+ on the reagent pad [32, 54, 55, 57, 58]. Highly concentrated urine magnifies the concentration of small amounts of protein normally found in the urine [3, 43]. The protein reagent pad was negative in 21%, trace in 27%, and positive at ≥1+ in 52% of 97 apparently healthy senior and geriatric dogs [27]. Mean dipstrip proteinuria ≥30 mg/dL was not detected in any voided urine sample from healthy Great Dane puppies of 0–28 days of age. Trace reactions for protein were detected in 65.5% of these urine samples at 10 days of age [44]. Most of the protein in urine from normal and diseased animals is albumin [32, 38]; small amounts of globulins from the urethra and genital tract may be detected in samples collected by voiding from normal animals. Randomly collected, voided urine samples from normal dogs contained 0–56 mg/dL of protein as measured by a urinary dipstrip or heat precipitation [60]. In another study of 31 normal dogs, positive urinary dipstrip reactions for protein recorded as trace (15 mg/dL) or 1+ (30 mg/dL) were common. This resulted in the recommendation that trace to 1+ reactions for protein on the dipstrip be considered within the reference range for dogs and not automatically be considered a false positive. By benchtop methods, the urinary total protein ranged from 3.0 to 39.0 mg/dL with a mean of 20.4 mg/dL, median of 15.0 mg/dL, and with the highest UPC at 0.21 [61]. When the dipstrip for urinary protein is ≥2+ regardless of USG, the UPC will usually be >0.4 for the cat or >0.5 for the dog. In minimally concentrated or dilute urine, a trace or 1+ dipstrip reaction for protein may indicate clinically relevant proteinuria when further assessed by UPC or microalbumin (MA). In samples with isosthenuria or very dilute urine (1.001–1.017), the dipstrip may register as negative or trace despite the presence of clinically relevant proteinuria due to the lower of limit of sensitivity (10–20 mg/dL) of the reagent pad. Table 5.5 Errors in the protein measurement on dipstrip analysis. The accurate interpretation of proteinuria necessitates integration of findings from the history, physical examination, urinary sediment activity, degree of urine concentration, and method of urine collection (Table 5.6). Postrenal proteinuria (UTI, stones, neoplasia) is most commonly encountered, renal origin is second in frequency, and prerenal proteinuria is uncommon in dogs and cats with clinical disorders. The origin of proteinuria as prerenal, postrenal, genital, glomerular, or tubular cannot be determined by the magnitude of the proteinuria in isolation on the dipstrip reagent pad. Persistent proteinuria associated with a normal urinary sediment (inactive sediment with few WBC, RBC, nonsquamous epithelial cells, no bacteria) or a sediment with excessive numbers of hyaline and granular casts is the hallmark of glomerular disease (e.g. glomerulonephritis, glomerular amyloidosis, glomerulosclerosis). Proteinuria associated with hematuria or pyuria does not allow anatomical localization, as inflammation or hemorrhage could occur anywhere along the urinary or genital tracts, allowing the entry of plasma proteins into the urine. Marked hematuria can be associated with the detection of proteinuria on the reagent pad, while disorders causing substantial pyuria can result in concomitant mild‐to‐moderate proteinuria by reagent pad. If the sediment is active and proteinuria is mild to moderate, consider inflammatory renal disease or disease of the lower urinary or genital tract to account for the proteinuria [62, 63]. Table 5.6 Causes of pathologic renal proteinuria. See Chapter 7 for details about renal and nonrenal proteinuria. Urine protein results should be integrated with the degree of urine concentration (USG) for the interpretation of whether the proteinuria is likely to be pathological or not. Higher protein concentrations in mg/dL are expected from urine samples with a high USG, and lower protein concentrations are expected from those with minimal urine concentration. For example, when the same amount of protein is excreted into urine, the protein may be measured by dipstrip at 30 mg/dL when the USG was 1.015 and at 300 mg/dL when the USG was 1.055. In another example, a trace or 1+ proteinuria (30 mg/dL) in a urine sample with a USG of 1.007 may be clinically relevant. The same 1+ proteinuria via reagent pad in a urine sample with a USG of 1.065 is less likely to be clinically relevant as a consequence of the concentration of urinary proteins following the removal of water by the kidneys in such a highly concentrated urine sample. Any positive protein pad color reaction in patients with minimally concentrated or dilute urine (1.001–1.020) may be clinically relevant and should be further investigated with UPC [1, 5, 32, 43, 53]. Trace and 1+ dipstrip reactions are the most problematic, regardless of the degree of urine concentration, to know if they indicate pathological proteinuria or not without confirmatory testing (UPC or MA). Dipstrip protein ≥2+ are usually associated with pathologic proteinuria and albuminuria. In some commercial veterinary laboratories, a trace or 1+ positive color reaction on the dipstrip is confirmed with sulfosalicylic acid (SSA) precipitation testing. If both are positive, then the proteinuria is deemed to be “real.” Other laboratories do not confirm the dipstrip reaction with SSA, but rather recommend a UPC to verify the significance of the positive pad reaction [38]. Quantitative methods for protein determination using the UPC are recommended to confirm proteinuria detected by dipstrip reagent pads. Urinary electrophoresis can be helpful to identify globulins entering urine, especially in cases with multiple myeloma. Since urinary protein excretion can display biological variation [4], it should be determined if findings from reagent pad positive reactions for protein are repeatable on subsequent urine samples. Alternate methods to measure protein as well as causes and interpretation of proteinuria are discussed in greater detail in Chapter 7. Glucose in health is almost entirely reabsorbed from glomerular filtrate by the proximal tubules following filtration from plasma, leaving small quantities of glucose that are excreted in urine. The amount of glucose (mg/min) that appears in urine is the difference between the filtered load of glucose (circulating glucose (mg/dL) × glomerular filtration rate (GFR) (mL/min) = mg/min) and its subsequent rate of tubular reabsorption (mg/min). The filtered glucose is extensively reabsorbed by sodium‐glucose cotransporters along the luminal surface of the proximal tubular cells in those with normal blood glucose concentrations and normal tubular function. The final concentration of glucose in the urine (mg/dL) depends upon this excretion rate plus the amount of water reabsorbed by the tubules during the urinary concentrating process. The maximal ability of the proximal tubular cells to reabsorb filtered glucose is called Tmax and is expressed as glucose reabsorbed in mg/minute. When plasma glucose is normal, the filtered load into tubular fluid in mg/minute is below Tmax, and therefore, most of the filtered glucose will be reabsorbed. When the plasma glucose is high, the filtered load of glucose will be high and can exceed the ability of the proximal tubules to reclaim this increased level of filtered glucose. Biological splay of tubular function leads to variability in reabsorptive capacity within an individual animal’s nephron population. This means that glucose can appear in the urine at a lower level of blood sugar than that associated with Tmax and is called the renal threshold. The renal threshold will vary somewhat by the genetics of individual animals, and is generally associated with a blood sugar that exceeds 170–220 mg/dL in dogs and that exceeds 260–290 mg/dL in cats [32, 39, 43, 64, 65]. The renal threshold for glucosuria to develop in the cat occurred at approximately 270 mg/dL of circulating glucose, with near total reabsorption of filtered glucose at lower levels in one study. Unlike in other mammals, a Tmax for glucose did not seem to exist [66]. The renal threshold for glucose in the dog was found to be considerably lower at180–200 mg/dL in one study [67]. The renal threshold and Tmax for glucose can differ between health and disease. As an example, Tmax and renal threshold are increased in some humans with diabetes mellitus (DM), especially those that are elderly and with increased body mass. This means that higher levels of blood glucose are required before glucose starts appearing in the urine. Insulin treatments have variable effects in lowering tubular glucose reabsorption between humans that are normal and those with diabetes. These phenomena have not yet been investigated in veterinary patients with diabetes [68]. There are also diseases that result in a decreased Tmax or renal threshold due to reduced expression or function of the sodium‐glucose cotransporter (e.g. tubulopathy from AKI, Fanconi syndrome). In these instances, glucosuria will be apparent at lower concentrations of blood glucose. This is detailed later in this section. Urine glucose concentrations from a hospital population of dogs demonstrated less than 40 mg/dL of glucose in over 80% of these patients when measured by methods suitable for small concentrations of glucose [69]. The mean urine glucose from 75 control bitches in another study was 0.27 ± 0.17 mmol/L (4.9 ± 3.1 mg/dL) [70]. When urine from healthy dogs was measured using a sensitive enzymatic kit, the median urinary glucose was 0.39 (0–1.55) mmol/L (7 mg/dL with range 0–27.9 mg/dL); 2.2% of the samples tested at zero. There was no effect of age, castration status, gender, obesity, or feeding in this study of normal dogs [71]. Urine glucose was measured in urine from cats without systemic disease using the same sensitive method for glucose measurement as in the dog study. All cats except one cat had detectable urinary glucose by the kit method, and no cat had glucose‐positive status on the urinary dipstrip from the same sample. A median of 0.389 mmol/L (7 mg/dL) of glucose was detected with a range of <0.11 to 1.1665 mmol/L (<2 to 21 mg/dL). In addition to the glucose oxidase reagent strip methodology for glucose detection, a copper reduction tablet methodology is available to measure glucose and other reducing substances in urine. The reduction of copper from the cupric to cuprous state results in a color change proportional to the amount of reducing substances in the urine. This method is not specific for glucose, and other reducing substances (e.g. fructose, lactose, galactose, maltose, pentoses, glucuronic acid, salicylates, penicillin, cephalosporin, formaldehyde, and chloral hydrate) also may cause a positive color reaction [5, 69
CHAPTER 5
Chemical Properties of Urine
INTRODUCTION
USING THE DIPSTRIP
Steps for performing urinary dipstrip measurement
Warm refrigerated urine to room temperature first.
Keep fresh urine sample ready (preferred over stored)
Make sure that only one brand of dipstrips is available to use throughout the hospital
Check weekly recording of negative and positive quality controls
Ensure dipstrips are not expired
Wear gloves
Ensure good room lighting
Open container and remove dipstrip
Do not touch reagent pads with fingers or gloves
Recap container immediately
Apply urine to strips (immersion of strip into urine or drop bulb technique)
Quickly remove urine from immersion
Apply dipstrip in horizontal position to absorbent paper to remove excess urine
Use stopwatch timing to know when to measure reagent pad color (times vary by pad)
Record color reactions as negative or plus and the mg/dL for most pads (not pH)
READING THE DIPSTRIP
Urine contamination or interfering substances
Extremes of urine pH
Extremes of urine concentration or dilution
Prolonged storage before measurement
Exposure to UV light
High levels of ascorbic acid in urine (importance yet to be investigated)
Turbidity of urine obscures the pads
Highly colored urine (blood, hemoglobin, bilirubin, drugs, vitamins) obscures pad
Contamination from disinfectants, bleach, food, feces
Handler errors
Failure to return urine to room temperature after refrigeration before measurement
Reading color development on pads at incorrect times
Inadvertently matching the dipstrip colors to the color chart from the wrong end of the strip
Outdated/expired dipstrips
Failure to cap and store dipstrips properly (exposure to air lessens pad reactivity)
Failure to run positive and negative quality controls for new batch of dipstrips
Touching reagent pads with fingers (oils or contaminants interfere with reagents)
Too little or too much urine applied to dipstrip during color development
Overflow of reagent pad chemicals from one pad to another
Loss of chemicals from reagent pad after prolonged exposure in urine
Comparing results from one brand of dipstrips to another
Dipstrip color is compared to color chart from another manufacturer
Failure to match automated reader with the appropriate dipstrip for the analyzer
Poor visual acuity of the personnel reading dipstrip color generation
Poor ambient lighting
AUTOMATED URINARY DIPSTRIP READERS
DIPSTRIP REAGENT PAD COLOR REACTIONS
URINE pH
Urine pH Measurement by Dipstrip
Urine pH Measurement by a pH Meter
Comparisons of Dipstrip and pH Meter Methodology
Expected Urine pH in Health and Disease
Causes of acidic urine
Meat‐based high protein diet
Administration of acidifying agents (e.g. dl‐methionine; NH4Cl)
Metabolic acidosis
Respiratory acidosis
Paradoxical aciduria in metabolic alkalosis with hypokalemia & chloride depletion
Protein catabolic states
Causes of alkaline urine
Plant‐based diet (cereal or vegetable)
Urine allowed to stand open to air at room temperature (loss of CO2)
Postprandial alkaline tide
Urinary tract infection by urease‐positive organism
Contamination of urine sample with bacteria during or after collection
Administration of alkalinizing agents (e.g. NaHCO3, citrate, acetate, lactate)
Thiazide diuretics
Metabolic alkalosis
Respiratory alkalosis (including stress‐induced, especially in cats)
Distal renal tubular acidosis
Paradoxical alkalinuria in states of primary hyperkalemia
URINARY PROTEIN
Methodology
Interferences
Interpretation
Errors in protein measurement on dipstrip analysis
False positive
False negative
Causes of pathologic renal proteinuria
Increased glomerular filtration of protein – increased permeability of glomerulus
Failure of renal tubular reabsorption of protein from tubular fluid
Tubular secretion of protein
Protein leakage from damaged tubular cells
Renal parenchymal inflammation
A combination of the above
URINARY GLUCOSE
Stay updated, free articles. Join our Telegram channel

Full access? Get Clinical Tree
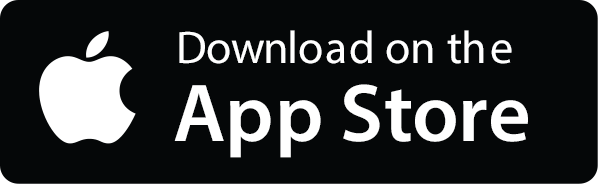
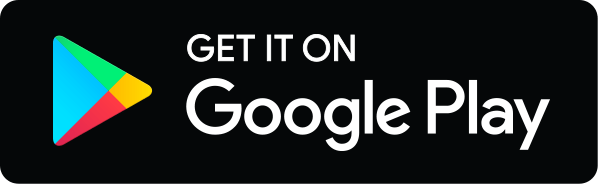