CHAPTER 20 The chicken and mouse as models of embryology
EARLY DEVELOPMENT OF THE CHICK EMBRYO
The three-week long development of a chicken embryo was first documented by Aristotle in the 4th Century B.C. Since then the domestic chicken (Gallus gallus) has been a favourite model organism in embryological studies. Today, modern hatcheries provide year round access to vast numbers of cheap and easily reared eggs. As the developmental stage can be accurately predicted at any given temperature, large numbers of embryos at defined stages can be obtained. Another advantage of the chick embryo is that it can be experimentally manipulated in a number of ways, from the surgical techniques used by John Saunders and Nicole Le Douarin in their classical experiments to current molecular techniques which, assisted by the nearly completed sequencing of the chick genome, allows forced expression and knock-down of genes via electroporation and/or use of retroviral vectors. Since both germ layer development (gastrulation) and subsequent organ formation is orchestrated by genes and cell movements similar to those of mammalian embryos, the chick embryos provide an important experimental system in which one can address fundamental questions about vertebrate development.
Fertilization, cleavage, and blastulation
Fertilization of the egg occurs in the oviduct prior to the secretion of albumen (‘egg white’) and the deposition of the shell. As with other eggs rich in yolk, cleavage occurs only in the blastodisc, a small disc of cytoplasm 2–3 mm in diameter. The horizontal cleavages create a single layered blastoderm where the cells are continuous with one another and with the yolk at their bases (Fig. 20-1). Later cleavages divide the blastoderm into a 5–6 cell layer thick tissue.
Between the blastoderm and the yolk is a space called the subgerminal cavity or space (Fig. 20-2A) which is created when the blastoderm cells absorb water from the albumen and then secrete it between themselves and the yolk. At this stage, the deep cells in the centre of the blastoderm are shed and die, leaving behind a one-cell-thick area pellucida which is the part of the blastoderm that forms most of the embryo proper (Fig. 20-1). The peripheral blastoderm cells retain their deep cells and constitute the area opaca. Between the area pellucida and the area opaca is a narrow layer of cells called the marginal zone some of which become very important in later development.
Gastrulation
Development of the hypoblast
By the time the egg is laid, the blastoderm contains approximately 20 000 cells. Most of the area pellucida cells stay on the surface and form the epiblast. A number of area pellucida cells delaminate and migrate into the subgerminal cavity where they form the primary hypoblast, a loose collection of cell groups containing 5–20 cells each (Fig. 20-2B). Next, a sheet of cells originating from a local thickening at the posterior margin of the blastoderm (Koller’s sickle) migrate anteriorly and push the primary hypoblast cells in front of them (Fig. 20-2C), thereby forming the secondary hypoblast, or endoblast. The resulting two-layered blastoderm (epiblast and hypoblast) is joined together at the marginal zone of the area opaca, and the space between the layers makes up the blastocoel. The hypoblast does not contribute any cells to the actual embryo; those cells come exclusively from the epiblast. Instead, the hypoblast cells form parts of the extraembryonic membranes, particularly the yolk sac and the vitelline duct – the stalk linking the yolk mass to the digestive tube. Hypoblast cells also provide chemical signals that direct the behaviour of epiblast cells.
Development of the primitive streak
The chief structural feature of amniote (avian, reptilian, and mammalian) gastrulation is the primitive streak. Dye-marking experiments, which allow cells to be traced as development progresses, suggest that primitive streak cells arise in the posterior marginal region. The streak is first visible as cells gather in the middle layer, followed by a thickening of the epiblast at the posterior marginal zone, just anterior to Koller’s sickle (Fig. 20-3A). The surrounding cells, which will later join the streak, become globular and motile, and they digest away the extracellular matrix beneath them. As the streak cells ingress they undergo convergent-extension. This process is responsible for the progression of the streak – a doubling in streak length is accompanied by a concomitant halving of its width. Those cells that initiated streak formation appear to migrate anteriorly and appear to comprise an unchanging cell population that directs the movement of epiblast cells into the streak.
As cells come together to form the primitive streak, a depression called the primitive groove forms within the streak. The primitive groove serves as an opening through which migrating cells pass into the blastocoel. The primitive groove is thus equivalent to the amphibian blastopore. At the anterior end of the streak is a thickening of the cells known as Hensen’s node. The centre of Hensen’s node has a funnel-shaped indentation through which cells migrate into the blastocoel. Hensen’s node is the functional equivalent of the dorsal blastoporal lip (also known as Spemann’s organizer) in amphibians.
The first cells to enter the primitive streak are endodermal progenitors (endoblast cells) from the epiblast (Fig. 20-3B). These cells go through an epithelio-mesenchymal transition and the underlying basal lamina breaks down. As these cells migrate into the streak, the streak extends in an anterior direction. Mitosis adds to the length generated by convergent-extension, and a number of the cells from the anterior part of the epiblast contribute further to the node. Simultaneously, the endoblast cells maintain their anterior migration away from the posterior edge of the blastoderm. The extension of the primitive streak appears to follow the anterior movement of the endoblast cells, and these are believed to direct the movement of the streak. In the end, the primitive streak will grow to ~70% of the length of the area pellucida.
Fate of cells ingressing through the primitive streak
As soon as the streak has developed, epiblast cells begin to move through it into the blastocoel to form mesoderm and endoderm (Fig. 20-4). Thus the primitive streak is formed by an always changing cell population. With respect to mesodermal components, the anterior end of the streak (Hensen’s node) gives rise to prechordal plate mesoderm, notochord, and rostral somites. Cells that migrate through the middle part of the streak give rise to somites, heart, and kidneys. Cell coming from the posterior part of the streak make the lateral plate and extraembryonic mesoderm (Fig. 20-3C–F).
The first cells that migrate through Hensen’s node form the pharyngeal endoderm of the foregut. These endodermal cells migrate rostrally and thereby displace the hypoblast, confining the hypoblast cells to the anterior part of the area pellucida. There they form the germinal crescent which does not form any of the embryonic structures but does contain germ cell precursors derived from the epiblast. The next cells moving through Hensen’s node also migrate rostrally, but they do not progress as far ventrally as the future foregut endoderm. Instead, these cells take up a location between the epiblast and the endoderm and form a portion of the head mesenchyme and the prechordal plate mesoderm. The head mesenchyme will later be enriched by contributions from the neural crest cells. The next cells to move through the node form the chordamesoderm. The chordamesoderm has two parts: the head process, which is formed by the medial mesodermal cells moving rostrally behind the prechordal plate mesoderm, and the notochord. The head process will underlie those cells that form the fore- and midbrain. When the primitive streak begins to regress, the cells migrating through Hensen’s node will lay down the second part of the chordamesoderm, the notochord. The notochord begins at the level where the ears and the hindbrain form, and extends caudally, where it will emit signals that instruct progenitor cells in adjacent tissues to adopt a specific cellular fate depending on the distance between the notochord and the responding progenitor cells. In fact, studies using chick embryos have delivered much of today’s detailed understanding of how the dorsal-ventral axis along the vertebrate spinal cord is patterned by signals from the notochord.
As the epiblast cells continue to move through the more posterior lateral parts of the streak they separate into two layers as they enter the blastocoel. The lower layer intercalates into the hypoblast and displaces its cells to the sides. These lower cells give rise to endoderm and will later form all of the embryo’s endodermal organs, as well as most of the extraembryonic membranes (with the hypoblast forming the rest). The other layer spreads between the epiblast and the endoderm forming a loosely connected layer of mesoderm. This mesodermal layer of cells gives rise to organs such as kidneys, heart, and vasculature as well as connective tissue parts of the endodermal organs. Also, the mesoderm lining the extraembryonic membranes is derived from these ingressed cells. The movement of these cells away from the streak is also controlled by FGF8 but a putative chemoattractive signal that can attract the cells towards the caudal end of the embryo has not yet been identified. By the end of the first day of incubation most of the future endodermal cells are located within the embryo but future mesodermal cells continue to move through the streak.
Now a transition of gastrulation occurs. While mesodermal cells continue to ingress, the primitive streak begins to regress, changing the position of Hensen’s node from near the centre of the area pellucida to a more posterior location (Fig. 20-5). The regressing streak generates the posterior dorsal axis of the embryo, including the notochord. As the node moves posteriorly, the caudal part of the notochord is laid down from precursors located in the node. Eventually, the node regresses to its most caudal position, forming the anal section. At this time, all the future meso- and endodermal cells have migrated into the embryo, and the remaining epiblast has gradually been transformed into the ectoderm.
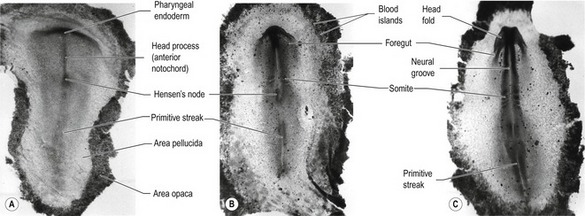
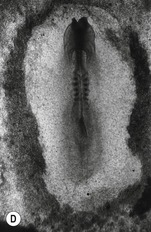
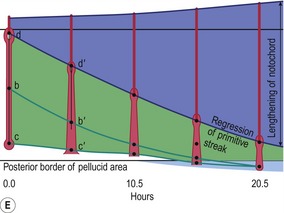
Fig. 20-5: Chick gastrulation 24–28 hours after fertilization. A: The primitive streak at full extension (24 hours). The head process (anterior notochord) can be seen extending from Hensen’s node. B: Two-somite stage (25 hours). Pharyngeal endoderm is seen anteriorly, while the anterior notochord pushes up the head process beneath it. The primitive streak is regressing. C: Four-somite stage (27 hours). D: At 28 hours, the primitive streak has regressed to the caudal portion of the embryo. E: Regression of the primitive streak, leaving the notochord in its wake. Various points of the streak (represented by letters) were followed after it achieved its maximum length. The x axis (time) represents hours after achieving maximum length (the reference line is about 18 hours of incubation).
Modified from Gilbert (2003). Reprinted with permission of Sinauer Associates Inc.
Axis formation
The dorso-ventral axis is set up when the blastoderm creates a barrier between the basic albumin above the blastodisc and the acidic subgerminal cavity below it. Water and sodium ions are transported from the albumin to the subgerminal cavity, creating a potential difference across the epiblast. The side facing the negative and basic albumin will become the dorsal side, and the side facing the positive and acidic subgerminal cavity fluid will become the ventral side. This orientation of the axis can be switched around experimentally by reversing either the pH gradient or the potential difference across the cell layer.
The ‘organizer’ of the chick embryo forms just rostral to the PMZ, where the epiblast and middle layer cells in the anterior part of Koller’s sickle form Hensen’s node. The posterior part of Koller’s sickle contributes to the posterior part of the primitive streak (Fig. 20-6). As mentioned above, Hensen’s node is considered to be the avian equivalent of the dorsal blastoporal lip in amphibians, since they are located at equivalent positions and share many properties. Thus, the cells of both can organize a second embryonic axis when transplanted into other locations of the gastrula (Fig. 20-7) and both are destined to become the chordamesoderm.
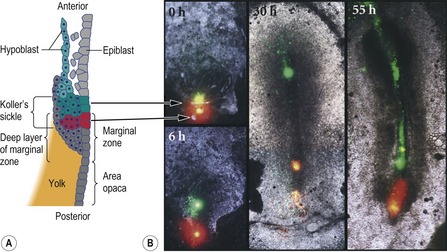
Fig. 20-6: Formation of Hensen’s node from Koller’s sickle. A: Diagram of the posterior end of an early (pre-streak) embryo, showing the cells labeled with fluorescent dyes in the photographs. Modified from Gilbert (2003). Reprinted with permission of Sinauer Associates Inc. B: Just before gastrulation, cells in the anterior end of Koller’s sickle (the epiblast and middle layer) were labeled with green dye. Cells of the posterior portion of Koller’s sickle were labeled with red dye. As the cells migrated, the anterior cells formed Hensen’s node and its notochord derivatives. The posterior cells formed the posterior region of the primitive streak. The time after dye injection is labeled on each photograph.
Reproduced with permission from Bachvarova (1998).
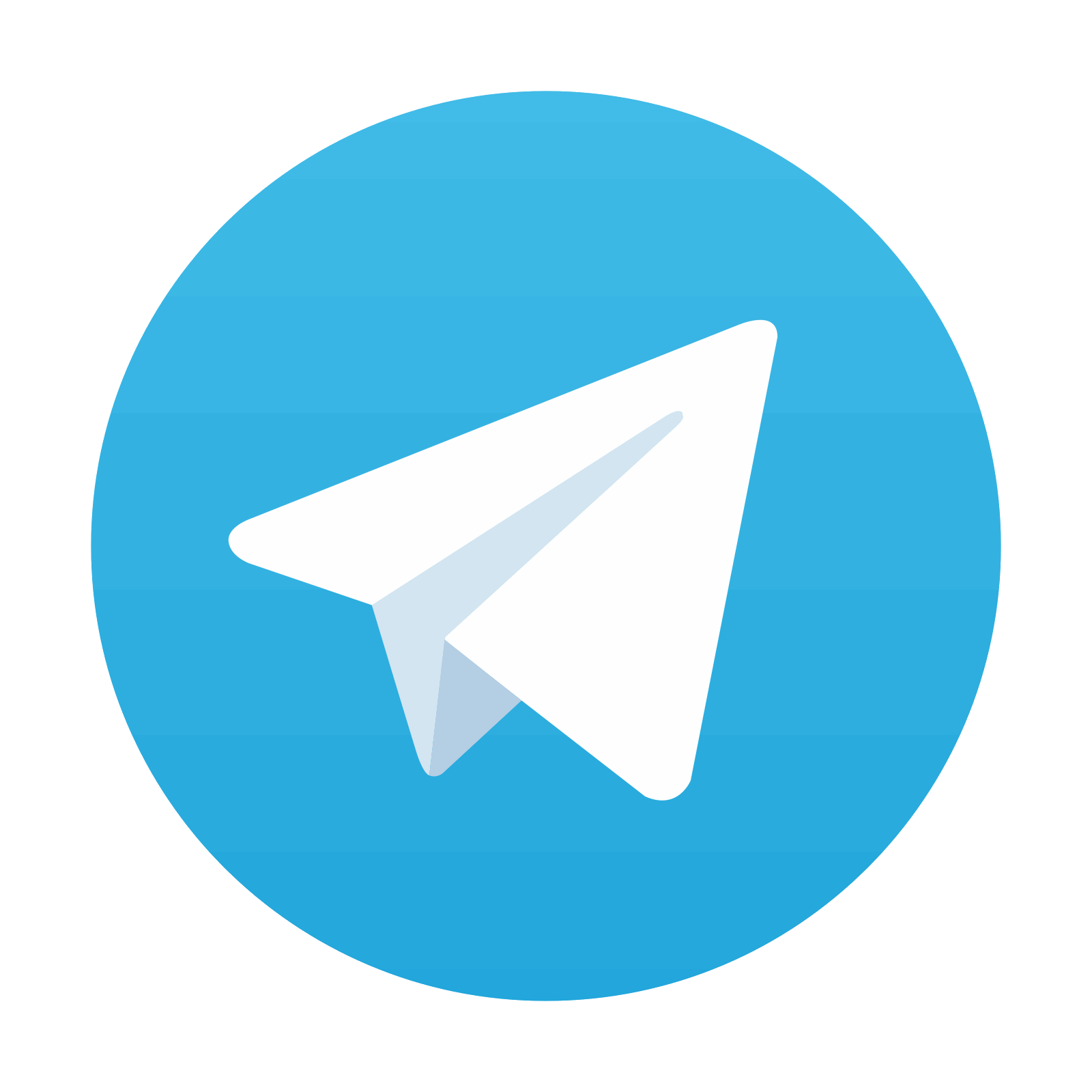
Stay updated, free articles. Join our Telegram channel

Full access? Get Clinical Tree
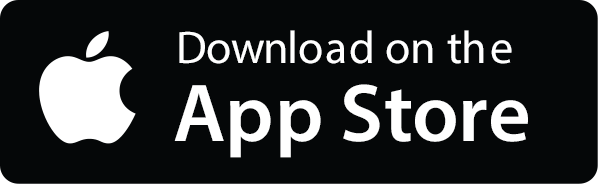
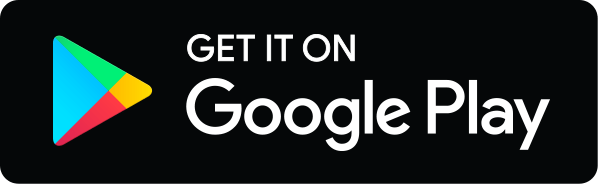