36 Urinary tract disease
Acute kidney injury and acute renal failure
Fortunately, ARF remains an uncommon problem in horses. Nevertheless, it is a serious disorder that if not properly recognized and managed often has a poor outcome. Reversible AKI usually develops as a complication of another disease process that causes hypovolemia and decreased renal perfusion (colic, colitis, hemorrhage, or exhaustive exercise). This may progress to ARF if renal hypoperfusion is not recognized and treated appropriately (Geor 2007). Treatment with nephrotoxic medications including aminoglycoside antibiotics, oxytetracycline (especially when administered at high dosages for correction of flexural deformities in neonatal foals), and nonsteroidal anti-inflammatory drugs (NSAIDs) as well as exposure to endogenous pigments (myoglobin or hemoglobin) or heavy metals (mercury, cadmium, zinc, arsenic, and lead) or excessive administration of vitamin D or vitamin K3 can also cause AKI and ARF (Schmitz 2007). Hemodynamically-induced AKI is often associated with oliguria (urine output <0.5 ml/kg for >6 hours) whereas urine production with nephrotoxin-associated AKI often remains normal (non-oliguric AKI).
Clinical signs in horses with AKI and ARF most commonly reflect the primary disease process: for example, colic, diarrhea, or restricted gait and pigmenturia due to rhabdomyolysis (Geor 2007). Oliguria or anuria followed by development of edema in the face of appropriate fluid therapy are alarm signs for ARF. More subtle clinical signs that should prompt investigation of AKI in non-oliguric patients include more severe lethargy and inappetance than would typically be expected with the primary disease.
When these clinical signs are observed, serum biochemical analysis is indicated to assess for azotemia as well as alterations in serum electrolyte concentrations and acid–base balance. Azotemia is the term used to describe increases in blood urea nitrogen (BUN) and creatinine (Cr) concentrations detected on a serum chemistry profile; thus, azotemia is a laboratory diagnosis. Azotemia can be prerenal in origin, consequent to decreases in renal blood flow (RBF) and glomerular filtration rate (GFR) or can be due to primary (intrinsic) ARF or disruption of the urinary tract (post-renal failure) (Bayly 1991). The term “prerenal failure” has been used to describe reversible increases in BUN and Cr associated with renal hypoperfusion. Serum electrolyte concentrations are generally within reference ranges and urine specific gravity is increased (>1.025 but often approaching 1.045–1.050) with prerenal failure, due to maintenance of concentrating ability (Brobst et al 1977, Grossman et al 1982). Although use of this term is firmly entrenched in both the human and veterinary medical literature, it likely contributes to a lack of recognition of subclinical renal damage that accompanies a number of medical and surgical conditions. This can be attributed to a large renal functional reserve capacity. In many patients with reversible azotemia, changes in glomerular and tubular function and integrity can be demonstrated by proteinuria and cast formation, impaired concentrating ability (urine specific gravity of 1.015–1.025 in a markedly dehydrated patient), and increases in urine sodium concentration (>20 mmol/l) and excretion (Seanor et al 1984).
To increase awareness of subclinical renal damage in patients with decreased RBF and GFR, the term acute kidney injury has been introduced in human and, subsequently, small animal medicine. AKI has been defined as an increase in Cr of as little as 0.3 mg/dl (≈25 µmol/l) within 24–48 hours of onset of disease or hospital admission, even when the upper limit of the reference range may not be exceeded (Mehta et al 2007, Lattanzio & Kopyt 2009). Further evidence to support subclinical renal damage with AKI includes the abnormal urinalysis findings mentioned above as well as biochemical analysis of urine to detect increased enzyme activity (e.g., urine gamma glutamyl transferase activity to urine Cr ratio [IU/g × 0.01] exceeding 25, indicative of sloughing of proximal tubule epithelial cells into the tubule lumen) (Adams et al 1986) and, in other species, detection of novel urine biomarkers such as kidney injury molecule-1 (Han et al 2008).
Metabolic changes accompanying AKI and ARF
Oxygen consumption, a measure of energy expenditure, in patients with uncomplicated ARF is similar to that of healthy individuals. In contrast, oxygen consumption may increase by 20–30% in patients with sepsis or multiple organ dysfunction syndrome (MODS) complicated by ARF (Schneeweiss et al 1990). Thus, energy requirements are determined more by the primary disease process than ARF.
Most patients with ARF have a poor appetite, leading to a negative energy balance. A decreased carbohydrate intake and depletion of hepatic glycogen stores in ARF leads to increased oxidation of fatty acids to meet energy demands. Increased mobilization of triglycerides to support this process is accompanied by development of hyperlipidemia with increased triglyceride content of plasma lipoproteins, especially very low density lipoproteins (VLDLs) and low density lipoproteins (LDLs) (Druml et al 1985). These lipid abnormalities in ARF are a consequence of impaired lipolysis by both hepatic triglyceride lipase and peripheral lipoprotein lipase, with metabolic acidosis further inhibiting the latter (Druml et al 1985). Despite prolonged insulin action due to decreased renal clearance, as well as decreased hepatic insulin degradation, maximal insulin-stimulated glucose uptake by muscle is decreased and muscle glycogen synthesis is impaired, supporting tissue resistance to insulin (May et al 1985). Consequently, both hypertriglyceridemia and hyperglycemia are common findings in patients with ARF.
Next, protein catabolism leading to a sustained negative nitrogen balance is a hallmark of ARF. In addition to inadequate intake, metabolic effects of uremic toxins along with metabolic acidosis cause accelerated protein degradation and impaired amino acid uptake by muscle cells. Release of cytokines and proteases during a systemic inflammatory response, coupled with increased secretion of catecholamines and glucocorticoids, also contribute to protein catabolism. Further, insulin-stimulated muscle protein synthesis is suppressed with ARF, again due to accelerated protein catabolism and insulin resistance. Another important mechanism of protein breakdown with ARF is stimulation of hepatic gluconeogenesis from amino acids (Druml 1998). Glucose administration to healthy subjects completely suppresses hepatic gluconeogenesis from amino acids; however, in patients with ARF hepatic gluconeogenesis can only be partly suppressed by glucose infusion, an effect mediated by a glucocorticoid dependent pathway (Cianciaruso et al 1991). Thus, no matter what nutritional support is provided to patients with ARF, it is impossible to fully counteract a negative nitrogen balance during the acute stage of ARF.
Nutritional management of AKI and ARF
Nutritional support for human patients with AKI and ARF is focused on limiting protein and energy wasting that commonly accompanies renal failure (Druml 2005, Fiaccadori et al 2008, Fiaccadori & Cremaschi 2009). Benefits of nutritional support include promoting tissue repair and supporting immune function, with the ultimate goal of reducing mortality. The enteral route is preferred; however, concurrent partial or total parenteral nutrition (TPN) is often required to meet nutritional needs, especially in patients in which the primary disease precludes enteral feeding. As described above, human patients with AKI or ARF are prone to developing hyperglycemia and hypertriglyceridemia as well as electrolyte and acid-base derangements. Judicious administration of intravenous (IV) fluids is the mainstay therapy to maintain hydration and normalize serum electrolyte concentrations and acid-base balance. A detailed discussion of fluid therapy is beyond the scope of this chapter and interested readers are referred to recent reviews (Langston 2008, Prowle et al 2010, Schrier 2010). Human, as well as companion animal, patients in which renal replacement therapy is pursued, by either intermittent peritoneal dialysis or hemodialysis, may derive benefits from greater control of uremia; however, fluid exchange procedures may further challenge macronutrient and micronutrient losses (Fiaccadori & Cremaschi 2009, Bloom & Labato 2011). Similar principles should also apply to nutritional support of horses with AKI and ARF.
In equids with AKI or ARF, the initial approach to nutritional support consists of offering a variety of highly palatable feeds to encourage voluntary intake, with fresh grass (that may need to be cut and brought to the patient) often the most readily accepted feedstuff. When patients remain completely anorectic for 48 hours or more, forced enteral feedings are recommended to provide energy and protein (Carr & Holcombe 2009). Enteral feedings can be made by pulverizing and moistening commercially available pelleted feeds (complete “senior” feeds are preferred due to their typically higher fiber content). Administration of 1 kg of feed in 6–8 liters of water via a nasogastric tube six to eight times a day can provide 15 to 18 Mcal (30–36 kcal/kg/day), essentially meeting maintenance energy requirements for a 500 kg horse (Carr & Holcombe 2009; see Chapter 41). Alternatively, low residue diets designed for humans (Vital HN™, Osmolite™) or horses (Critical Care Meals™, Platinum Enteral Immunonutrition Formula™, WellSolve Well-Gel™) can also be provided as liquid formulations through smaller diameter indwelling nasogastric tubes (Sweeney & Hansen 1990, Buechner-Maxwell et al 2003). Unfortunately, these commercial “liquid diets” are considerably more expensive and have not been documented to be of greater benefit than complete pelleted feeds, providing feeding of the latter is tolerated.
In human patients with AKI, nutritional support has targets of at least 1.5 g/kg protein and 30 kcal/kg non-nitrogen calories with lipids providing 30–35% of total calories per day (Fiaccadori et al 2008). Although no data exist for equids with AKI or ARF, these goals would seem reasonable targets as well, perhaps with a lower percentage of calories derived from fat because most equids would likely be less adapted to fat in their diets. Fat limitation may be even more important to consider in patients that are predisposed to hyperlipidemia (e.g., ponies, Miniature horses, donkeys, and burros) or that have grossly lipemic plasma prior to nutritional supplementation. Generally, enteral feeding with pelleted feeds or commercial low residue diets should also provide an adequate amount of protein; however, protein catabolism will not be completely attenuated in patients with ARF for the reasons described above. A further benefit of providing enteral nutrition is local nutrient delivery to enterocytes, thereby helping to maintain their function as well as integrity of the intestinal mucosal barrier (Carr & Holcombe 2009).
In addition to being cautious about the amount of fat added to the diet, the type of fat supplemented may also have an impact on severity of renal damage. Specifically, although study findings are somewhat conflicting, supplements rich in omega-3 polyunsaturated fatty acids (PUFA), as compared to fat supplements that are largely omega-6 PUFA, have been documented to slow progression of CKD in human and small animal patients (Fassett et al 2010, Roudebush et al 2010). However, recent data suggest that short-term feeding of omega-3 PUFA may also be “renoprotective” during AKI. For example, mice that were fed an omega-3 PUFA-enhanced diet (fish [menhaden] oil with 28% omega-3 PUFA, providing 5% of total calories) as compared to mice that had been fed a diet with corn oil (2% omega-3 PUFA, providing 5% of total calories) for 4 weeks had dramatically improved survival following experimental renal ischemia (Hassan & Gronert 2009). Novel omega-3 PUFA-derived autacoids, in particular protectin D1, appear to exert potent anti-inflammatory effects and limit neutrophil influx during the acute inflammatory response (Hassan & Gronert 2009). Despite the fact that acute dietary supplementation with omega-3 PUFA to equids at the onset of AKI and ARF may have little or no immediate renoprotective effect, their use in nutritional support may still be preferred over supplementation with vegetable oils that contain minimal omega-3 PUFA.
When enteral feeding is either not possible (e.g., with nasogastric reflux due to ileus) or not well tolerated (e.g., produces colic signs), TPN will need to be pursued. There have been no investigations of altering common TPN formulations of dextrose, amino acids, and lipid emulsions for supportive care of horses with ARF; thus, a recommended approach would be to initiate TPN with routine formulations (Carr & Holcombe 2009) with close monitoring of serum glucose and triglyceride concentrations. In patients with significant hyperglycemia (>200 mg/dl ≈10 mmol/l) or hyperlipidemia (triglycerides >500 mg/dl ≈6 mmol/l), it would seem prudent to initiate TPN using formulations with reduced amounts of dextrose and lipid and to monitor changes in these values over the initial few hours of administration. To facilitate use of TPN in patients with ARF, it may be useful to initially prepare TPN in smaller volumes (e.g., 1–2 liters) until a formulation that is reasonably well tolerated is found. Finally, because uremia can increase the risk of gastrointestinal ulceration, equids with ARF should receive concurrent anti-ulcer drugs, preferably a proton pump inhibitor, in an attempt to limit development of gastric ulcers.
Key Points
• Energy and protein malnutrition along with hyperglycemia and hyperlipidemia are common problems with acute renal failure
• Because acute kidney injury and acute renal failure are typically consequences of other primary diseases, nutritional support for recovery from the renal insult must also address nutritional requirements for the primary disease
• The initial approach to nutritional support includes offering a variety of feedstuffs, especially fresh grass, to encourage voluntary feed intake
• In anorectic horses, enteral and parenteral nutrition are often required to attenuate energy and protein malnutrition
Chronic kidney disease
Although chronic renal failure (CRF) is also fairly uncommon in equids (Schott et al 1997), the primary management challenge is maintenance of body condition of affected horses. Thus, nutritional support is a key aspect of long-term care.
A change in terminology has also recently been adopted in human and small animal medicine for patients with chronic renal disease. Rather than describing patients as having CRF (typically an end stage problem), the term chronic kidney disease has been introduced to shift attention to detection of earlier stages of chronic renal disease (Winearls & Glassock 2009; http://www.iris-kidney.com/guidelines/en/staging_ckd.shtml). Although CKD by nature is a progressive disorder, earlier recognition and intervention may slow rate of progression and thereby prolong life and, for people and small animals, delay the need for renal replacement therapy (Finco et al 1999, Ruggenenti et al 2001).
In contrast to human and small animal patients in which glomerular disease, accompanied by proteinuria, is a common cause of CKD, most horses with CKD have tubulointersitial disease, termed chronic interstitial nephritis (CIN). In some instances, a history of neonatal disease, a serious medical or surgical problem, or treatment with nephrotoxic medications may be associated with subsequent onset of CIN but often a precipitating cause or event is not recognized. Less commonly, anomalies of development (renal agenesis or hypoplasia, renal dysplasia, or polycystic kidney disease) may be the cause of CKD in equids (Schott 2007). Horses with CKD are often presented for evaluation of weight loss; however, for horses in competition, loss of condition, decreased performance, and a dull hair coat may be earlier complaints before weight loss becomes evident. Additional findings may include mild ventral edema, polyuria and polydipsia (PU/PD), excessive dental tartar and halitosis (associated with gingivitis attributed to urease producing bacteria that result in excess ammonia production), and a uremic or “fishy” odor to the hair coat (as urea can also be eliminated through sweat glands) (Schott et al 1997). Regardless of the presenting complaint, a diagnosis of CKD is generally made by detection of azotemia and isosthenuria (urine with a tonicity similar to plasma and a specific gravity ranging from 1.008–1.014), typically in a euhydrated patient. Depending on the diet being consumed, hypercalcemia is also sometimes found in equids with CKD (Leroy et al 2011) and the combination of azotemia, hypercalcemia, and isosthenuria is essentially pathognomonic for CKD in equids. Further clinical evaluation often includes transabdominal ultrasonographic imaging that may reveal increased echogenicity of the renal parenchyma, consistent with interstitial fibrosis, and nephrolithiasis providing further support that the disease is chronic in nature (Schott 2007).
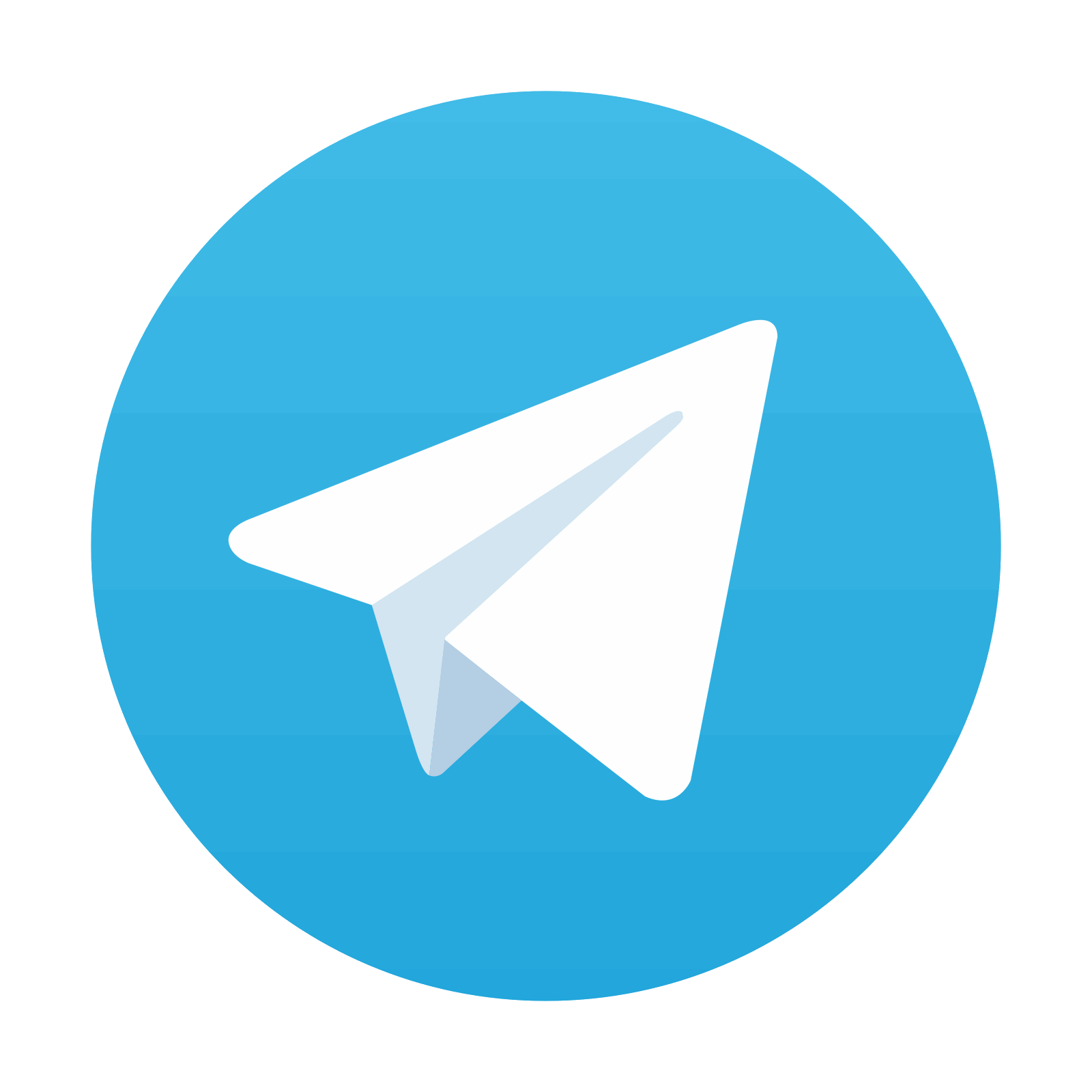
Stay updated, free articles. Join our Telegram channel

Full access? Get Clinical Tree
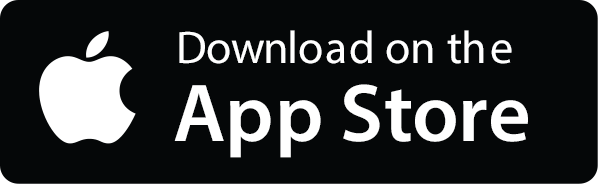
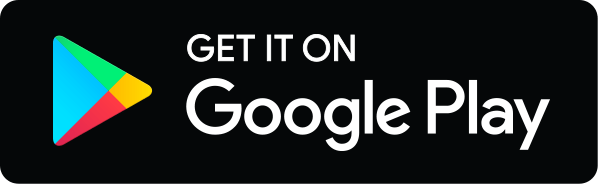