Species
Enzymes
pH optimum
Localization
R. prolixus
β-Acetylglucosaminidase
4.5
st
R. prolixus
α-Galactosidase
4.5
st, si
R. prolixus
α-Glucosidase
4.5
st, si
R. prolixus
β-Glucosidase
4.5
si
R. prolixus
α-Mannosidase
4.5
st, si
R. prolixus
β-Mannosidase
4.5
si
R. prolixus
Alkaline phosphatase
10.4
st, si
R. prolixus
Acid phosphatase
4.5
st, si
R. prolixus
Aminopeptidase
8.0
st, si
R. prolixus
Carboxypeptidase A
n.d.
si
R. prolixus; T. infestans
Cathepsin D-likea,b
3.5
sic
R. prolixus; T. infestans
Cathepsin B-liked
5.5
si
R. prolixus; T. infestans
Cathepsin L-liked,e
5.5
si
R. prolixus; T. infestans
Lipasesf
7.0–7.5
st, si
In all nymphal instars and adults, the blood is processed by lysis of erythrocytes (Azambuja et al. 1983). This hemolytic activity in the stomach is highest 2–4 days after feeding. Erythrocytes of different blood sources are efficiently lysed by stomach contents of Rhodnius prolixus, illustrating a similar lysis mechanism for a broad spectrum of hosts (Azambuja et al. 1983). Highest rate of hemolysis was induced by feeding on whole blood, while similar but considerable lower activities were observed by feeding pure hemoglobin or erythrocytes. After partial purification and biochemical characterization, the hemolytic factor of R. prolixus was classified as a basic peptide which is released to the stomach lumen and activated upon blood ingestion. This hemolytic activity is restricted to the lumen of the stomach, and it is not associated with the stomach wall tissue or the small intestine.
In contrast, proteolytic activity was only found in the small intestine after a transport of small portions of blood. Using azocasein as substrate, no activity was detectable in the stomach of R. prolixus (Azambuja et al. 1983). However, high expression rates of cathepsin-like genes—suggested to encode digestive enzymes—are also evident in the stomach tissue (Balczun et al. 2012; Ribeiro et al. unpublished). Using hemoglobin as substrate in determinations of the activity of another digestive enzyme, cathepsin D, after depletion of stomach contents, only a slight activity occurred in homogenates of the stomach wall of T. infestans, even at the optimal pH 3 (Gajewski and Schaub unpublished). However, the pH in the lumen of the intestine never possessed acidic conditions that are optimal for the activities of cathepsin D. A careful analysis of cathepsin D-like activity of stomach contents is necessary to elucidate if blood proteins are not processed in the stomach of triatomines, neither by cathepsin D nor by cathepsins B and L (see below).
Using homogenates of the small intestine of R. prolixus and Triatoma phyllosoma pallidipennis, activities of different exopeptidases and endopeptidases were identified (Houseman 1978; Houseman and Downe 1980, 1981a, b; Terra et al. 1988). Carboxypeptidase A and a lysosomal carboxypeptidase B were detected in homogenates of the midgut and small intestine (Garcia and Guimarães 1979; Houseman and Downe 1981b), and at least three aminopeptidases were localized between microvillar membranes or on the surface of cells of the small intestine (Ferreira et al. 1988). The aspartate protease cathepsin D and the cysteine protease cathepsin B of R. prolixus were identified in the luminal contents of the small intestine by their pH optima and using specific substrates (Houseman and Downe 1983a, b). For cathepsin B, D and the lysosomal carboxypeptidase B, high activities were measured 6 days after feeding, and a second peak of cathepsin B activity occurred at the time of ecdysis (Billingsley and Downe 1988; Houseman and Downe 1983b). In R. prolixus, cathepsin B was suggested as the major digestive protease, while cathepsin D should contribute only to a minor extent to the overall proteolytic activity (Terra et al. 1988).
However, molecular biological investigations of R. prolixus, Triatoma brasiliensis and Triatoma infestans identified the nucleotide sequences not only of the cysteine protease cathepsin B but also of another cysteine protease, cathepsin L (Lopez-Ordoñez et al. 2001; Kollien et al. 2004; Waniek et al. 2012). Since the previous measurements of the activities of cysteine proteases covered the activities of cathepsin B as well as cathepsin L without differentiation, the relative impact of both proteases on blood digestion is an open question. Despite the common GCDGG motif, the triatomine cysteine proteases share characteristic motifs of the respective family C1 of cysteine proteases, in particular the ERFNIN and GNFD motifs of cathepsin L and the “occluding loop” of cathepsin B (Schaub et al. 2011).
Two isoforms of cathepsin D-like protease encoding genes were characterized in T. infestans (Balczun et al. 2012). The expression level of TiCatD in the small intestine is immediately and strongly induced after feeding, while expression of TiCatD2 is induced much later and at a considerably lower level. Both proteases were identified to be active in the lumen of the small intestine. This is remarkable since TiCatD2 possesses a motif called “proline loop” which is suggested to be a characteristic motif of lysosomal cathepsin D-like proteases (Padilha et al. 2009). This is another indication for the peculiarities of the digestion of triatomines.
Several isoforms of the cysteine and aspartate protease encoding genes of triatomines are available in GenBank. In addition, the analysis of the transcriptome of R. prolixus midgut reveals the existence of multiple transcript variants of the respective genes (Ribeiro et al. unpublished). Apparently, protein digestion in triatomine midguts is a complex process with a concerted interaction of endopeptidases and exopeptidases, and therefore, elucidation of putative regulatory networks of proteases in the midgut of triatomines demands further sophisticated approaches.
12.3.3 Excretion
After ingestion of such enormous volumes of blood, the nymphs can hardly move. Therefore, the most effective excretion system in the animal kingdom is already induced during blood ingestion (Maddrell 1969; Maddrell et al. 1991). Ions of the blood are transported into the hemolymph and then into the Malpighian tubules, followed by the water. The urine flows into the rectum, sweeping out the remnants of digestion. Thereby, within 24 h, the bugs excrete 76% of the imbibed fluid part of the blood (Schaub 2009).
12.4 Symbionts and Antibacterial Compounds of Triatomines
Like many blood-sucking insects, triatomines require symbionts for a normal development (summarized by Schaub 2009). These develop in the anterior midgut, cardia and stomach and are mainly lysed in the small intestine. Symbionts are obtained by coprophagy, a risk of a contamination by other bacteria. In addition, there are other occasions at which bacteria might enter the digestive tract (summarized by Schaub 2009). Therefore, the ingested sterile blood has to be protected from an uncontrolled growth of microorganisms, but the growth of the essential symbionts and their utilization has to be assured.
Saliva is the first defence mechanism against oral infections. So far, only three antibacterial proteins were described from the saliva of triatomines, trialysin, lysozyme and defensin, possessing molecular masses of 30, 13 and 5 kDa (Amino et al. 2002; Waniek et al. 2009; Klenner and Schaub unpublished). In the saliva of T. infestans, proteins with molecular masses of 14–17 kDa showed the highest bacteriolytic activity in zymography using Gram-positive bacteria as substrate (Klenner and Schaub unpublished). Lysozymes possess such a molecular mass, and bacteriolytic activity in extracts of the midgut of R. prolixus and T. infestans was particularly attributed to these proteins (Ribeiro and Pereira 1984). In addition, several lysis bands in higher molecular ranges are visible in such approaches. Since such zymograms do not allow an identification of the active compounds, the origin of the activities remains to be identified. Besides trialysin and lysozymes, the low-molecular-mass defensins, which are expressed in the digestive tract as well as in the salivary glands of triatomines (Waniek et al. 2009), might attribute to the antibacterial action. However, their mode of action and their putative role in immune responses require detailed investigations (Bartholomay et al. 2004).
The high number of different bacteriolytic components in the saliva indicates a more general function at the entrance of the intestine. Here, activity against a broader spectrum of bacteria is apparently necessary. The need for such a defence during oral infections, e.g. during coprophagy, is shown by the pathogenic effects occurring after feeding of blood containing faeces (Schaub unpublished). However, the saliva compounds are not effective against all bacteria indicated by the high number of different species of microorganisms isolated from triatomines (Vallejo et al. 2009).
12.5 Trypanosoma cruzi: Taxonomy and Developmental Cycle
The protozoan flagellate T. cruzi belongs to the order Kinetoplastida which have arranged concentrated extranuclear DNA including the mitochondrial DNA in a unique order in a specific region of the mitochondrium, the kinetoplast. In the family Trypanosomatidae, all members are parasites, and in the genus Trypanosoma, nearly all are heteroxenous, i.e. the development includes a host change between a blood-sucking invertebrate and a vertebrate. In the respective host, different forms, phenotypes, develop (Walochnik and Aspöck 2010).
According to recently established molecular biological criteria, the species T. cruzi is divided into six discrete typing units (DTU), TcI–VI (summarized by Schaub et al. 2011). The genetic structure of the populations is mainly clonal, but restricted genetic recombinations occur. Presumably, TcI and TcII are the most anciently divergent, TcIII and TcIV resulted from ancient hybridization events between TcI and TcII, and TcV and TcVI are hybrids from TcII and TcIII (Patterson and Guhl 2010). TcI is suggested to have developed north of Amazonia, closely associated with arboreal triatomines, e.g. Rhodnius sp., and arboreal marsupials, e.g. Didelphis sp., whereas TcII–TcVI developed in the south, associated with terrestrial triatomines, tribe Triatomini, and armadillos. At present, in some South American countries, up to four different DTUs occur, and a more geographic than host association is evident (Patterson and Guhl 2010). The strong migrations of humans introduce new strains to different regions. However, this does not generally induce a long-term establishment at the new location. Local mammals can be refractory to the respective strain, acting as “filters” (Jansen and Roque 2010).
Usually, an ingestion of parasites with the blood of an infected host initiates the development in the vector. These parasites, blood trypomastigotes, transform in the stomach to other stages, especially sphero- and epimastigotes. These stages initially multiply in the small intestine, then after passage to the rectum also there. Only in the rectum the development of the non-dividing metacyclic trypomastigotes is finished (reviewed by Kollien and Schaub 2000). All stages are present in the faeces and urine deposited by the bug during or after blood ingestion, but only this trypomastigotes stage can initiate an infection in the mammalian host. There it is phagocytized and transforms to amastigotes, which multiply and finally transform to non-dividing blood trypomastigotes. After rupturing of the exhausted host cell, they infect new cells or circulate in the blood (summarized by Schaub et al. 2011).
12.6 Effects of the Triatomines on Trypanosoma cruzi
In the field, the infection rates of even neighboured populations of triatomines vary strongly, indicating refractoriness mechanisms in the vector (summarized by Garcia et al. 2010). Thus, after the transport of a strain of the flagellate to another region, not only the mammalian hosts but also triatomines can act as “filters” and loose the respective strain at least during prolonged starvation (summarized by Schaub and Lösch 1989). However, susceptibilities also differ between individuals of a population, indicated by the high standard deviations in the number of intestinal flagellates after a standardized experimental infection in an optimal parasite–vector system in which both components originated from the same village (Schaub 1989b). In this system, even after eight generations of selection for differences in susceptibility, i.e. separate breeding of bugs which possessed low or high numbers of parasites in the rectum, all bugs became infected, but the “less susceptible” strain contained less parasites (Schaub unpublished). Unfortunately, these strains of T. infestans were killed by a failure of the incubator and its security system. Thus, the search for factors responsible for the differences in the susceptibilities could not be performed.
Of the different compounds in the saliva of triatomines, only trialysin lysed cell culture-derived trypomastigotes and epimastigotes (summarized by Schaub et al. 2011), but an effect on the initial development in the vector remains to be investigated. The initial establishment of specific strains is determined by agglutinins and hemolysins (summarized by Schaub et al. 2011). However, in these infections, epimastigotes were used, mimicking only the less important infection by coprophagy. Intestinal proteases seem to have no effects on T. cruzi, but knock-down studies are required.
Strong effects on T. cruzi are induced by starvation and feeding (summarized by Schaub 2009). During starvation, many intestinal flagellates die, and in the remaining population, the percentages of spheromastigotes and the respective intermediate forms increase from 2 to about 20%. Feeding of long-term starved bugs induces the development of giant cells, containing many nuclei, flagella and kinetoplasts. However, between 5 and 10 days after feeding, these cells disappear completely. Feeding of short-term starved bugs induces metacyclogenesis. Whereas the development of spheromastigotes and giant cells could not be correlated with specific factors in the gut, the rapid induction of metacyclogenesis could be correlated with proteins in the urine of the vector. An induction requiring a longer period of time was caused by a fragment of chicken αD-globin and free fatty acids (summarized by Schaub et al. 2011).
12.7 Effects of Trypanosoma cruzi on Triatomines
T. cruzi only slightly affects the vector (summarized by Schaub 1992, 2009). Under optimal conditions, i.e. the mortality rate of control groups is <10%, the nymphal development and mortality rates are not affected by the infection. However, the competition of flagellate and vector for food components is indicated by a reduced starvation capacity (Schaub and Lösch 1989). Therefore, T. cruzi is classified as “subpathogenic” (Schaub 1989a, 1992). Slight effects are known for the probing behaviour, but the increased probing in infected bugs might also be caused by the competition (Schaub et al. 2011). In electron microscopy, the wax layer of the rectal cuticle is damaged in infected bugs, but the proof of an effect on the absorption processes requires precise measurements. The changes in concentrations of rectal free and protein/peptide bound amino acids (Kollien and Schaub unpublished) might be caused by cysteine proteases of the flagellate. However, in the stomach, the gene of a cysteine protease inhibitor—acting against cruzain (cruzipain) of T. cruzi—is expressed by T. infestans after an infection (Buarque et al. 2011). The activity of an aspartate protease, cathepsin D, is increased in the small intestine at 1 and 3 days after an experimental infection with epimastigotes (Borges et al. 2006). However, this protease might be involved not only in the digestion of food but also in intestinal immune responses (Balczun et al. 2012). Ingestion of trypomastigotes stimulates the immune reactions in the intestine. In R. prolixus, it induces the expression of the gene of the more intestinal active lysozyme RpLys-A, but not of the RpLys-B gene, which is primarily expressed in the fat body (Ursic-Bedoya et al. 2008). In T. brasiliensis, after infection, more mRNA encoding defensins are produced (Waniek et al. 2011). Besides these antimicrobial peptides, another antimicrobial factor, nitric oxide, is also synthesized in the intestine after an infection (Whitten et al. 2007). Since the expression rates of the genes of these compounds are also increased after blood ingestion (Araújo et al. 2006; Whitten et al. 2007), these short-term reactions to T. cruzi can partly be a general defence reaction against microorganisms. In long-term infected T. infestans, T. cruzi suppresses the intestinal immunity in the intestine, enabling the development of different microorganisms (summarized by Schaub et al. 2011).
12.8 Future Works
There are several aspects that need to be considered in long-term investigations to understand the co-evolution and the interactions of T. cruzi and triatomines. Investigations of the first topic are complicated by the human migration and the introduction of specific DTUs of T. cruzi in regions in which these DTUs had not been present previously. Therefore, many strains originating from mammals and vectors have to be typed to get indications for the original situation. Then detailed investigations of the development in the vector including the metacyclogenesis rates can be performed. Investigations of the interactions of T. cruzi and triatomines will be strongly be supported by the genome project of R. prolixus and large-scale applications of the RNAi technique to identify factors which are responsible for the different susceptibility of species and individuals of triatomines. These investigations with systems in which vector and parasite should originate from the same locality should be performed not only with R. prolixus, T. brasiliensis and T. infestans but also with triatomines engaged in the sylvatic cycle of T. cruzi. Thereby, factors inducing the initial killing of some strains of T. cruzi in the stomach and the molecular basis of the intestinal immune response and the metacyclogenesis in the rectum of the vector can be identified.
Acknowledgements
We are deeply grateful for the cooperation with many colleagues—recognizable by co-authorships—and the funding of the investigations and travels by the UNDP/World Bank/WHO Special Programme for Research and Training in Tropical Diseases, the VolkswagenStiftung, the German Academic Exchange Service (DAAD), European Community programmes, the Humboldt Foundation and especially the Deutsche Forschungsgemeinschaft.
References
Abad-Franch F, Monteiro FA (2005) Molecular research and the control of Chagas disease vectors. An Acad Bras Ciênc 77:437–454PubMed
Amino R, Porto RM, Chammas R, Egami MI, Schenkman S (1998) Identification and characterization of a sialidase released by the salivary gland of the hematophagous insect Triatoma infestans. J Biol Chem 273:24575–24582PubMed
Amino R, Tanaka AS, Schenkman S (2001) Triapsin, an unusual activatable serine protease from the saliva of the hematophagous vector of Chagas’ disease Triatoma infestans (Hemiptera: Reduviidae). Insect Biochem Mol Biol 31:465–472PubMed
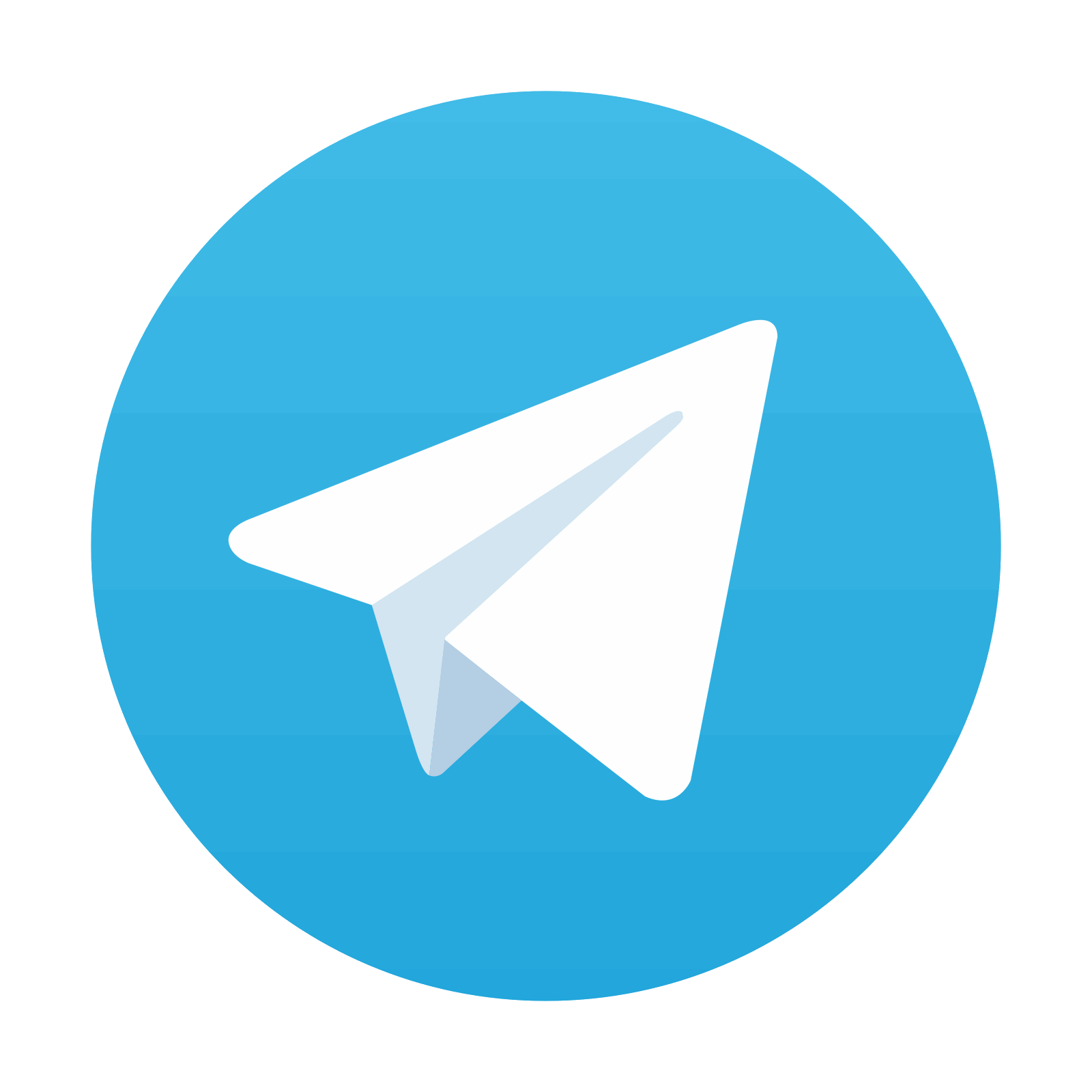
Stay updated, free articles. Join our Telegram channel

Full access? Get Clinical Tree
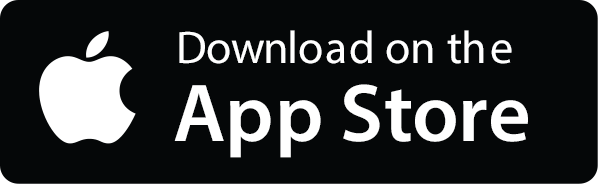
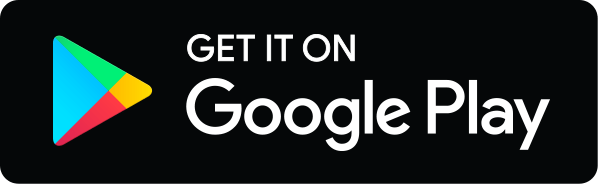