Shubhika Srivastava1, Ira A. Parness2, and Tal Geva3 1 Nemours/Alfred I. duPont Hospital for Children, Wilmington, DE, USA 2 Cohen’s Children’s Medical Center/Northwell Health, New York, NY, USA 3 Boston Children’s Hospital; Harvard Medical School, Boston, MA, USA Tetralogy of Fallot (TOF) is a conotruncal anomaly characterized by: (i) hypoplasia of the subpulmonary conus (infundibulum) resulting in right ventricular outflow tract obstruction; (ii) anterior deviation of the conal (infundibular) septum with respect to the muscular septal crest that leaves a malalignment conoventricular septal defect; (iii) the aortic valve overriding above the crest of the ventricular septum; and (iv) (secondary) right ventricular (RV) hypertrophy. In 1671, Niels Stenson first described the pathology of the syndrome now known as tetralogy of Fallot. Etienne‐Louis Arthur Fallot, in his seminal 1888 paper, debunked the prevailing theory that cardiac cyanosis (“la maladie bleue”) was due to right‐to‐left foramen ovale shunting. Fallot noted that most autopsied patients with cyanotic heart disease had a set of four cardiac malformations that he coined “tetralogy” consisting of pulmonary stenosis, ventricular septal defect (VSD), dextroposition of the aorta, and RV hypertrophy [1–3]. Maude Abbott and W. T. Dawson, in 1924, named this complex malformation “tetralogy of Fallot.” In the nomenclature for TOF recommended by the Congenital Heart Surgeons Society, TOF is classified into three main groups: Tetralogy of Fallot with pulmonary atresia was classified under the category of pulmonary atresia with VSD [4]. However, in this chapter pulmonary atresia with VSD is categorized as the most severe morphologic variant of TOF, termed “TOF with pulmonary atresia.” Tetralogy of Fallot is the most common cyanotic congenital heart defect, with an incidence of 32.6 per 100,000 live births [5,6]. The survival of patients with TOF improved dramatically with the introduction of the Blalock–Taussig shunt in 1944 as the first palliative procedure for cyanotic congenital heart disease [7]. The substantial contributions of Vivien Thomas to the development of this procedure have been belatedly recognized [8]. In the 1980s, surgical management strategies evolved into early complete repair [9], although initial medical and surgical mortality was reported to be as high as 28% [10]. Current surgical practices involve a complete repair with an effort to minimize or avoid RV outflow tract scar and to maintain functional integrity of the pulmonary valve [11]. Successful TOF repair has yielded a large population of adults with repaired TOF, which has necessitated advances in diagnostic techniques and management [12,13]. The pathognomonic conotruncal abnormality of TOF has been attributed to arrest in neural crest cell migration [14]. Studies in chick and mouse embryos have demonstrated the key role of the secondary heart field (SHF) from the anterior mesoderm in cardiac embryogenesis [14–17], including a significant role in conotruncal and RV development in humans [18,19]. Following primary heart tube looping, committed precardiac cells from the SHF migrate to the anterior pole, where they are incorporated into the outflow tracts. Neural crest cells influence and modulate migration of the SHF. Ablation of the SHF or neural crest results in arrest of caudal migration of the committed precardiac cells toward the aortic sac [20–25]. The consequently short outflow tract [24], failure of normal conotruncal rotation, and abnormal coronary artery origin and/or course are typical of TOF [26]. This embryologic theory supports Van Praagh et al.’s hypothesis that the primary morphologic abnormality in TOF is an underdeveloped subpulmonary infundibulum [3]. The secondary precardiac mesoderm expresses NKX2.5 and GATA4 transcription factors [17,20,21]. Gene defects affecting the expression of these transcriptional factors may explain the resultant conotruncal abnormality in TOF. Microdeletion of chromosome 22q11, seen in DiGeorge or velocardiofacial phenotype syndromes, is a common example of a single gene defect causing abnormal neural crest cell migration and resulting in TOF [19]. The prevalence of 22q11 deletions among patients with TOF is much higher in patients with associated pulmonary atresia than in patients with TOF and pulmonary stenosis [27]. Approximately 50 syndromes associated with TOF have been identified [28]. Known chromosomal anomalies, syndromes, or single gene defects are associated with approximately one‐third of the cases with TOF [29,30]. In one study of 64 patients with TOF and associated common AV canal, 88% had a genetic syndrome and extracardiac abnormalities, 67% had Down syndrome, but none had 22q11 deletion [31]. Genetic syndromes in the absence of abnormal pulmonary artery architecture have not been shown to be an independent risk factor for mortality in TOF repair [32], but they may affect perioperative outcomes [33]. Maternal diabetes, exposure to retinoic acid, maternal phenylketonuria, and trimethadione have been reported to be associated with TOF [34,35]. The recurrence rate of nonsyndromic TOF varies between 2.5% and 3% if one sibling is affected, and is 8% if more than one sibling is affected. The reported risk of recurrence is 1.4% from an affected father and between 0.9% and 2.6% for an affected mother. The recurrence rate also depends upon associated syndromes and genetic or environmental factors [36,37]. Tetralogy of Fallot comprises anterior/cephalad malalignment conoventricular septal defect (also called outlet with anterior malalignment VSD), overriding aorta, RV outflow tract obstruction, and RV hypertrophy (Figure 23.1). However, the RV hypertrophy component is the consequence of prolonged systemic level RV pressures rather than being a primary morphologic feature. The principal developmental abnormality in TOF resulting in its components has been debated. There are two theories: Figure 23.1 Tetralogy of Fallot (TOF) with pulmonary stenosis. (a) Diagram showing anterior‐leftward deviation of the infundibular septum (IS) relative to the muscular ventricular septum, narrowed subpulmonary infundibulum (Inf), pulmonary valve (PV) stenosis, right ventricular hypertrophy, aortic override, and right aortic arch (Ao arch). The ventricular septal defect (VSD) is enclosed anteriorly and posteroinferiorly between the limbs of the septal band (SB) and superiorly by the infundibular septum and the junction of the anterior limb of the septal band and right ventricular free wall. This anterior malalignment type of conoventricular septal defect is typical of TOF. (b) Waxed heart specimen showing the right side of the heart of an infant TOF with pulmonary stenosis. AoV, aortic valve; IVC, inferior vena cava; MPA, main pulmonary artery; PA, pulmonary artery; RA, right atrium; RV, right ventricle; TV, tricuspid valve; asterisk denotes the infundibular septum. Source: (a) Courtesy of Dr. Paul Weinberg, Children’s Hospital of Philadelphia. Figure 23.2(a) Normal relationship of the pulmonary valve (PV) in relation to the aortic valve (AoV) as viewed from the apex. (b) Clockwise rotated conotruncus, as viewed from the apex, typical of tetralogy of Fallot with increased rotation and more inferior displacement of the PV which is correlated with severity of TOF and coronary anomalies. In either scheme, the embryonic morphologic abnormality of conal hypoplasia or conal septal malalignment results in failure of ventricular septation (VSD); subpulmonary or valvar pulmonary stenosis; and overriding of the aorta. The anterior malalignment type of conoventricular septal defect is typical of TOF [2,3]. Some authors have classified these defects as paramembranous or perimembranous [44], however most recognize the distinct nature of conal (infundibular or outlet) malalignment in TOF. The defect results from anterior, superior, and leftward deviation of the conal septum, which fails to align with the crest of the muscular septum (Figure 23.4). The malalignment of the conal septum results in a wide communicating space between the ventricles rather than a deficiency, per se, in the “pars membranacea.” The boundaries of the defect (Figure 23.1) are formed in four directions [40–43]: The VSD is thus enclosed anteriorly and posteroinferiorly between the limbs of the septal band, and superiorly by the conal septum and the junction of the anterior limb of septal band and RV free wall (Figure 23.1) [39]. Conal septal morphology is an independent variable in TOF. Irrespective of the degree of subpulmonary conal free wall hypoplasia, the conal septum may be elongated and/or hypertrophied – or, variably hypoplastic or even completely absent. Conal septal absence and subpulmonary infundibular hypoplasia in association with hypertrophy of the septoparietal band results in a variant of TOF with doubly committed subarterial VSD (Figure 23.5, Video 23.1), overriding aorta, and pulmonary annular hypoplasia and stenosis [45]. Figure 23.3 Eisenmenger ventricular septal defect (VSD). (a) Apical view showing the aortic valve (AoV) partially overriding the crest of the ventricular septum and subaortic VSD. (b) Parasternal short‐axis in 2D demonstrating anterior deviation of the conal septum and a widely expanded subpulmonary infundibulum. (c) Parasternal short‐axis with color flow mapping showing left‐to‐right flow across the VSD and absence of obstruction across the right ventricular outflow tract and pulmonary valve (PV). LV, left ventricle; RV, right ventricle. Figure 23.4(a) Subxiphoid right anterior oblique view illustrating the conal septal and right ventricular (RV) free wall relationship. The RV outflow tract obstruction is due to conal septal deviation. Aliased flow at the os infundibulum is demonstrated by color flow Doppler. (b) Parasternal short‐axis view illustrating RV outflow tract obstruction from the conal septal deviation. AoV, aortic valve; PV, pulmonary valve. Figure 23.5 Tetralogy of Fallot with hypoplastic infundibulum (Inf.) and absent conal septum shown from the subxiphoid right anterior oblique view. Note that there is still malalignment but obstruction is primarily from the hypoplastic pulmonary valve (PV) annulus. AoV, aortic valve; RPA, right pulmonary artery; RV, right ventricle. Figure 23.6 Subxiphoid view of a restrictive ventricular septal defect is demonstrated in this patient with tetralogy of Fallot and pulmonary atresia. Accessory tissue is billowing into the left ventricular outflow tract. AoV, aortic valve; LV, left ventricle; RV, right ventricle. The VSD in TOF is typically large but a restrictive defect has been reported in 1.5% of cases (Figure 23.6) [46,47]. The mechanism of obstruction in nearly all the cases results from overlying abnormal or accessory tissue associated with the tricuspid valve. Rarely, myxomatous outpouching of the septal leaflet associated with the Ebstein anomaly in TOF can result in a restrictive VSD [47,48]. VSD restriction due to absolutely small VSD dimensions (caused by posterior septal band deviation and ventricular septal hypertrophy) has been described (Figure 23.7) [47]. TOF may be seen in association with common AV canal defects, as discussed in detail later in this chapter (Figure 23.8) [39,49–51]. Clockwise rotation of the aortic root (as viewed from the cardiac apex), and the resultant rotated origins of the coronary arteries, is common in TOF (Videos 23.2 and 23.3). Anomalies in the branching pattern of the coronary arteries are reported in approximately 5% of all cases (Figure 23.9) [51,52]. The most common anomaly, occurring in 3% of patients, is origin of the left anterior descending coronary artery from the right coronary artery (RCA) (Videos 23.4 and 23.5); this is followed by dual left anterior descending coronary artery in 1.8%, single RCA in 0.3%, single left coronary artery (LCA) in 0.2%, and coronary‐to‐pulmonary artery fistula in 0.2%. In an angiographic study, anomalies of coronary artery branching pattern were more prevalent in patients with the most prominent aortopulmonary rotation [53]. The extraordinarily rare “isolated infundibulo‐arterial inversion” in TOF is associated with the RCA crossing anteriorly across the subpulmonary infundibulum. Anomalous origin of the LCA from the pulmonary artery has been reported in patients with TOF [54], but echocardiographic recognition of its hallmark retrograde flow jet into the main pulmonary artery may be masked by the marked color flow turbulence related to the pulmonary stenosis. This rare coronary variant should be suspected whenever TOF is accompanied by any degree of left ventricular (LV) dysfunction and/or mitral regurgitation along with echo brightness of the LV endocardium. Origin of the LCA from the noncoronary cusp can also occur; its clinical implication thus far remains unclear. Coronary artery‐to‐pulmonary artery fistulae may rarely serve as a source of pulmonary blood flow in TOF with pulmonary valvar atresia or severe stenosis (Figure 23.10, Video 23.6) [55]. Isolated coronary ostial stenosis or obstruction has also been reported in the absence of any other coronary anomaly [56]. Acquired coronary cameral fistulae may be observed following surgery, particularly with aggressive RV muscle bundle resection [57]. Some authors have categorized extreme dextroposition, or aortic overriding with more than 50% of the aortic annulus over the RV, as double‐outlet right ventricle (DORV) [39,58]. Nonetheless, according to Van Praagh et al., the maintenance of aortic‐to‐mitral valve fibrous continuity or normally related great arteries is pathognomonic for TOF irrespective of the degree of aortic override or dextroposition [2,39]. Specifically, TOF is a specific type of conotruncal anomaly in which aortic–mitral fibrous continuity is a prerequisite regardless of the degree of aortic override. In contrast, DORV is a specific type of ventriculoarterial alignment defined as exclusive or near‐exclusive origin of the great arteries from the RV regardless of the type of conus (see Chapter 26). This approach recognizes a wide spectrum of aortic overriding within TOF without imposing an arbitrary dichotomous cut‐off between TOF and DORV depending on the percentage of aortic override. Aortic–mitral fibrous continuity is also important in the prediction of postoperative LV outflow tract obstruction: progressive subaortic obstruction following the repair of typical TOF is rare [59]. However, progressive muscular hypertrophy causing subaortic obstruction may develop in cases of “TOF‐like” DORV with retention of subaortic conus (see Chapter 26). Figure 23.7 Tetralogy of Fallot with a small, restrictive ventricular septal defect (VSD) secondary to hypoplasia of the ventriculoinfundibular fold. (a) Subxiphoid short‐axis view demonstrating the small dimensions of the defect in the superior–inferior plane. (b) Parasternal long‐axis view demonstrating the small dimension in the base‐to‐apex plane. (c) Restrictive left‐to‐right shunting at the VSD demonstrated by continuous‐wave Doppler interrogation. AoV, aortic valve; LV, left ventricle; RV, right ventricle; TV, tricuspid valve. Figure 23.8(a) Subxiphoid short‐axis view in systole showing tetralogy of Fallot with complete common atrioventricular canal defect, Rastelli type C, with anterior deviation of the conal septum (CS) and flow acceleration in the right ventricular outflow tract (RVOT). (b) Subxiphoid short‐axis view in diastole demonstrating the common atrioventricular valve (AVV). Figure 23.9 Diagram of the coronary artery patterns in tetralogy of Fallot. br., branch; Cx, circumflex artery; LAD, left anterior descending coronary artery; LCA, left coronary artery; RCA, right coronary artery. Figure 23.10 Parasternal short‐axis image with color Doppler of a large left coronary‐to‐left pulmonary artery fistula in a patient with tetralogy of Fallot. LCA, left coronary artery; MPA, main pulmonary artery. Double‐chambered right ventricle (DCRV) is an anomaly characterized by obstruction within the right ventricle with or without an associated VSD (see Chapter 17). It can also present as a progressive lesion following corrective surgery for TOF [58]. DCRV is often misdiagnosed as TOF because both lesions have a VSD and subpulmonary stenosis [60]. However, in DCRV, the primary morphologic abnormality resulting in subpulmonary stenosis is either marked hypertrophy of the septoparietal trabeculations or moderator band, and/or abnormal displacement of the moderator band resulting in narrowing of the proximal infundibular ostium. Unlike TOF, DCRV is typically associated with normal size and morphology of the pulmonary valve and main and branch pulmonary arteries. Additionally, the VSD seen in DCRV is typically membranous or central perimembranous rather than the malalignment‐type defect typical of TOF. However, there are overlap syndromes that share features of DCRV and TOF, such as those with an anterior malalignment type of VSD and aortic overriding but with a morphologically normal pulmonary valve and a well‐developed infundibulum; in these cases, the subpulmonary obstruction is limited to the proximal os infundibulum (Figure 23.11, Video 23.7). Tetralogy of Fallot with pulmonary atresia is a severe form of TOF. In addition to valvar atresia (short‐segment atresia) or valve and main pulmonary artery absence (long‐segment atresia), this condition is characterized by marked variability in the degree of pulmonary artery hypoplasia or its absence and/or the presence of abnormal pulmonary arterial supply. The intracardiac anatomy, like other forms of TOF, typically consists of an enlarged and overriding aorta and an anterior malalignment VSD. In addition, there is marked infundibular hypoplasia due to extreme leftward deviation of the conal septum, which may even merge with the septal parietal band and RV free wall producing subvalvar atresia. This defect was initially misclassified as truncus arteriosus type IV in the classification of Collett and Edwards [61]. However, Van Praagh and Van Praagh correctly recognized this entity as being at the most severe end of the pulmonary obstruction spectrum in TOF, i.e., TOF with pulmonary atresia [62]. This entity was subsequently given the moniker of “pseudotruncus” by Bharati et al. [63]. The Congenital Heart Surgeons’ Society Nomenclature Committee classified TOF with pulmonary atresia under the umbrella of “pulmonary atresia with VSD” [64]. Macartney et al. coined the now widely adopted term major aortopulmonary collateral artery(s) or MAPCA(s), to describe the abnormal pulmonary arterial supply often seen in TOF with pulmonary atresia [65]. Figure 23.11 Double‐chambered right ventricle. (a) Parasternal short‐axis image of tetralogy of Fallot with double‐chambered right ventricular (RV) variant. Note the deviated conal septum and prominent RV free wall muscle bundle resulting in narrowing of the proximal infundibular os but absence of infundibular hypoplasia and a normal pulmonary valve or distal infundibular os. (b) Subxiphoid right anterior oblique view in color compare mode demonstrating the deviated conal septum (CS) and hypertrophied septal band (SB) resulting in the double‐chambered RV variant. Again note a well‐expanded infundibulum and distal os infundibulum. AoV, aortic valve; PV, pulmonary valve. TOF with pulmonary atresia can be further classified into three broad categories [64]: Figure 23.12 Tetralogy of Fallot with pulmonary atresia. High left parasternal axial oblique color compare image showing “long‐segment” (main) pulmonary artery atresia and diminutive, confluent mediastinal pulmonary arteries with retrograde flow in the right pulmonary artery on color flow mapping. The asterisk (*) highlights the pericardial fluid in between the appendage and ascending aorta that can sometimes simulate the appearance of a main pulmonary artery. LA, left atrium; LAA, left atrial appendage. Generally, the degree of branch pulmonary artery hypoplasia is highly associated with the extent of MAPCAs as well as the likely absence of a ductus arteriosus [66–68]. The angiographically determined Nakata index of the native branch pulmonary artery cross‐sectional area predicts good outcomes of complete repair of TOF with pulmonary artery hypoplasia if the index is greater than 100 mm2/body surface area (BSA) [69]. A number of cardiovascular anomalies associated with TOF with pulmonary atresia have been reported, including restrictive VSD [70,71], coronary artery‐to‐pulmonary artery fistula as a source of pulmonary blood flow [50], and aortopulmonary window [70]. TOF with pulmonary atresia is associated with increased incidences of retroaortic innominate vein [63,72,73] and right aortic arch (particularly in those with 22q11 deletion). A common AV canal (CAVC) in association with TOF is an uncommon variant, seen in 1.7% of all cases of TOF and in 6.2% of cases with complete CAVC defects [49,50]. A very high incidence (87.5%) of genetic (typically Down syndrome) and extracardiac abnormalities are seen in this morphologic variant [74]. Rastelli type C morphology of the common AV valve has been reported in 85% of cases of TOF with CAVC (Video 23.8) [49]. There can be concomitant absence of the conal septum in the setting of otherwise typical morphology for TOF with CAVC. A small or absent primum atrial septal defect component can also occur in this morphologic variant, which can increase the challenge of surgical correction of this lesion [50,51,74,75]. TOF may also exist in conjunction with a cleft mitral valve without a primum atrial septal defect [76]. The prevalence of TOF with an absent pulmonary valve is reported as being between 3% and 6% of TOF cases [77,78]. This anatomic variant is characterized by typical anterior malalignment VSD and aortic overriding but is differentiated by generally modest hypoplasia of the pulmonary valve annulus with rudimentary, foreshortened and dysplastic leaflets, not only causing pulmonary stenosis but also allowing significant regurgitation. Typically, the main pulmonary artery and/or one or both branch pulmonary arteries are aneurysmally enlarged (Figure 23.13, Video 23.9) [79,80]. This lesion is usually associated with absent ductus arteriosus [79,81]. Marked dilation of the pulmonary arteries is associated with variable degrees of bronchial compression and intraparenchymal pulmonary arteriopathy and may cause severe neonatal respiratory distress. In the fetus, severe forms may lead to hydrops fetalis or fetal demise. In rare cases, a ductus arteriosus may be present, usually with discontinuous branch pulmonary arteries or in the setting of a vascular ring. In the setting of discontinuous pulmonary artery the branch pulmonary artery supplied by the ductus is not usually aneurysmal. In this exceedingly rare variant of TOF, the dysplastic pulmonary valve leaflets are “inverted” such that the valve remains closed during systole (functional “atresia”), allowing no antegrade pulmonary valve flow, whereas the valve opens during diastole to allow regurgitant flow from the main pulmonary artery into the right ventricle (Figure 23.14, Video 23.10). In this unique situation, other sources of pulmonary blood flow must be present (such as ductus arteriosus or MAPCAs). Figure 23.13 Color flow Doppler mapping of the right ventricular outflow tract (RVOT) in an infant with tetralogy of Fallot and absent pulmonary valve syndrome. The left panel shows a systolic frame with antegrade flow from the RVOT to the main pulmonary artery (MPA). The right panel shows a diastolic frame with a wide jet of retrograde flow from the MPA into the RVOT. Figure 23.14 Tetralogy of Fallot with “inverted” pulmonary valve. (a) Parasternal short‐axis view of the right ventricular (RV) outflow tract showing no antegrade systolic flow from the RV outflow tract through the pulmonary valve (PV). Note right‐to‐left flow through the ventricular septal defect (VSD) into the aortic root (Ao). (b) Diastolic frame showing retrograde flow through the PV into the RV outflow tract. LPA, left pulmonary artery; MPA, main pulmonary artery; RPA, right pulmonary artery. Right aortic arch is seen in approximately 25% of patients with TOF [82], and is often associated with an aberrant left subclavian artery [73]. Double aortic arch, persistence of the fifth aortic arch, or other morphologic variations of vascular rings may also be associated with TOF [65,83,84]. Right aortic arch with isolation of the subclavian artery or with aberrant origin of the left subclavian artery from the ascending aorta have been reported [85,86]. The isolated left subclavian artery can be supplied by the left vertebral artery (in which case the direction of blood flow is reversed) and may cause a subclavian steal phenomenon. Alternatively, the isolated left subclavian artery may be connected via a patent ductus arteriosus to the main pulmonary artery. In the latter case, the left vertebral artery supplies the main pulmonary artery resulting in a congenital pulmonary artery steal [87]. Aortic origin of branch pulmonary artery [88], unilateral absence of branch pulmonary artery, crossed pulmonary arteries [89,90], aortopulmonary window [91], and pulmonary artery slings [92] have also been described in association with TOF. Crossed pulmonary arteries may be a marker for 22q11 deletion[93]. Isolated reports of annuloleaflet (also known as supramitral) mitral ring, LV outflow tract obstruction secondary to adherent anterior mitral valve leaflet, and cleft mitral valve have been described in patients with TOF [3,76,93]. Other associated left heart lesions include discrete subvalvar aortic stenosis, aortic valve abnormalities (bicommissural, stenosis, or regurgitation) [91,94,95], coarctation of the aorta [95], LV diverticulum [96], LV hypoplasia, and cor triatriatum [97]. In TOF, systemic and pulmonary venous anomalies may be seen independently or in association with heterotaxy syndrome [98–101]. A retroaortic position of the innominate vein is more commonly seen in TOF with pulmonary atresia (Video 23.11) [72]. Left superior vena cava to the coronary sinus (or rarely, to the left atrium) has been described in up to 11% of cases [101,102]. Left superior vena cava to left atrium without a connecting left innominate vein, if undiagnosed, can result in persistent hypoxemia following TOF repair. Persistence of the levoatrial cardinal vein has also been reported [100]. A partially or totally anomalous pulmonary venous connection may be seen in association with TOF [103], rarely as part of the scimitar syndrome [104]. Atrial septal defects, typically secundum type, are seen in 86% of cases [102]. Rarely, sinus venosus defects or coronary sinus septal defects occur in TOF [99,105]. In TOF associated with heterotaxy syndrome, small left‐sided structures including mitral stenosis and left ventricular hypoplasia are often seen. Isolated infundibuloarterial inversion in the setting of atrial situs solitus, D‐looped ventricles, and inversus normally related great arteries, or {S,D,I}, has been reported in TOF [106–109]. In the right side of the heart, Ebstein anomaly and double‐orifice tricuspid valve have been reported [48,99]. The association of TOF with ectopia cordis (often in combination with other midline defects and ventricular diverticulum) is known as “pentalogy of Cantrell” [100]. There is no known impact on survival secondary to physiologic alterations from TOF in utero. Of note, the RV outflow tract obstruction can progress throughout the pregnancy, even becoming pulmonary atresia, and the branch pulmonary arteries can become smaller. In TOF with common AV canal, the degree of regurgitation may influence the development of hydrops as with any other lesion in utero, with significant AV valve regurgitation. In TOF with absent pulmonary valve, outcomes are dependent upon the degree of LV dysfunction, and immediate postnatal outcomes on the degree of respiratory compromise. The physiology in TOF is determined by the combination of the VSD and the degree of pulmonary stenosis. The degree of cyanosis is primarily determined by the degree of RV outflow tract obstruction and right‐to‐left shunting at the VSD. The clinical presentation varies from that of no cyanosis “pink TOF” to that of significant cyanosis that may require patency of the ductus arteriosus to maintain adequate pulmonary blood flow. Hypercyanotic spells (commonly known as “tet spells”) result from right‐to‐left shunting at the level of the VSD, induced by dynamic obliteration of the RV outflow tract or from acute shifts in pulmonary and systemic vascular resistance. It is essential to determine the need for ductal patency in TOF with severe RV outflow tract obstruction/pulmonary atresia. Prostaglandin infusion may also be needed in TOF with a balanced circulation in the setting of discontinuous pulmonary arteries – when a branch pulmonary artery is supplied by a ductus arteriosus. In the setting of severe RV outflow tract obstruction and absent ductus arteriosus, medical management in maintaining adequate pulmonary blood flow may fail, necessitating an urgent surgical or catheter intervention in the immediate neonatal period. Patients are usually asymptomatic following TOF repair. The need for future interventions will be dependent upon residual lesions, degree of RV outflow tract and branch pulmonary artery obstruction, and pulmonary regurgitation. Symptoms of right‐sided heart failure can develop with RV dysfunction and/or severe tricuspid regurgitation. Some patients may be symptomatic from arrhythmias such as atrial or ventricular tachycardia. Detailed echocardiographic assessment has made diagnostic angiography nearly obsolete in the routine preoperative assessment of TOF. However, angiography, cardiac magnetic resonance (CMR), and computed tomography (CT) serve an important complementary role in the assessment of rare aortic arch anomalies as well as in the setting of pulmonary atresia, MAPCAs and hypoplastic branch pulmonary arteries, and in absent pulmonary valve syndrome. CMR and CT provide unique information about pulmonary vessels and airway architecture, both of which are important for catheter intervention and surgical planning. Organized, systematic, and detailed imaging starting with the subxiphoid long‐axis window is the preferred approach for these authors for accurate diagnosis of congenital heart disease (see Chapter 4). In TOF with moderate stenosis, sedation imposes an additional risk for a hypercyanotic spell due to the combination of intravascular volume depletion (from being kept nil per os for sedation) and systemic vasodilation. Hence, whenever possible, the details of branch pulmonary artery anatomy, arch morphology, and coronary morphology should be defined during a newborn examination. Neonates will often sleep soundly after feeding, allowing a comprehensive and detailed examination without sedation. Color flow mapping and spectral Doppler are crucial in refining the diagnosis, screening for subtle structural abnormalities (e.g., coronary, arch, and branch pulmonary artery) and, of course, in assessing the hemodynamics, as described in Chapter 6. The initial subxiphoid long‐axis sweep demonstrates visceral and atrial situs, systemic venous connections, coronary sinus, and atrial septal morphology (Video 23.12) [111]. The descending aorta in its cross‐section may be visualized to the left of the spine, and through the course of the sweep it can be seen crossing over to the right of the thoracic spine. The VSD with overriding of the aorta may be seen as well as subpulmonary infundibular hypoplasia and stenosis. Branch pulmonary arteries may also be identified from subxiphoid long‐axis imaging as the right pulmonary artery traverses from left to right above the atrial mass; the left pulmonary artery and ductus are often better imaged with color Doppler flow mapping (Video 23.13). The subxiphoid short‐axis view defines the atrial septum, the entrance of the right upper pulmonary vein into the left atrium, VSD number, size, and location, RV hypertrophy, and muscle bundles within the right ventricle that could result in additional intracavitary obstruction, as in DCRV. The cleft mitral valve and CAVC are also well imaged in this plane. A modified right anterior oblique subxiphoid view is of exceptional benefit in cases of TOF because it highlights the subpulmonary infundibulum, inclusive of both the size of the conal septum and its displacement (see Figure 23.11b, Video 23.14) [112]. This view is also useful for assessing additional muscle bundles contributing to RV outflow tract obstruction in the DCRV variant. Apical views demonstrate the AV valves, VSD, and overriding aortic valve (Video 23.15). This view may also help identify other associated anomalies, such as left‐sided obstructive lesions. Additionally, the mechanism of atypical restriction of the VSD may be visualized (Video 23.16). Parasternal imaging may be limited if the thymus, which usually provides a reliably good acoustic window, is absent. The parasternal long‐axis view demonstrates the overriding aorta, aortic‐to‐mitral valve fibrous continuity (distinguishing TOF from its related type of DORV) (Figure 23.15, Video 23.17), VSD morphology, and pulmonary valve morphology as the transducer sweeps leftward and anteriorly (Video 23.18). Figure 23.15 Parasternal long‐axis view. (a) Image of the large ventricular septal defect (arrow) with the aortic valve overriding the ventricular septum. Note the fibrous continuity between the aortic and mitral valve (arrowhead). (b) Tilting the transducer toward the left shoulder demonstrates the pulmonary valve (PV), main pulmonary artery (MPA), and proximal right pulmonary artery (RPA). Measurements of the pulmonary valve annulus and MPA are shown. AAo, ascending aorta; DAo, descending aorta; LA, left atrium; LV, left ventricle; RV, right ventricle. The parasternal short‐axis view further defines VSD morphology, subpulmonary obstruction, infundibular hypertrophy, and deviation of the conal septum (Video 23.19). An absent conal septum and extension of the VSD to the hypoplastic pulmonary valve annulus defines a doubly committed subarterial defect in TOF [45]. Imaging of the long axis of a ductus arteriosus is best performed from a high parasternal window in a sagittal plane or from the suprasternal notch long‐axis view. At times, differentiating between a ductus arteriosus and aortopulmonary collateral is difficult because both may arise from the same location in the proximal descending thoracic aorta. However, the termination of the vessel at the proximal branch pulmonary artery origin is consistent with a ductus arteriosus, whereas aortopulmonary collaterals usually insert more distally into the branch pulmonary arteries at or distal to the hilum. Assessment of the ductus arteriosus has become more important in recent years because of ductal stenting as a potential initial palliation when pulmonary blood flow is inadequate. Orthogonal imaging in a coronal plane from a high left parasternal view will demonstrate the site of ductal insertion into the proximal branch pulmonary artery to evaluate the possibility for development of peripheral pulmonary artery stenosis or discontinuity consequent to ductal closure. Crossed pulmonary arteries are diagnosed when the right pulmonary artery originates inferiorly from the left aspect of the main pulmonary artery and the left pulmonary artery originates more superiorly from the right aspect of the main pulmonary artery (see Chapter 19). The high left and right parasternal views help to evaluate the main and branch pulmonary arteries (Video 23.20). It is important to align the beam perpendicular to the pulmonary artery being interrogated by altering the window of interrogation for each pulmonary artery. High (subclavicular) left sternal border windows are usually best for the main and left pulmonary arteries. High (subclavicular) right sternal border windows are usually best for the right pulmonary artery. Larger branch pulmonary arteries have a lesser likelihood of concomitant presence of MAPCAs. A branch pulmonary artery diameter z‐score of less than −2.5 had a sensitivity of 88% and specificity of 100% for the presence of one or more MAPCAs in one study [66]. Arch sidedness and branching pattern, retroaortic innominate vein (Video 23.11), partially anomalous pulmonary venous connections to a systemic vein, double aortic arch, and vascular rings can be evaluated from this view supplemented by high parasternal views. Left superior vena cava to coronary sinus or directly to left atrium with an unroofed coronary sinus are best seen from this view or from a left parasternal window in the sagittal plane. Color flow mapping of the atrial septum usually demonstrates left‐to‐right shunting even in the presence of severe pulmonary stenosis. Bidirectional or right‐to‐left shunting may be present in newborns or in those with restrictive VSDs. Flow across the large VSD is usually nonrestrictive and bidirectional; only rarely is it restrictive. The degree of RV outflow tract obstruction determines the direction of flow across the VSD – less pulmonary stenosis allows predominantly left‐to‐right shunting, the clinical scenario of “pink” TOF. More severe pulmonary stenosis results in predominantly right‐to‐left shunting and cyanosis. In the typical setting of a nonrestrictive VSD, RV peak pressures cannot exceed systemic levels. Suprasystemic RV pressures in TOF can only occur in the setting of a restrictive VSD with pulmonary atresia or severe stenosis (see Figures 23.6 and 23.7) [110]. Also, in the setting of a nonrestrictive malalignment VSD, additional muscular VSDs may be difficult to identify. Therefore, it is essential to interrogate the muscular septum by color flow mapping with a low Nyquist limit to identify low‐velocity shunting across these small defects (Video 23.21). In the rare preoperative TOF patient with pulmonary hypertension, as seen in Eisenmenger syndrome, the degree of right‐to‐left shunting across the VSD is affected by the presence of elevated pulmonary vascular resistance. Identification of a nonrestrictive source of pulmonary blood flow (such as an aortopulmonary window [113] or large ductus arteriosus [114]) is essential, as is the assessment for reversibility of pulmonary hypertension. Pulmonary venous obstruction has also been reported in association with TOF [115]. The level of obstruction can be determined by imaging and by sequential pulsed Doppler interrogation, starting from within the RV cavity to the main pulmonary artery. In severe stenosis, antegrade flow may be demonstrated using a lower frequency transducer that achieves a higher Nyquist limit; this allows less “aliasing” of the color flow map of the high‐velocity flow across the stenotic outflow tract. Continuous‐wave Doppler interrogation is essential to estimate the total maximal instantaneous gradient, but accurate separation of the infundibular and valvar contributions to the total gradient is difficult with any Doppler interrogation technology (Figure 23.16). A double envelope is often noted by continuous‐wave interrogation; the late‐cresting systolic velocity peak represents the proximal dynamic portion of the RV outflow tract gradient. The need for a transannular patch repair may be predicted by the size of the pulmonary valve annulus and morphology. The decision for sparing the pulmonary valve based on annulus size and associated supra‐annular narrowing is surgeon and institution dependent. The following representative experiences have been reported: (i) pulmonary valve annulus z‐score ≥ –4, tricommissural valve, and RV/LV pressure ratio <0.7 associated with a higher likelihood of pulmonary valve‐sparing procedures [2,116]; (ii) no association of pulmonary valve annulus z‐score with ability to preserve the pulmonary valve [117]; and (iii) pulmonary valve annulus z‐score < –1.3 more likely to have postoperative pulmonary stenosis ≥30 mmHg [118]. In TOF with absent pulmonary valve syndrome, the severely dilated branch pulmonary arteries are readily apparent on subxiphoid imaging (Videos 23.9 and 23.22), and pulmonary regurgitation is easily demonstrated by color flow mapping in many views (Videos 23.23 and 23.24). It is typically associated with diastolic flow reversal in the branch pulmonary arteries [119]. Figure 23.16(a) Right ventricular (RV) outflow tract gradient by continuous‐wave Doppler demonstrating a double envelope. The outer envelope with a parabolic shape reflects the total gradient. The envelope with a delayed systolic peak and the lower gradient is due to proximal RV outflow tract obstruction. (b) Pulsed‐wave Doppler in the RV outflow tract demonstrating obstruction due to dynamic subpulmonary narrowing with a delayed maximal instantaneous gradient. In patients with TOF and long‐segment pulmonary atresia, the left atrial appendage can mimic the central main pulmonary artery segment (Video 23.25); this can be further delineated by careful imaging and by demonstration of low velocity to‐and‐fro flow by color and pulsed‐wave Doppler. Because of its position under the aortic arch, the retroaortic innominate vein may mimic central confluent branch pulmonary arteries in pulmonary atresia. Doppler interrogation demonstrates the connection of the retroaortic innominate vein to the internal jugular vein or superior vena cava and demonstrates the typical multiphasic venous flow pattern of an innominate vein. Color flow mapping from the apical window is used to assess the AV valve regurgitation that is usually seen in patients with associated malformations of the AV valves (e.g., Ebstein anomaly, double‐orifice tricuspid and mitral valves, common AV valve, and cleft mitral valve). In the setting of significant mitral regurgitation without morphologic abnormalities and/or LV hypokinesis, associated anomalous origin of the LCA from the pulmonary artery must be excluded. Standard measurements in parasternal views will identify root and ascending aortic dilation, commonly seen in TOF, and allow serial follow‐up of aortic size. Aortic regurgitation is uncommon preoperatively; it is most often acquired postoperatively or secondary to endocarditis. The operator should employ the highest frequency transducers to achieve high‐resolution coronary artery imaging. The Nyquist limit should be lowered on Doppler interrogation to accentuate low‐velocity flow. The origin of the coronary arteries can be demonstrated from the parasternal short‐axis image. Clockwise rotation of the origins of the coronary arteries, a common finding in TOF, may be appreciated in this view. The LCA and its bifurcation are elongated by clockwise rotation of the transducer from the short‐axis into a transverse plane (Videos 23.3–23.5). Evaluation for a left anterior descending coronary artery origin from the RCA crossing the RV outflow tract requires a careful sweep from the standard reference view in the parasternal long axis, angling toward the left shoulder. Additional views that may be helpful include a high left parasternal cut in a transverse plane or a modified apical four‐chamber view angled anteriorly. Prominent crossing conal branches or dual left anterior descending coronary arteries may be diagnosed in these sweeps, or occasionally from the most coronal extent of subxiphoid long‐axis sweeps. Rare single RCA or LCA and anomalous origin of the LCA from the pulmonary artery may also be diagnosed. However, the echocardiographer must recognize that the turbulent pulmonary artery flow jets that characterize TOF may obscure the retrograde coronary flow emptying into the pulmonary artery. Coronary–cameral fistulae may also be detected by color flow mapping but coronary–pulmonary artery fistulae may be obscured by aliased pulmonary artery flow patterns due to the pulmonary stenosis. Intraoperative echocardiographic assessment of TOF repair is almost always accomplished by using transesophageal echocardiography (TEE) or, in situations where esophageal access is not possible, utilizing an epicardial approach. The goal of the intraoperative TEE evaluation should be aimed at identification of hemodynamically significant lesions that can affect immediate postoperative management as well as long‐term outcomes. Knowing the details of the surgical repair is essential to a successful intraoperative echocardiographic evaluation, and good communication between the surgeon and echocardiographer is paramount. Conventional echocardiography and TEE are important in the evaluation of the postoperative patient in the intensive care unit, especially to assess acute changes in clinical status. In addition to the TEE evaluation described next, conventional echocardiography enables assessment of diaphragmatic motion and pleural or pericardial effusions. Conventional windows are often superior to TEE for assessment of the aortic arch and branch pulmonary arteries.
CHAPTER 23
Tetralogy of Fallot
Definition
Incidence
Embrylogy, genetics, and environmental factors
Embryology
Genetics
Environmental factors
Recurrence risk
Morphology
The conotruncus in TOF
Ventricular septal defect morphology
Coronary arteries
Variants and associated lesions
TOF and double‐outlet right ventricle
TOF and double‐chambered right ventricle
TOF with pulmonary atresia or extreme pulmonary hypoplasia
TOF with common atrioventricular canal defect
TOF with absent pulmonary valve syndrome
TOF with “inverted” pulmonary valve
Aortic arch anomalies
Aortopulmonary defects and variations in pulmonary artery origin and bifurcation
Left‐sided lesions associated with TOF
Systemic and pulmonary venous anomalies and atrial septal defects
Other rarely associated malformations
Pathophysiology
Prenatal
Neonatal
Repaired TOF
Imaging
Preoperative assessment
Organization of preoperative anatomic assessment
Subxiphoid views
Apical views
Parasternal views
Ductal views
High left parasternal view
Suprasternal notch views
Preoperative assessment by structures
Atrial septum
Ventricular septal defect
Right ventricular outflow tract and pulmonary arteries
Atrioventricular valves
Aortic root dilation and regurgitation
Coronary arteries
Intraoperative and postoperative assessment after TOF repair
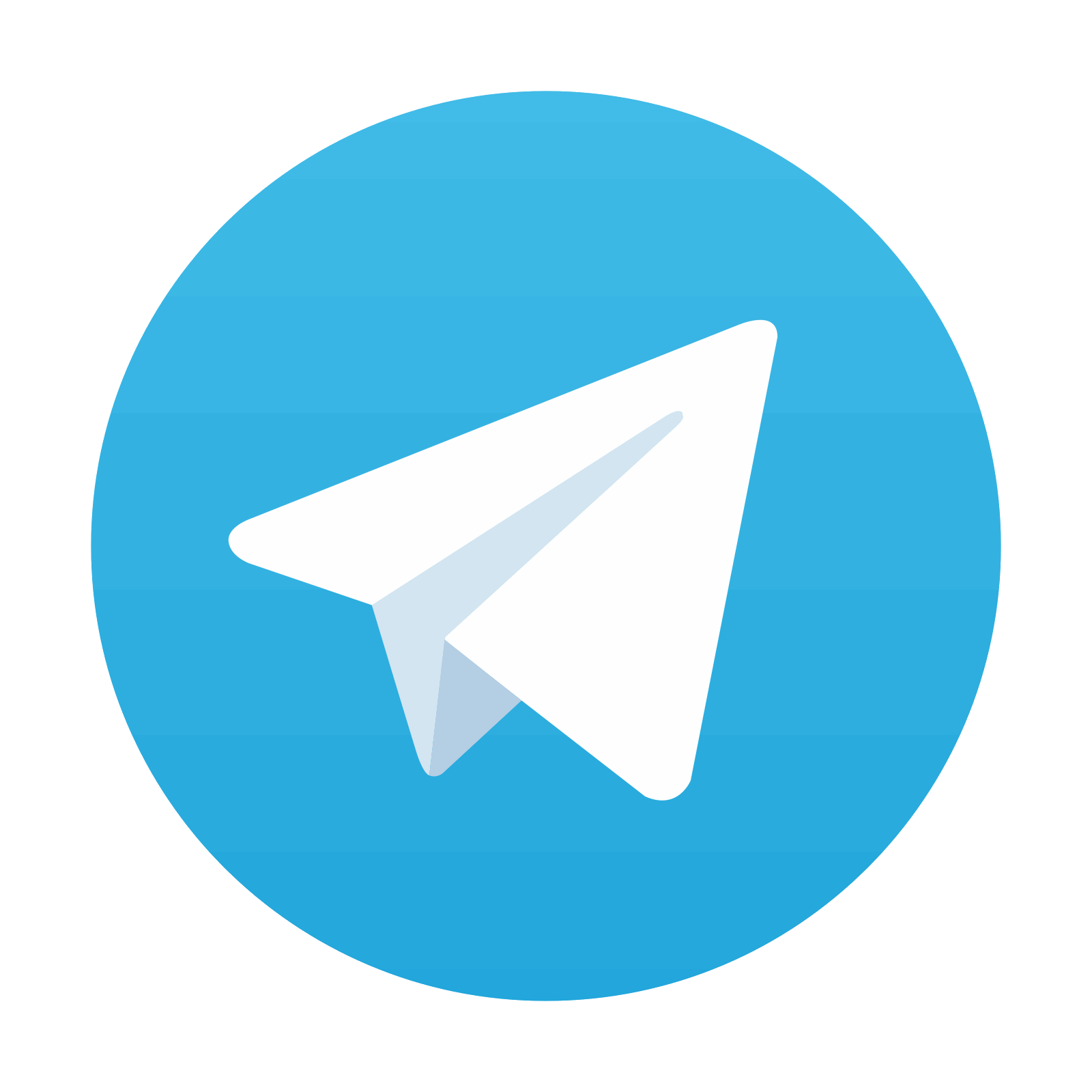
Stay updated, free articles. Join our Telegram channel

Full access? Get Clinical Tree
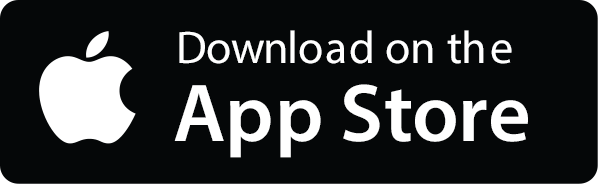
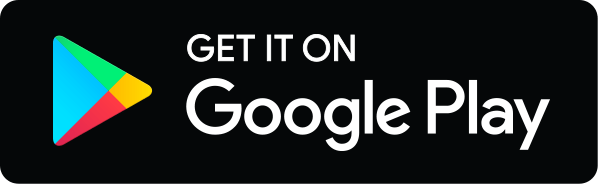