Category
Determinants of outcome
Patient (host)
variables
• Age
• Intelligence
• Comorbidities: substance abuse, neurologic disorders, psychiatric disorders
• Genotype
• Resilience
Injury variables
• Severity and duration of impaired consciousness
• Pupillary response to light
• Mass lesion and location (especially penetrating missile)
• Intracranial pressure
• Complications: hypotension, hypoxia, post-traumatic epilepsy
• Other injuries such as limb fractures
Environment
• Medications and associated neurotoxicity
• Rehabilitation and neuroplasticity
• Social support and environmental enrichment
5 Assessment of Outcome of TBI
An interagency Common Data Elements Traumatic Brain Injury Outcomes Workgroup (19) identified domains of outcome, including recovery of consciousness, global level of function, neuropsychological impairment, psychological (emotional) status and post-concussion symptoms, performance of everyday activities, social role participation, and perceived health-related quality of life. Within each domain, the Workgroup distinguished core measures that have been well validated for general application to the adult TBI population and shown to be sensitive to change over time. Specialty measures recommended for use with specific populations or to address specific research questions were identified for each domain as were future measures that are under investigation. Table 10.2 provides a summary of the outcome measures recommended by the TBI Outcomes Workgroup. Under neuropsychological impairment, the subdomains including episodic memory, attention/processing speed, and executive function encompass the most frequent impairments reported in patients following TBI due to closed head trauma. Although quantitative neuropsychological tests are widely used to measure the subdomains noted in Table 10.2, problems in executive function and memory may also impact performance of daily activities such as planning, monitoring performance, and flexibility in switching tasks or dividing one’s attention between tasks.
Outcome | Core measure(s) | Specialty measures |
---|---|---|
Global outcome | ||
Recovery of consciousness | • JFK Coma Recovery Scale-Revised (CRS-R) (91) | |
Neuropsychological impairment | ||
Episodic memory | • Rey Auditory Verbal Learning Test (RAVLT) (92) | • Brief Visuospatial Memory Test-Revised (BVMT-R) (93) |
Attention/processing speed | • Processing Speed Index from the WAIS-III (94) | • Digit Span subtest of the Wechsler Adult Intelligence Scale (WAIS-III) (94) |
Executive function | • Trail Making Test (TMT) (95) | |
Motor speed | • Grooved pegboard test (96) | |
• † NIH toolbox cognitive battery | ||
Psychological status | • Brief Symptom Inventory-18 Item (BSI-18) (100) | • Minnesota Multiphasic Personality Inventory-2-Restructured Form (MMPI-2-RF) (101) • Alcohol Use Disorders Identification Test (AUDIT) (102–104) • Substance Use Questions from the TBI Model Systems Dataset (105, 106) • Alcohol, Smoking, and Substance Use Involvement Screening Test (ASSIST) (107, 108) • PTSD Checklist – Civilian/Military/ Stressor Specific (PCL – C/M/S) (109, 110) • The Family Assessment Device (FAD) (111, 112) |
• † NIH toolbox emotional battery | ||
TBI-related symptoms | • Neurobehavioral Symptom Inventory (NS) (115) | |
Behavioral function | ||
Cognitive activity limitations | • Functional Independence Measure-Cognition Sub scale (Cog-FIM) | |
Physical function | ||
• † NIH Toolbox Motor and Sensory Batteries • † Neurological Outcome Scale for Traumatic Brain Injury (NOS-TBI). | ||
Social role participation | ||
• † ParticipationAssessmentwith Recombined Tools (PART) | ||
Perceivedgeneric and disease-specific health-related quality of life | ||
Health-economic measures | ||
Patient reported outcomes(future multidimensional tools) | ||
Psychological (emotional) status and behavior: This domain of outcome is also sensitive to prefrontal–temporal injury including effects of TBI on the orbitofrontal–amygdala system. Major depression, disinhibition, and irritability are frequent behavioral sequelae of TBI associated with closed head trauma. These behavioral sequelae may also result from penetrating missile wounds of the brain, depending on the neuroanatomic site of injury. Depression and anxiety may persist despite good cognitive recovery following mild TBI (20). In addition to self-report measures, scales completed by a caregiver and rating forms completed by an examiner based on a structured interview are informative. Behavioral sequelae been implicated in residual problems in social functioning, including loss of friends and loneliness. Families have reported that the behavioral effects of severe TBI constitute the greatest burden on caregivers (21), but also see Temkin et al. (22).
Challenges to researchers investigating outcome: The diversity and number of relevant outcome measures are often a challenge for examiners to implement. First, patients tend to fatigue easily during the early post-injury phase thus limiting their capacity to complete a lengthy examination. Catastrophic TBI may also result in cognitive deficits that limit the patient’s capability of comprehending and completing more difficult neuropsychological tests, thus resulting in selection bias.
Assessment of global outcome and functional recovery depends on integration of sources of information, raising a question of interexaminer reliability and sufficient training of the examiners. Multicultural issues must also be addressed, including the assessment of individuals whose primary language is not English. Analysis of multiple outcome measures raises questions about spurious results, an issue which can be potentially mitigated by developing composite measures. Models of outcome that emphasize participation in activities within the family, community, and at work have drawn a distinction between impairment identified by a neuropsychological test and disability which reflects curtailment of participation.
Heterogeneity in outcomes: In general, cognitive and behavioral sequelae contribute more to disability following TBI than motor or other specific neurologic deficits (23). Cognitive deficits at least partially resolve during the first 6–12 months after moderate-to-severe TBI, whereas cognitive recovery is generally complete within 1–3 months following mild TBI. However, impaired episodic memory on tests of recalling word lists or spatial location of items persists following moderate-to-severe TBI in about 20–25% of adults whose other cognitive functions recover relatively well during the first year post-injury (24). This dissociation may be attributed to hippocampal damage, but recent studies using diffusion tensor imaging (DTI) have shown that compromise of the microstructure of white matter connections including prefrontal and temporal white matter and corpus callosum is related to the cognitive sequelae of TBI (25, 26).
However, there is heterogeneity in outcomes and the most salient deficits among patients sustaining moderate-to-severe TBI. A patient whose TBI is complicated by extensive focal injury in the posterior region of the right hemisphere may be disabled by visuospatial neglect despite otherwise good recovery of cognition whereas other patients’ functional recovery may be limited by language deficits though these severe, specific deficits persist in a relatively small subgroup of recovering TBI patients whose injury mechanism was closed head trauma.
Heterogeneity seems to be one of the fundamental barriers to successful therapeutic trials in TBI. A clear disconnect exists between preclinical research and the lack of treatment effects in clinical trials (27). In the search for neuroprotective therapeutics, animal models play an important role in elucidating the molecular and cellular mechanisms underlying secondary pathogenesis. While individual models of TBI may lack the heterogeneity seen in human TBI, the diversity of models provides a means for validating robust neuroprotection that is not constrained to a single model. In the following sections, we review the most common rodent models of TBI with special emphasis on those behavioral metrics that are most closely aligned to human TBI.
6 Animal Models of TBI: Overview
A number of tests have been developed to profile emerging deficits and/or recovery after experimental TBI. Behavioral assays, conducted at varying times post-injury, provide an essential background for understanding the underlying relationships between pathogenesis, wound healing, and clinical measures of recovery. Broadly, these tests fall into three categories: sensorimotor, cognitive, and affective behaviors. In the following sections, we review these measures of function in the context of experimental models of TBI in the rodent. We limit this review to rodent models, the species that have been most studied in experimental models of TBI, and to the most common models of TBI; namely, fluid percussion injury, controlled cortical impact, impact acceleration injury, and closed head injury (Table 10.3). Readers are referred to recent reviews that describe these models in detail (28, 29–31). While the unique characteristics of these models may impart behavioral “signatures,” they also produce some similar findings across different models. In these sections, we highlight both the differences and similarities in behavioral profiles across the different models of TBI. In general, comparisons described in this review are against sham-injured animals.
Experimental model | Characteristic pathologic features |
---|---|
Fluid percussion injury | • Focal cortical contusion • Intraparenchymal hemorrhage • Marked diffuse axonal injury • Widespread cortical and subcortical damage |
Controlled cortical impact | • Focal cortical contusion • Intraparenchymal hemorrhage • Some diffuse axonal injury • Widespread cortical and subcortical damage |
Impact acceleration injury | • Absence of focal lesions • Mild subarachnoid hemorrhage • Marked diffuse axonal injury • Cortical damage directly beneath impact site and in hippocampus |
Closed head injury | • Focal cortical contusion • Intraparenchymal hemorrhage • Some diffuse axonal injury • Increased possibility of skull fracture |
Early determinants of outcome (Table 10.4): With this focus on rodent models, we acknowledge differences that exist between species and strains (32, 33). Such differences may influence how the brain responds to injury and the profile of behavioral recovery. Thus, we present findings in the context of both the type of injury, and when available, the species/strain studied.
Determinants | Comments |
---|---|
Location of injury | • Midline brain injuries have a greater brain stem component than lateral injuries. Midline injury leads to greater asymmetrical dysfunction than anterior or posterior injuries |
Severity of initial insult | • Mild, moderate, or severe injury yields graded effects on the behavioral sequelae |
Gender | • Sensorimotor deficits after TBI are greater in males compared to females |
Species and strain | • Rats and mice have different responses to injury, with mice showing less severe injury • C57BL/6 are more sensitive to injury on the beam walking task, compared to FVB/N or 129/SvEMS. The latter strains are also unable to learn the Morris water maze or Barnes circular maze tasks |
Age at time of injury | • The developing brain is sensitive to age at time of injury • Both the immature and the aging brain show enhanced response to injury |
Beyond differences related to species/strain, a number of early determinants of behavioral outcomes have been identified (Table 10.4), paralleling the determinants of outcome following human TBI. These include type of injury, location and severity of the initial insult, gender, and age at time of injury. The pathophysiology of TBI occurs over time and in a pattern consistent with the biomechanics of the initial impact. Acceleration/deceleration, focal, and diffuse models of injury have differing but well-defined cascades of temporal progression and lead to specific patterns of cellular and neurophysiological damage (34). Moreover, the biomechanics of injury are differentially impacted by location and severity of injury, leading to varying outcome (35). While animal models replicate different aspects of human TBI, the choice of experimental model depends on the underlying objectives of a study (28, 30) and an understanding of the relationship between the model and the emerging behavioral phenotype.
Gender is also a determinant of the behavioral phenotype. Experimental models of TBI have historically focused on males. However, over the past decade it has become increasingly clear that gender is a determinant of performance in behavioral tasks (36, 37) including those that assess working, reference, and spatial memory (38, 39). The underlying differences between genders may at least in part reflect diverging pathophysiology and behavior that show a differential sensitivity to a given therapeutic. For example, sensorimotor deficits, measured by foot faults in the grid walk test, are significantly greater in male C57Bl/6 mice compared to females, 1 week following a controlled cortical impact, while spatial learning deficits are similar between genders (40). While human studies have noted a gender difference in the epidemiology of TBI (2), the differences in injury response and behavioral phenotype due to gender are not well understood.
Age at the time of injury also influences outcome. For example, trauma to the developing brain modifies subsequent maturation and interferes with the acquisition of age-appropriate skills (41). The developing, immature brain is uniquely vulnerable to injury. Moreover, the enhanced post-injury plasticity attributed to the developing brain limits normal developmental neuroplasticity, especially at selective time periods after injury (42). At the opposite end of the temporal spectrum, TBI to the aging rodent brain results in a greater loss of function compared to the injured adult brain. For example, when adult and aging rodents are subjected to controlled cortical impact, the latter shows more pronounced early edema, neurodegeneration, and functional deficits (43). These findings suggest that both early and prolonged secondary pathogenesis in the aging brain contribute to poorer behavioral outcomes.
7 Experimental TBI: Behavioral Assays
Various behavioral tests have been used to assay specific components of neurological dysfunction following TBI. Here we summarize these findings according to sensorimotor, cognitive, and affective behaviors.
Sensorimotor behavior (Table 10.5): Motor function is integrated and mediated by a complex network of nuclei of the vestibulomotor pathway (44). Motor deficits, arising from TBI, may in part reflect damage to this pathway. A number of tests measure the ability of the brain-injured animal to locomote and maintain balance. Few of these tasks, however, purely test motor behavior. Each has a component of sensory feedback and proprioception, and therefore, most tasks are considered to be sensorimotor in nature.
Behavioral measures | Species/strain | Type of injury |
---|---|---|
Aggregate tests | ||
Composite neuroscore | Rats/Sprague Dawley | Fluid percussion injury |
Mice/C57Bl/6 | Controlled cortical impact | |
Neurologic severity score | Rats/Sprague Dawley | Closed head injury |
Mice/Sabra | Impact acceleration injury | |
SNAP assessment | Mice/C57Bl/6 | Controlled cortical impact |
Individual tests | ||
Rotarod | Rats/Sprague Dawley | Controlled cortical impact |
Rats/Sprague Dawley | Fluid percussion injury | |
Rats/Sprague Dawley | Impact acceleration injury | |
Mice/C57Bl/6, FVB/N, 129/SvEMS | Controlled cortical impact | |
Grid walk | Mice/C57Bl/6 | Controlled cortical impact |
Spontaneous forelimb use | Mice/C57Bl/6 | Controlled cortical impact |
Wire hang | Mice/CF-1 | Closed head injury |
Beam balance | Rats/Sprague Dawley | Fluid percussion injury |
Rats/Sprague Dawley | Controlled cortical impact | |
Mice/C57Bl/6 | Controlled cortical impact | |
Beam walk | Rats/Sprague Dawley | Fluid percussion injury |
Rats/Sprague Dawley | Controlled cortical impact | |
Adhesive tape removal | Rats/Sprague Dawley | Fluid percussion injury |
One approach to profiling motor/sensory function is the use of aggregate tests that incorporate different measures of function. In the context of TBI, aggregate tests include the composite neuroscore, the neurological severity score (NSS), and the Simple Neuroassessment of Asymmetric imPairment (SNAP).
The composite neuroscore includes tests of flexion of forelimb and hindlimb, walking ability in a line or in a circle, abnormal movements, proprioception, as well as reflexes. This test was first described in rats and then modified for mice (45). Animals are scored on a 4 (normal) to 0 (severely impaired) scale using each of the following indices: (a) forelimb flexion, (b) resistance to lateral pulsion, (c) circling behavior in spontaneous ambulation, (d) ability to stand on an inclined plane, and (e) open-field activity. A total composite functional neuroscore of 0–20 is determined by combining the scores for the various tests so that 20 = normal, 15 = slightly impaired, 10 = moderately impaired, 5 = severely impaired, and 0 = non-functional. Fluid percussion injury in Sprague Dawley rats produces a chronic neurological deficit lasting up to 4 weeks that reflects to the severity of the initial injury (46). A modified composite neuroscore, ranging from 0 (non-functional) to 12 (normal), has been described for mice (C57Bl/6) and shown to predict marked behavioral impairments (scores of 9) when animals are subjected to a controlled cortical impact (45).
The NSS is another example of an aggregate measure (Table 10.6). Animals are scored on an all or none scale on multiple tests, with the overall NSS score being the sum of these tests. Individual tests include limb flexion, head movement, ability to walk straight, abnormal movements such as immobility, tremor, and myodystony, sensory tests, and tests of reflexes (47). Sprague Dawley rats with a closed head injury and evaluated on a scale of 1–24 show a decrease in the score over 30 days (48). The NSS likewise reveals deficits in murine (Sabra mice) models of TBI including impact acceleration injury that persist for at least 30 days post-injury (49).
Table 10.6
Measurement of neurological severity score. For details, refer to (48). One point is given for failure to perform a task, thus, a higher score indicates a more severe injury. The animals are evaluated at 1 h and again at predetermined time points between 1 and 30 days. The difference between the new score and the 1 h score, ΔNSS, defines the extent of recovery of the tested animal
Evaluation (time after injury) | ||
---|---|---|
Neurological severity score | 1 h | >1 h |
Inability to exit from a circle (50 cm in diameter) when left in its center | ||
For 30 min after injury | 1 | – |
For 60 min after injury | 1 | – |
> 60 min after injury | 1 | – |
Loss of righting reflex | ||
For 20 min after injury | 1 | – |
For 40 min after injury | 1 | – |
> 60 min after injury | 1 | 1 |
Hemiplegia – inability to resist forced changes in position | 1 | 1 |
Flexion of hindlimb when raised by the tail | 1 | 1 |
Inability to walk straight when placed on the floor | 1 | 1 |
Reflexes | ||
Pinna reflex | 1 | 1 |
Corneal reflex | 1 | 1 |
Startle reflex | 1 | 1 |
Clinical grade | ||
Loss of seeking behavior | 1 | 1 |
Prostration | 1 | 1 |
Limb reflexes | ||
Loss of placing reflexes | ||
Forelimbs left/right | 1 | 1 |
Hindlimbs left | 1 | 1 |
Hindlimbs right | 1 | 1 |
Functional test | ||
Failure in beam balancing task (1.5 cm wide) | ||
For 20 s | 1 | 1 |
For 40 s | 1 | 1 |
For >60 s | 1 | 1 |
Failure in beam walking task | ||
8.5 cm wide | 1 | 1 |
5 cm wide | 1 | 1 |
2.5 cm wide | 1 | 1 |
Maximum points | 24 | 20 |
The aggregate scoring test known as the SNAP test has recently been developed to evaluate the relationship between magnitude of injury and functional recovery in a murine model of controlled cortical impact (50). This test incorporates measures of vision, proprioception, motor strength, and posture, and the SNAP score reflects a summation of these assessments. The SNAP is based on a scale from 0 to 25, with a neurologically intact animal expected to have a score of 0. C57Bl/6 mice, when subjected to a moderate controlled cortical impact, show scores of about 8.4 at 3 days post-injury and persistent deficits (SNAP score = 6.1) at 12–14 months post-injury.
While aggregate tests provide an overall behavioral profile, individual tests offer insight into sensorimotor functions that may be affected by the injury. These include assessments of performance on a rotarod, ability to locomote across a wire grid, spontaneous forelimb use, beam balance, beam walk, and adhesive removal test. The rotarod test, in particular, is a very sensitive and efficient index for assessing sensorimotor impairments produced by TBI (51). This test assays the ability of the animal to maintain balance on a rotating rod by measuring latency to end of a trial (falling off the rotarod or gripping the device for two consecutive revolutions). Test paradigms include different rotation speeds and accelerating rotation. Mice (C57Bl/6, FVB/N, 129/SvEMS), subjected to a controlled cortical impact, show deficits in performance up to 7 days following a moderate insult (52) whereas Sprague Dawley rats, subjected to a similar injury, show deficits in the rotarod test for several weeks (53).
The grid walk test allows for the independent assessment of all four limbs. Animals locomote on an elevated grid surface, and the percentage of stepping errors (foot faults) is calculated. The asymmetry of foot faults is measured by the difference between ipsilateral and contralateral foot faults. Injury results in location-dependent contralateral forelimb deficits. Increased contralateral foot faults are evident up to 4 weeks following controlled cortical impact in C57Bl/6 mice (54).
The spontaneous forelimb-use task allows the exclusive evaluation of forelimb function. It assays function of the forelimbs in vertical wall exploration (54). When placed in a Plexiglass cylinder, the animal rears to a standing position on the cylinder wall, supporting its weight with either one or both of its front limbs. The forelimb preference during vertical exploratory movements has been quantitatively evaluated by measuring the time spent supporting its weight on each foot. C57Bl/6 mice, sustaining a controlled cortical impact, show asymmetry in their forelimb use, with the ipsilateral paw being favored. This task is also sensitive to the location of the injury. Animals with midline TBI show greater asymmetry than animals with anterior or posterior injuries. These deficits may persist up to 5 months after injury (54).
The wire hang task is typically assayed in murine models of TBI. The latency of the animal to hang on a taut string is measured up to a maximum of 60 s. Decreased wire hang scores are reported acutely after closed head injury or impact acceleration injury in CF-1 mice (47, 55).
The beam balance or beam walk tasks assess the more complex components of vestibulomotor function and coordination. The beam balance task has been studied in brain-injured rats and mice. The animal is placed on a narrow wooden beam and its ability to maintain equilibrium is scored from 1 to 5 based on predetermined criteria. Based upon early studies, C57Bl/6 mice, subjected to controlled cortical impact, are impaired in their performance on the beam balance by 48 h post-injury but recover over 3 weeks (56). Sprague Dawley rats show beam balance deficits early after moderate fluid percussion or controlled cortical impact, which are then resolved by about 10 days post-injury (57, 58). In the beam walk task, animals are trained to escape a bright light and loud white noise by traversing an elevated narrow wooden beam to enter a darkened goal box at the opposite end of the beam. Performance is measured by the animal’s latency to traverse the beam. Sprague Dawley rats show increased latency in traversing the beam in the first 5 days following lateral fluid percussion injury (57, 59) or controlled cortical impact (58).
< div class='tao-gold-member'>
Only gold members can continue reading. Log In or Register a > to continue
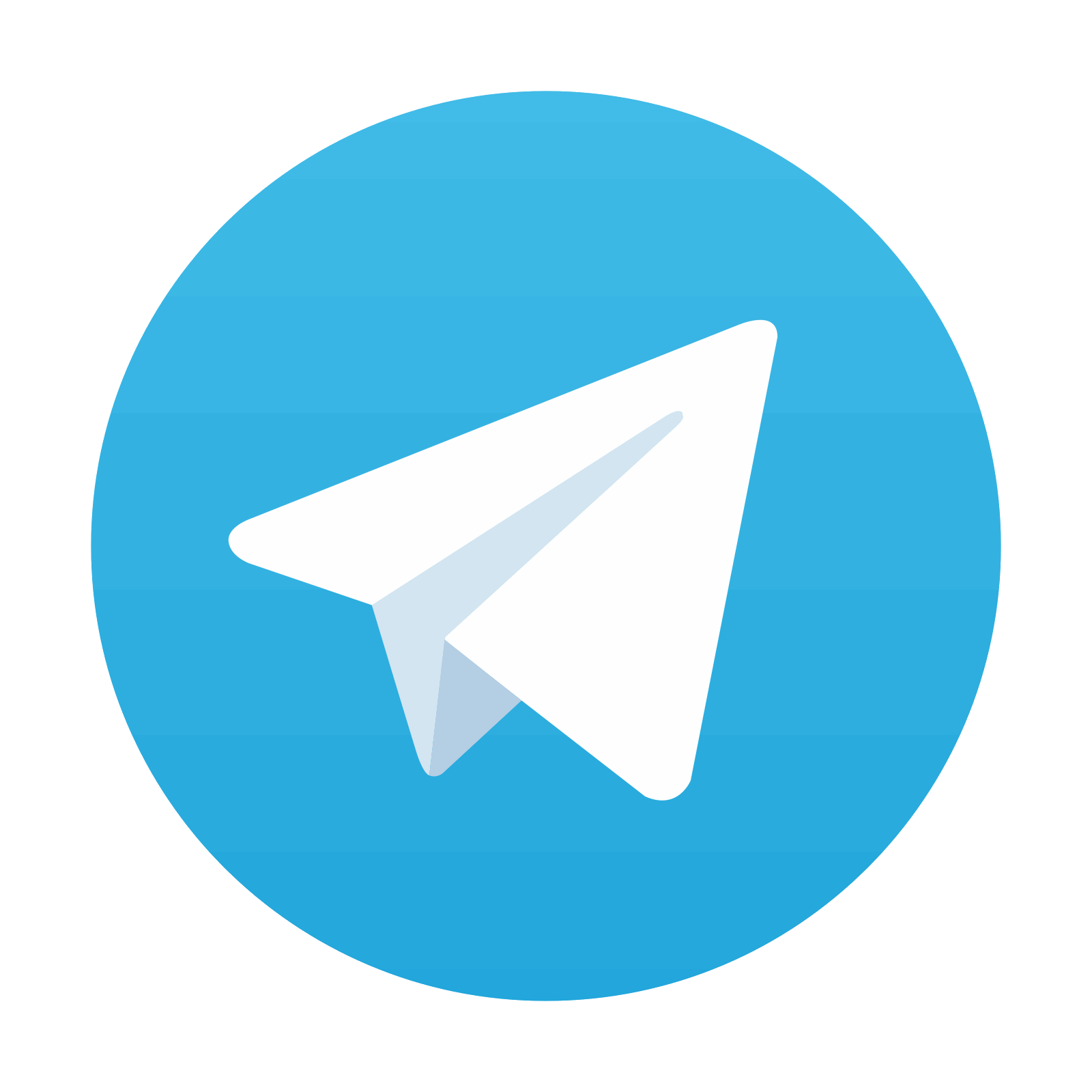
Stay updated, free articles. Join our Telegram channel

Full access? Get Clinical Tree
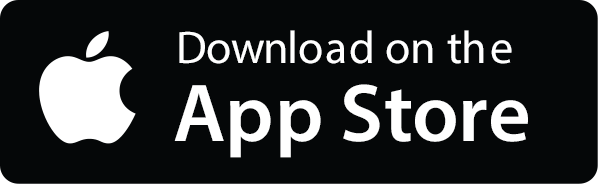
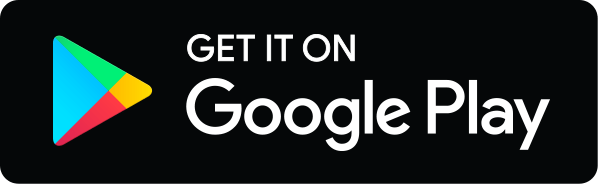