Elizabeth Caris and Bhawna Arya University of Washington School of Medicine; Seattle Children’s Hospital, Seattle, WA, USA The ductus arteriosus (DA) is a normal vascular structure in the human fetus. It normally connects the main pulmonary artery (MPA) to the descending aorta, allowing most of the blood from the right ventricle (RV) to bypass the fetus’s fluid‐filled lungs and high‐resistance pulmonary vascular bed. In a left aortic arch, the DA inserts into the proximal descending aorta at the junction with the aortic isthmus, distal to the origin of the left subclavian artery. In rare cases, there can be a right patent ductus arteriosus (PDA) in association with a left aortic arch in which case the right PDA connects the right pulmonary artery to the right subclavian artery. In a right aortic arch, there are three types of DA insertion from the MPA to the aorta: (i) directly into the right descending aorta (right DA); (ii) directly into the proximal left subclavian artery (left DA); or (iii) both (bilateral DA). Normally, the DA closes during the first hours to days of life. Persistence of ductal blood flow is termed PDA. The incidence of persistent PDA without additional cardiac anomalies varies greatly depending on the postnatal age of the patients and their degree of prematurity. The overall incidence of PDA is inversely related to increasing gestational age and birth weight [1]. PDA is common in preterm infants and is considered a functional abnormality as opposed to a structural congenital cardiac anomaly. In full‐term infants, the DA may remain patent for several days after birth. Doppler echocardiogram studies in healthy newborn infants have demonstrated closure of the DA in nearly all subjects by 4–7 days after birth [2,3]. Based on a review of 40 published articles over several decades, it has been estimated that the median incidence of PDA in term infants is 56.7 per 100,000 live births [4]. If children with an incidental finding of a PDA are included in this estimate, the incidence may be has high as 1 in 500 [5]. The etiology of isolated PDA in full‐term infants older than 1 week of age is not well understood. PDA can be a result of environmental exposure before birth, isolated genetic mutations, or genetic syndromes. Congenital rubella is associated with a high incidence of PDA [6–8]. Patients at high altitude are at increased risk for PDA, likely due to chronic hypobaric hypoxia, as oxygen saturation and arterial oxygen pressure are lower [9]. Recent investigations have identified the genes that affect the development of the fourth and sixth pharyngeal arches, and noted their association with cardiovascular defects similar to those observed in chromosome 22q11 deletions [10]. Char syndrome is a familial form of PDA with characteristic facial dysmorphisms and hand anomalies [11]. The normal anatomy and congenital malformations of the DA and branch pulmonary arteries are determined by the development of the embryonic sixth aortic arches. The human embryo has two dorsal aortas, which communicate with the aortic sac through several branchial arch arteries. During normal development, there is systematic appearance and regression of the six pairs of primitive aortic arches. Both the ductus arteriosus and the branch pulmonary arteries are derived from the fourth and sixth arches. The left fourth arch becomes the distal left aortic arch (between the left common carotid and left subclavian artery). The right fourth arch persists as the innominate artery and proximal right subclavian artery. The left dorsal aorta persists as the segment that extends from the left subclavian artery to the aortic isthmus, or site of entrance of the DA. The right dorsal aorta involutes. The proximal portions of the right and left sixth arches form the proximal portions of the right and left pulmonary arteries, respectively. The distal left sixth arch forms the DA and the distal right sixth arch regresses [12]. The pathophysiology of PDA depends on several factors, including patient age, associated congenital heart disease, and hemodynamic and anatomic factors. In preterm infants, the presence of a PDA is common and associated with increased morbidity. It is considered a functional abnormality in this population. In full‐term infants and children, PDA likely results from a significant structural abnormality. The flow pattern and hemodynamic consequences of an isolated PDA depend on resistance to flow through the duct, as well as the pulmonary and systemic vascular resistances. The PDA resistance is determined by the smallest cross‐sectional area, length, and its configuration. In the setting of normal pulmonary vascular resistance (PVR), an isolated PDA demonstrates flow from the aorta to the pulmonary artery (left‐to‐right), resulting in increased pulmonary blood flow and left heart volume overload. If persistent, the left atrium and ventricle will dilate and mitral regurgitation may develop over the long term. Left ventricular systolic function is usually preserved. A large PDA with significant left‐to‐right flow leads to pulmonary overcirculation, respiratory distress, poor growth, and eventually pulmonary vascular disease [6]. The diastolic blood pressure in the aorta decreases owing to diastolic “run‐off” into the pulmonary arteries, potentially resulting in decreased coronary perfusion pressure. This feature, coupled with increased intramyocardial tension from left ventricular dilation and increased oxygen demand, may result in subendocardial ischemia [13]. Conversely, a small PDA is usually not associated with significant hemodynamic effects and most patients are asymptomatic. PDA carries an increased risk of infective endocarditis, which is estimated at ~1% per year [6,14]. In patients with elevated PVR, ductal flow is either bidirectional (right‐to‐left during systole) or exclusively from the pulmonary artery to the aorta (right‐to‐left). In patients who have associated right heart obstructive lesions, a PDA provides a source of pulmonary blood flow. Conversely, in patients with obstructive left heart lesions, a PDA provides a source of systemic perfusion through the right heart. Congenital heart disease in which either the pulmonary or systemic circulation is dependent on blood flow from a PDA for survival is termed a “ductal‐dependent lesion.” A PDA with left aortic arch represents a persistent vascular connection between the aortic isthmus and the superior aspect of the proximal left pulmonary artery (Figure 19.1). In the absence of right ventricular outflow obstruction, the course of the PDA is similar to that of the aortic arch. However, the ductal arch can be easily distinguished from the aortic arch with the following features: (i) the ductal arch is inferior to the aortic arch; (ii) the ductal arch does not give rise to head vessels; and (iii) in postnatal life with normal PVR, the ductal flow is directed from the aorta to the MPA, while the aortic flow is in the reverse direction. Rarely there is a left aortic arch with a right PDA with the PDA arising from the right subclavian artery. Figure 19.1 High left parasternal view in color compare mode of a small patent ductus arteriosus (arrow) that is conical in shape and funnels at the pulmonary artery end. Ao, aorta; DAo, descending aorta; LPA, left pulmonary artery; RPA, right pulmonary artery. Right aortic arch occurs in approximately 0.1% of the population and is more common among patients with tetralogy of Fallot, truncus arteriosus, and tricuspid atresia. It is also highly associated with chromosome 22q11 deletion, particularly when there are arch branching abnormalities [15–18]. In individuals with normal intracardiac anatomy, a right aortic arch is often associated with aberrant origin of the left subclavian artery from the right descending aorta with a retroesophageal course [17]. The most common form of the right aortic arch is with mirror image branching of the head vessels and a left‐sided DA [19]. Recognizing the location of the DA in a right aortic arch is especially important, as it may be one of the components of a vascular ring (see Chapter 32) [20]. In normal fetal development, blood flow is directed from the MPA to the descending aorta through the DA. The right‐to‐left flow results in a configuration of the ductal arch with its concave portion facing downward. In circumstances when there is decreased or no flow across the right ventricular outflow tract in fetal life, blood flow is reversed in the DA and is directed from the descending aorta to the MPA. When flow in the DA originates from the aorta, the DA is typically long and tortuous, and the abnormal left‐to‐right flow results in a configuration of the ductal arch with its concave portion facing upward. This ductal course is often referred to as a “reverse‐oriented” or “vertical” DA. Reverse‐oriented DA is commonly seen in critical right heart obstruction including tricuspid atresia, pulmonary atresia/intact ventricular septum, and tetralogy of Fallot with severe pulmonary stenosis or atresia [21,22]. Bilateral DAs may be present in the setting of right aortic arch as described earlier, often with an aberrant left subclavian artery. Rarely, bilateral DA occurs as an isolated anomaly. Bilateral DA is also associated with congenital heart defects. In one study over two decades, 55% of bilateral DAs had their origin from bilateral nonconfluent branch pulmonary arteries to the aorta. This anomaly is likely a result of interruption in the development of the proximal sixth aortic arches (branch pulmonary arteries), with persistence of both distal sixth aortic arches (bilateral ductus) and continuity with the proximal arches. Bilateral DA has also been associated with interruption of the aortic arch with isolation of the subclavian artery and interruption of the aortic arch in which a bilateral DA supports the entire systemic circulation [23]. Ductal aneurysm is a rare congenital anomaly defined as marked dilation of the DA. The reported incidence varies from 0.8% at neonatal autopsy [24] to 1.5% in a prenatal series [25]. A study in term neonates demonstrated an incidence of 8.8% [26,27]. There is a strong association with chromosomal abnormalities or syndromes (25%) as well as with connective tissue disorders (13%) [25]. An increased incidence of ductal aneurysm has also been noted in infants of diabetic mothers [27]. The variability in reported incidence may be explained by the inconsistent diagnostic criteria and variable age at examination of ductal aneurysms [28,29]. The clinical course for ductal aneurysm is usually benign with closure in approximately 70% by 3 days of age [27]. Symptoms are uncommon but likely occur due to compression of adjacent structures, thrombus formation and extension, or erosion into the airways. Surgical resection is indicated for aneurysms persisting beyond the neonatal period or for those that develop complications [30]. In infancy, one of the most common indications for an echocardiogram is to screen for PDA. Echocardiography is necessary for early diagnosis of a PDA, especially in the preterm infant, due to the management implications of this finding [31–33]. The most accurate Doppler signal of the PDA flow is often obtained from the high left parasternal window, because this is the view where the transducer is nearest to the PDA and lines up well for assessment of flow. The direction and timing of ductal flow are valuable tools for assessing pulmonary artery pressure. Three ductal Doppler flow patterns are possible, depending on pulmonary artery vascular resistance and associated heart lesions: Figure 19.2 Continuous‐wave spectral Doppler interrogation of a small, restrictive patent ductus arteriosus with a peak velocity of 3.6 m/s in systole. Figure 19.3 Continuous‐wave spectral Doppler interrogation of a moderate size patent ductus arteriosus with left‐to‐right shunting and peak velocity of 3.5 m/s in systole. Figure 19.4 Pulsed‐wave spectral Doppler image from a parasternal short‐axis view of a large patent ductus arteriosus with bidirectional shunting; left‐to‐right in diastole and right‐to‐left in systole. With spectral Doppler, the late systolic peak velocity can be used to calculate the peak gradient across the PDA with the modified Bernoulli equation. The pulmonary artery systolic pressure can be calculated by subtracting the peak gradient across the PDA from the systolic blood pressure. These measurements correlate well with the peak instantaneous gradient between the aorta and pulmonary artery during cardiac catheterization [34]. The estimation of pulmonary artery pressure using this method is limited by the position of the sample volume, the artifact from the left pulmonary artery, and ductal anatomy. Once a PDA has been identified, it is important to determine whether the shunt is clinically significant. As the pulmonary vascular resistance falls after birth, left‐to‐right PDA shunting increases, resulting in increased pulmonary venous return. This eventually leads to left atrial and left ventricular enlargement. In the preterm infant, the following echocardiographic criteria may aid in recognizing a clinically significant left‐to‐right PDA [33,35]: A PDA with right‐to‐left flow can be difficult to identify because the flow profile through the duct appears similar to the flow in the descending aorta or left pulmonary artery. Anatomic assessment by 2D imaging accompanied by color Doppler is most important in these cases, as well as consideration of their clinical context. Detection of a right‐to‐left PDA can be supported by evidence of severe pulmonary hypertension (see Chapter 46) and arterial oxygen saturation differential between the upper and lower extremities (upper extremities > lower extremities). If there is restriction to a right‐to‐left ductus, the Doppler flow profile resembles that of coarctation of the aorta and the right ventricle should be considered suprasystemic. A restrictive right‐to‐left PDA can be differentiated from coarctation by: (i) imaging an unobstructed aortic arch and isthmus; (ii) clear imaging of flow originating in the MPA and coursing to the descending aorta via a PDA; (iii) evidence of severe pulmonary hypertension (see Chapter 46); and (iv) clinical correlation (normal lower extremity pulses and absence of blood pressure gradient from upper to lower extremities). Infants with profound pulmonary vascular disease may rarely develop systolic and diastolic flow reversal in the aortic arch. This is due to low cardiac output secondary to low pulmonary blood flow. In this situation, flow from the RV aids systemic perfusion to the cerebral circulation through the PDA and is an indication of decreased left ventricular outflow and worsening clinical status [36,37]. In most infants with a PDA, ductal flow can be visualized by color Doppler from the subxiphoid, apical, parasternal, and suprasternal notch views. Transthoracic imaging in older children may be difficult due to poor acoustic windows (see “Imaging of the adult” later in this chapter). High left parasternal and suprasternal notch views are the best windows for evaluation of a PDA in older children. Figure 19.5 Large patent ductus arteriosus (PDA). (a) Color Doppler image in a high left parasternal view of a large PDA with right‐to‐left shunting. (b) Spectral Doppler image from the suprasternal notch of a large PDA with all right‐to‐left shunting and a peak velocity of 2.0 m/s. DAo, descending aorta; LPA, left pulmonary artery. In the parasternal long‐axis view, ductal flow can be demonstrated with the transducer angled leftward and superiorly toward the distal portion of the right ventricular outflow tract and MPA. This acoustic window is only useful if the extension of the jet from the PDA to the MPA is relatively long. In the parasternal short‐axis view, the PDA is visualized by 2D along the left lateral border of the MPA, leftward of the left pulmonary artery. Cranial tilt of the transducer demonstrates the PDA. In the setting of a typical left‐to‐right PDA, flow is directed toward the transducer. Movement of the transducer superiorly to the high left parasternal window and clockwise rotation allows for visualization of the pulmonary artery bifurcation. From this view, counterclockwise rotation of the transducer toward 12 o’clock demonstrates the entire length and width of the typical PDA with its connection from the underside of the descending aorta to the left pulmonary artery. This view is often called the “ductal view.” It is not uncommon to see a color Doppler flow pattern in normal individuals that can be confused for PDA flow. In the parasternal short‐axis view, a flow signal directed toward the transducer can sometimes be seen along the medial aspect of the MPA in early systole. This flow pattern most likely represents helical flow within the MPA, a consequence of flow in a curved tube [35]. The medial location and systolic timing of the flow distinguish this flow profile from typical left‐to‐right ductal flow. With the aortic arch in view, a slight leftward tilt of the transducer demonstrates the left pulmonary artery. A slight clockwise rotation of the transducer will reveal the left pulmonary artery and the descending aorta in the same plane. Once both of these vessels are imaged simultaneously, the PDA, if present, will be visualized in this plane. Evaluation of a PDA associated with a right aortic arch begins with determination of arch sidedness. The suprasternal coronal view is the best acoustic window for assessing arch sidedness and brachiocephalic vessel branching order. However, the suprasternal frontal view can be used in a sweep to visualize which side of the mediastinum the aortic arch descends. With the transducer rotated toward 3 o’clock (coronal plane), sweeps should progress from anterior–inferior to superior–posterior. The transducer is initially angled to visualize the ascending aorta in cross‐section. Angling the transducer superiorly, the ascending aorta can be followed to the level of the transverse arch, and the innominate vein is visualized anterior to the distal ascending aorta. Just posterior to the innominate vein, the first brachiocephalic artery branches from the aortic arch. The direction of the first branch, the innominate artery, is opposite to the arch side in almost all cases. Once the side of the first brachiocephalic artery is determined, the next step is to determine whether this artery bifurcates. This is evaluated by following the vessel as it courses towards the neck. If it does not bifurcate, this branch is most likely the ipsilateral carotid artery, which can be confirmed by following it to the neck. In this situation, an aberrant origin of the subclavian artery from the proximal descending aorta is suspected. The aberrant subclavian artery is often visualized with the use of a color Doppler inferior view, separately from the carotid artery. Alternatively, the origin of the aberrant subclavian artery from the proximal descending aorta can be visualized from the suprasternal coronal view. The descending aorta is evaluated by 2D and color Doppler for an artery that courses medially and superiorly in the posterior mediastinum. In young patients, the proximal descending aorta can be evaluated for an aberrant subclavian artery from the subxiphoid windows. The PDA in a right aortic arch can originate from the proximal descending aorta (right PDA) and/or the base of the left subclavian artery (left PDA). Both of these sites must be interrogated with 2D, color, and spectral Doppler for potential arterial flow heading toward the pulmonary artery, consistent with a PDA. The PDA is visualized in its entirety, to determine its course. This can be achieved from a high parasternal view or suprasternal view. A right‐sided PDA courses from the proximal descending aorta to the origin of the right pulmonary artery. A left‐sided PDA courses from the left subclavian artery to the origin of the left pulmonary artery. Demonstration of a right aortic arch, aberrant left subclavian artery, and a left‐sided PDA is highly likely to be a vascular ring (see Chapter 32). A reverse‐oriented PDA arises from the underside of the aortic isthmus, and its tortuous course is best seen during visualization of the aortic arch in the suprasternal long‐axis view (Videos 19.4 and 19.5). Following the length of the PDA with color Doppler interrogation demonstrates its entire course (Video 19.4). Often it is difficult to visualize the entire length of the PDA in a single plane due to its tortuosity. If a reverse‐oriented PDA is identified, detailed assessment of the right ventricular outflow tract and pulmonary arteries is imperative to exclude stenosis or atresia of these segments. In particular, the pulmonary artery segment at or just distal to the duct insertion site should be imaged in detail for discrete stenosis or luminal discontinuity. Imaging of bilateral PDA requires a high index of suspicion and familiarity with the anatomic possibilities. Accurate diagnosis requires interrogation of the aortic isthmus and ipsilateral branch pulmonary artery as well as the base of the innominate artery or proximal subclavian artery on the contralateral side. The possibility of bilateral PDA should be investigated in the setting of a right aortic arch with aberrant origin of the left subclavian artery and when there are congenitally absent mediastinal branch pulmonary arteries. Bilateral PDA can also be seen with discontinuous branch pulmonary arteries associated with other congenital heart disease such as tetralogy of Fallot, interrupted aortic arch type B, isolation of a subclavian artery, and others. Echocardiographic evaluation, especially with color Doppler, of all possible sites of ductal insertion is required to exclude the presence of bilateral PDA (Video 19.6). When there is concern for bilateral PDA and possible discontinuous pulmonary arteries, additional imaging modalities such as magnetic resonance imaging (MRI), computed tomography (CT), or angiography may be required for accurate diagnosis, particularly in the newborn when considering initiation or discontinuation of prostaglandin therapy. Figure 19.6 Parasternal short‐axis image of a large ductal aneurysm. AoV, aortic valve; DA, ductal aneurysm; LA, left atrium; LPA, left pulmonary artery. When imaging from the high left parasternal transverse view, ductal aneurysm is suspected when unusual flow is noted from the PDA to the MPA. A large vessel is visualized leftward and superior to the left pulmonary artery with the restricted portion of the duct at its insertion into the MPA. Thus, three large vessels are seen from medial to left lateral position: the ascending aorta, MPA, and ductal aneurysm (Figures 19.6 and 19.7, Videos 19.7 and 19.8). A characteristic image of ductal aneurysm is visualized from a suprasternal short‐axis view. Angling the transducer posteriorly to image the descending aorta, the “rabbit‐ear sign” is seen. The right‐sided ear is the transverse aortic arch and the descending aorta, and the left‐sided ear comprises the ductal aneurysm. Ductal aneurysm generally demonstrates saccular dilation with a maximal internal diameter larger than that of the adjacent transverse arch or descending aorta [27]. Images that mimic ductal aneurysm include mirror image aliasing of the pulmonary artery, normal soft tissue shadow of the superior portion of the PDA, left superior vena cava, dilated left atrial appendage, and a vertical duct. Figure 19.7 Three‐dimensional reconstruction from a CT angiogram with contrast demonstrating a large ductal aneurysm and its relationship to the aortic arch (Ao arch) and left pulmonary artery (LPA). DA, ductal aneurysm. A PDA is a normal structure in the fetus and is generally quite large with flow from the pulmonary artery to the aorta in the normal heart. In those fetuses with right heart obstruction, a reverse‐oriented PDA may be seen and heralds severe disease. Assessment of the PDA in the fetus is a component of a complete fetal echocardiogram. Patent ductus arteriosus is uncommon in adults; however, when present it is associated with several complications including calcification, endocarditis, aneurysm formation, dissection, rupture, left heart volume overload, and pulmonary vascular disease. Transthoracic echocardiographic imaging of PDA in adults is challenging due to restricted acoustic windows. Imaging is best performed from the suprasternal view, using techniques similar to those described in pediatric patients. CT and MRI provide noninvasive evaluation of the PDA when echocardiographic evaluation is inconclusive. Cardiac MRI is preferred due to its ability to not only visualize the PDA, but also to assess its hemodynamic effects, including biventricular size and function and estimation of pulmonary‐to‐systemic flow ratio. In preterm infants, PDA closure can be induced by pharmacologic means. However, even after documentation of cessation of ductal flow, recurrence can occur. When medical therapy fails, surgical ligation and division via lateral thoracotomy is usually performed if the PDA is hemodynamically significant. Recently, transcatheter occlusion has been demonstrated in preterm infants (lower weight limit 2 kg) and is becoming the treatment of choice in this group [38]. Recent studies demonstrate feasibility in infants weighing as little as 700 g. Prior to closure, a complete echocardiogram is required to define the size and type of PDA and to assure that there is no structural heart defect, particularly ductal‐dependent disease. Measurements of the aortic and pulmonary end of the PDA as well as the PDA length are required to determine the appropriate choice of device for closure. Echocardiography can be used for procedural guidance in infants during device closure of the PDA as it limits radiation and contrast exposure [39]. Procedural echocardiograms typically focus on the PDA device after deployment but before its final release to assess for impingement of the left pulmonary artery and/or descending aorta. A high left parasternal view allows for visualization of the PDA device, aortic arch, and left pulmonary artery. These structures should be evaluated by 2D, color, and spectral Doppler to fully assess for obstruction to flow or vascular compression. The immediate post‐procedural echocardiogram can assess for residual PDA flow as well as evaluate for pericardial effusion or new valvular regurgitation. Finally, follow‐up echocardiograms after successful device closure should evaluate for residual PDA flow and left pulmonary artery or descending aorta narrowing in addition to the routine assessment of ventricular function, atrioventricular valve function, and evaluation for evidence of elevated right ventricular or pulmonary artery pressures [40]. In older patients, closure of the PDA can be performed via surgical ligation and division or by minimally invasive thoracoscopic clipping. In recent years, transcatheter occlusion has become the treatment of choice when feasible [6]. Transcatheter closure is achieved by endoluminal placement of a coil or occluding device into the PDA. After surgical or transcatheter PDA occlusion, echocardiography is utilized to assess for residual ductal shunt and/or inadvertent impingement of the left pulmonary artery or descending aorta. In patients after transcatheter PDA closure, echocardiography is also useful in identifying device position and inadvertent migration (Figures 19.8 and 19.9, Videos 19.9 and 19.10) [41]. Stenting of the PDA in ductal‐dependent cyanotic congenital cardiac anomalies has become a nonsurgical alternative to conventional systemic‐to‐pulmonary artery shunt surgery. In cases where ductal stenting is considered, echocardiographic evaluation of PDA origin from the aorta, site of insertion into the pulmonary artery, and its course (long and tortuous versus short and straight) is imperative. Accurate description of the PDA aids in determination of the feasibility of successful stent placement. Echocardiographic imaging following stent placement initially assesses for inadvertent occlusion of the pulmonary artery or descending aorta by the stent (Figure 19.10, Video 19.11). These patients are followed closely with echocardiography to exclude stent occlusion by thrombus formation or neointimal proliferation [42–44]. In normal anatomy, the MPA arises from the RV supported by an infundibulum and travels to the left of the ascending aorta in the direction of the left shoulder. As it bifurcates, the left pulmonary artery continues as a smooth arch and courses over the left bronchus. The right pulmonary artery arises at an acute angle and courses posterior to the ascending aorta and superior vena cava. Congenital anomalies of the pulmonary arteries include branch stenosis, hypoplasia, discontinuity, absence, and abnormal origin (e.g., from the ascending aorta). The MPA and its branches have their origins from three separate vascular components. The proximal portion of the pulmonary trunk develops from the cranial end of the bulbus cordis (conus cordis) and the distal portion of the pulmonary trunk is derived from the division of the truncus arteriosus into the MPA and ascending aorta. The proximal portions of the right and left pulmonary arteries are formed from the proximal portions of the right and left sixth arches. The distal portions of the pulmonary artery branches arise from the post‐branchial pulmonary vascular plexus [12]. Figure 19.8 Parasternal short‐axis image of a ductal occluder device (double arrow) causing near complete occlusion of the proximal left pulmonary artery (*). MPA, main pulmonary artery. Figure 19.9 Parasternal short‐axis color Doppler image of a ductal occluder device (double arrow) causing near complete occlusion of the proximal left pulmonary artery (single arrow) with minimal color flow around device. MPA, main pulmonary artery. Figure 19.10 Doppler color compare image from the suprasternal notch of a ductal stent placed as part of a hybrid procedure for hypoplastic left heart syndrome. The ductal stent (*) allows for unobstructed flow into native aortic arch (arrow). Congenital stenosis of the pulmonary arteries, isolated or in association with other cardiac defects or genetic syndromes, occurs in 2–3% of all patients with congenital heart disease [4]. The pathogenesis of branch pulmonary artery stenosis is not completely understood. It appears that multiple factors and many types of pathologic changes may produce this type of abnormality. When intracardiac anomalies are associated, the pathogenesis is likely developmental in origin. Certain teratogenic agents may interfere with the development of any of the components of the pulmonary artery tree and may lead to stenosis, hypoplasia, or atresia. Specific genetic abnormalities associated with pulmonary artery branch stenosis have also been identified [45]. The physiology associated with branch pulmonary artery stenosis depends on the severity and distribution of the stenoses. It may result in elevated RV and pulmonary artery (proximal to stenosis) systolic pressure. In most cases, the obstruction is central. Bilateral branch pulmonary artery stenosis results in right ventricular pressure overload and hypertrophy. Branch pulmonary stenosis may be mild and transient in the neonate. Physiologic branch pulmonary artery stenosis in infants is a common cause of functional murmur and sometimes results in referral for echocardiographic evaluation. It is characterized by a mild increase in branch pulmonary artery flow velocity, less than 2 m/s, in the absence of discrete anatomic narrowing or hypoplasia. The pulmonary artery stenosis in these cases usually resolves within several months [46]. The flow velocity may also be increased in the presence of high cardiac output states and atrial level shunts. Isolated branch pulmonary artery stenosis is characterized by diffuse arterial involvement generally affecting the main and lobar arteries. When severe, it produces symptoms of right ventricular failure [43,44]. Isolated branch pulmonary artery stenosis is often associated with a congenital syndrome, the most common being Alagille (often a JAG1 mutation) and Williams–Beuren (caused by a microdeletion at chromosome 7q11.23) syndromes [47–50]. In Alagille syndrome, branch pulmonary artery stenosis is found in 76% of patients, and 10% have tetralogy of Fallot [50]. Approximately 50% of patients with Williams–Beuren syndrome have peripheral pulmonic stenosis, which can be progressive [43]. Isolated branch pulmonary artery stenosis has also been associated with Noonan syndrome, cutis laxa, Ehlers–Danlos syndrome, Silver–Russell syndrome, and congenital rubella [7,8,51,52]. Although rare, familial and nonfamilial cases of isolated branch pulmonary artery stenosis with no underlying syndrome have also been described [53]. Branch pulmonary artery stenosis associated with congenital heart disease, especially conotruncal anomalies, is discussed in the respective chapters. An important cause of unilateral branch pulmonary artery stenosis, termed pulmonary artery coarctation, is PDA tissue encircling the origin of the branch pulmonary artery. The stenosis is discrete, and often the proximal portion of the affected pulmonary artery is distorted. This abnormality is present in 67% and 10% of patients with congenital pulmonary atresia and pulmonary stenosis, respectively, and more commonly occurs in the left pulmonary artery [54]. Early identification of this anomaly is imperative as early correction greatly impacts future pulmonary artery growth [55]. When the stenosis is unilateral, right ventricular pressure remains normal, because the normal contralateral branch pulmonary artery can accommodate the cardiac output without significant pressure increase. Flow to the stenotic side is reduced and the systolic pressure difference tends to underestimate the severity of the obstruction. Congenitally discontinuous branch pulmonary arteries may be due to regression of the proximal sixth arch segment as part of a conotruncal anomaly [23]. The incidence of chromosome 22q11 deletion is increased in these patients (45%), as compared with patients with left aortic arch with normal branching (3%) [56,57]. Discontinuous branch pulmonary arteries with bilateral DA are present in up to 16% of patients [58–60]. Anatomic variants of discontinuous branch pulmonary arteries include: (i) both branch pulmonary arteries are discontinuous with each other and with the right ventricular outflow tract – each pulmonary artery is supplied by either a DA or an aortopulmonary collateral vessel; and (ii) both branch pulmonary arteries are discontinuous with each other – one pulmonary artery is continuous with the right ventricular outflow tract and the other pulmonary artery is supplied by either a DA or a collateral vessel (Figure 19.11, Video 19.12) [61]. When recognized in the neonate, prostaglandin may be used to keep the pulmonary artery patent until surgery can be performed. Early diagnosis is important as growth of the affected branch pulmonary artery is most successful with early surgical recruitment and establishment of antegrade flow [56,62]. Congenital absence of a branch pulmonary artery is a rare anomaly most frequently associated with other congenital heart disease, especially tetralogy of Fallot. Other cardiac anomalies that have been associated with absent branch pulmonary artery include coarctation of the aorta, subvalvar aortic stenosis, transposition of the great arteries, and Taussig–Bing malformation. A few cases of isolated congenital absence of a branch pulmonary artery have also been reported [63]. The absent mediastinal pulmonary artery is usually contralateral to the side of the aortic arch. In fetal life and in neonates, the distal branches of the absent pulmonary artery are supplied by a DA or aortopulmonary collateral. The DA usually arises from the base of the subclavian artery contralateral to the side of the aortic arch and terminates on the side of the absent pulmonary artery at or near the hilum of the affected lung. Upon closure of the DA, a dimple is seen at the base of the subclavian artery [64]. Although some patients with isolated congenital absence of a branch pulmonary artery are completely asymptomatic, others may have severe pulmonary hypertension and congestive heart failure [65]. The origin of a branch pulmonary artery from the ascending aorta is a rare congenital cardiac abnormality. The developmental etiology is not well understood, but several theories exist. One consideration is incomplete migration of the affected branch pulmonary artery towards the contralateral pulmonary artery. Alternatively, the defect may result from failure of fusion of one of the sixth arches with the MPA [66]. An ascending aortic origin of a branch pulmonary artery is often associated with other cardiac anomalies, including tetralogy of Fallot, pulmonary atresia, and interrupted aortic arch [66–68]. It has also been associated with chromosome 22q11 microdeletion [68,69]. Figure 19.11 Discontinuous branch pulmonary arteries. High parasternal view in color compare mode showing an absent main pulmonary artery segment. The right pulmonary artery (RPA) arises from a tortuous ductus arteriosus. The left pulmonary artery (LPA) arises from the base of the innominate artery. Ao, ascending aorta. Typically, the anomalous branch pulmonary artery arises from the posterolateral wall of the ascending aorta above the aortic sinus. Alternatively, the pulmonary artery can originate distally from the ascending aorta near the origin of the innominate artery [68,70,71]. An origin of the right pulmonary artery from the ascending aorta occurs more commonly than anomalous origin of the left. Anomalous origin of the right pulmonary artery is invariably associated with a left aortic arch, and usually a left‐sided PDA. Anomalous origin of the left pulmonary artery from the ascending aorta is rare and is usually associated with a right aortic arch [66] and right‐sided PDA. In the setting of tetralogy of Fallot, the anomalous branch pulmonary artery is often larger than a branch pulmonary artery that arises normally from the MPA [67]. Physiologically, the lesion may result in abnormal pulmonary vasculature in the associated lung [67]. Stenosis of the affected branch pulmonary artery is unusual. Coronary ischemia is a reported adverse outcome, likely related to steal phenomenon or low aortic diastolic pressure [68]. Irrespective of the mechanism, early diagnosis and surgical repair is important to prevent the development of irreversible pulmonary vascular disease in the affected pulmonary artery [67,72]. In the past, the origin of a branch pulmonary artery from the ascending aorta was referred to as a “hemitruncus.” This anomaly is distinct from truncus arteriosus embryologically and morphologically, as it occurs in the setting of normal separation of the aortic and pulmonary valves. Aortic origin of a branch pulmonary artery should be distinguished from other cardiac defects associated with abnormal aortopulmonary flow such as PDA, major aortopulmonary collaterals, truncus arteriosus, or discontinuous branch pulmonary artery with ductal connection to the aorta. Normally, the right pulmonary artery originates from the MPA lower than the left pulmonary artery. In crossed pulmonary arteries, the left pulmonary artery ostium lies rightward and superior to the right pulmonary artery ostium. As a result, the branch pulmonary arteries cross each other proximally as they course to their respective lungs [73,74]. Despite the anomalous course of the crossed pulmonary arteries, a PDA usually arises from its normal position, at the base of the left pulmonary artery. Crossed pulmonary arteries may be confused with a left pulmonary artery sling, but can be distinguished by the posterior course of the left pulmonary artery to the left of the trachea [75]. In one study, approximately 50% of patients with crossed pulmonary arteries had a chromosome 22q11 deletion [74]. When crossed pulmonary arteries are associated with truncus arteriosus, there also tends to be an interruption of the aortic arch [76]. All patients referred for an echocardiogram due to suspected congenital heart disease or associated syndromes should undergo careful evaluation of the main and branch pulmonary arteries. Early identification of congenital abnormalities of the pulmonary arteries results in early surgical and/or transcatheter intervention and increased potential for pulmonary artery growth and improved right heart hemodynamics [55,56]. Careful examination of the pulmonary arteries is imperative after surgical repair involving manipulation of the MPA or branches, such as in the LeCompte maneuver for the arterial switch operation or in tetralogy of Fallot or truncus arteriosus repair. Swirling of color flow in the MPA is a normal variant that is characterized by systolic reversal of flow, usually in the rightward aspect of the MPA. Branch pulmonary artery stenosis can be subtle, and both 2D and color Doppler assessment are necessary to adequately check for narrowing. Careful attention to the Doppler color scale is important to not over‐ or underestimate the degree of branch stenosis. The branch pulmonary arteries are imaged from the parasternal and suprasternal windows (Figure 19.12, Video 19.13). From the suprasternal window, with the transducer turned toward 3 o’clock, the greatest length of the branch pulmonary arteries is visualized. Evaluation by 2D imaging includes determination of the extent of stenosis, measurement of the narrowest diameter, and assessment of post‐stenotic dilation. Note that measurements derived from color Doppler images often overestimate the vessel diameter. When color Doppler is employed, any flow reversal should be noted, as this is suggestive of pulmonary regurgitation, distal stenosis, increased stiffness, or elevated resistance. Discrete narrowing may be masked by the systolic color flow, but can be identified in diastole when reversed flow is noted. If any pulmonary regurgitation is noted with reversal in the branch pulmonary arteries, the diameter of reversed flow through the stenotic area will demonstrate more stenosis than the systolic forward color flow.
CHAPTER 19
Abnormalities of the Ductus Arteriosus and Pulmonary Arteries
Abnormalities of the ductus arteriosus
Definition
Incidence
Etiology
Morphology
Pathophysiology
PDA with left aortic arch
PDA with a right aortic arch
Reverse‐oriented PDA
Bilateral ductus arteriosi
Ductal aneurysm
Imaging
Doppler examination
Considerations for the left‐to‐right PDA shunt
Considerations for the right‐to‐left PDA shunt
PDA with left aortic arch
Parasternal views
Suprasternal window
PDA with right aortic arch
Assessment of PDA course
Reverse‐oriented PDA
Bilateral PDA
Ductal aneurysm
Parasternal view
Suprasternal view
Prenatal assessment
Imaging of the adult
Echocardiographic assessment during and after PDA closure
Preterm infants
Older children and adults
Echocardiographic assessment during and after PDA stenting
Abnormalities of the pulmonary arteries
Definition
Morphology
Congenital branch pulmonary artery stenosis
Incidence and etiology
Pathophysiology
Physiologic branch pulmonary artery stenosis
Isolated branch pulmonary artery stenosis
Branch pulmonary artery stenosis associated with congenital heart disease
Unilateral branch pulmonary artery stenosis
Abnormal pulmonary artery origin
Crossed pulmonary arteries
Imaging
Branch pulmonary artery stenosis
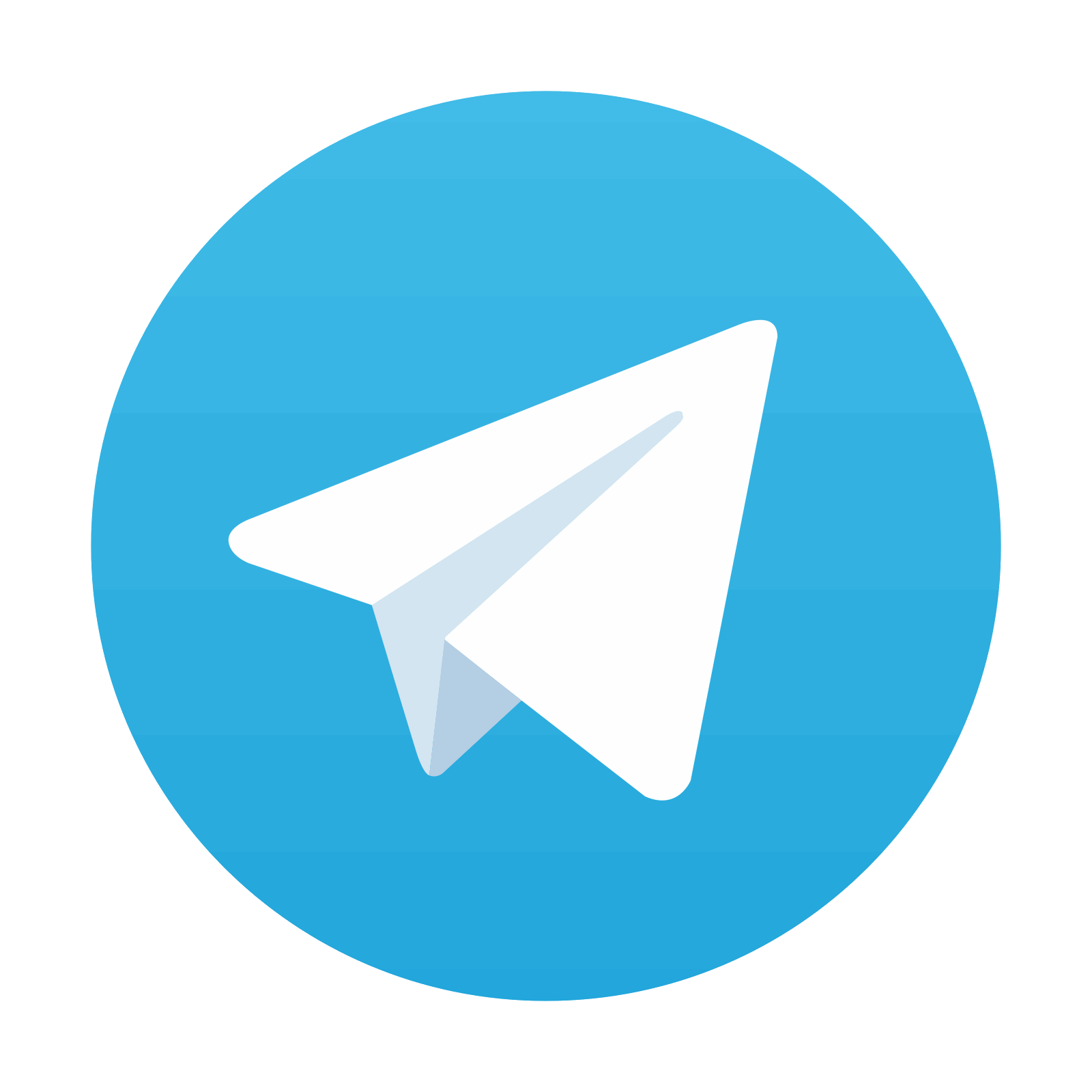
Stay updated, free articles. Join our Telegram channel

Full access? Get Clinical Tree
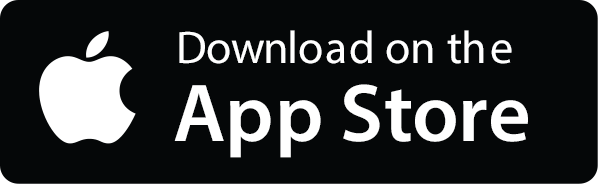
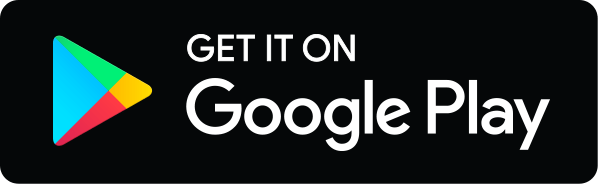