Chapter 2 Toxicokinetics and Toxicodynamics
DEFINITIONS
The basic concepts regarding the toxicokinetics and toxicodynamics of xenobiotics are clinically relevant to veterinary toxicology and need to be understood by veterinary practitioners, professional students, and other personnel who will be participating in the diagnosis and treatment of small animal intoxications. In discussing the aspects of toxicokinetics and toxicodynamics most pertinent to small animal toxicoses, it is first necessary to define several terms. “Xenobiotic” is a general term referring to any chemical foreign to an organism or, in other words, any compound not occurring within the normal metabolic pathways of a biological system.1,2 Depending on the compound and the level of exposure, interactions between xenobiotics and animals can be benign, therapeutic, or toxic in nature. The pharmacokinetics and pharmacodynamics of a therapeutic xenobiotic influence the time course and efficacy of that compound in a pharmacological setting. Likewise, the toxicokinetics and toxicodynamics of a toxic xenobiotic determine the “when,” “how long,” “what,” and “why” for the adverse effects of that toxicant.2
The “disposition” of a xenobiotic is what the animal’s body does to that compound following exposure. The disposition or fate of a xenobiotic within the body consists of the chemical’s absorption, distribution, metabolism (biotransformation), and excretion characteristics, which are collectively abbreviated as ADME.2,3 “Toxicokinetics” refers to the quantitation and determination of the time course of the disposition or ADME for a given toxic xenobiotic.3 There are a variety of specialized toxicokinetic terms, including bioavailability, volume of distribution, clearance, half-life, one-compartment model and first- and zero-order kinetics, which will be discussed later in this chapter under the separate components of ADME.
The term “toxicodynamics” describes what a toxicant does physiologically, biochemically, and molecularly to an animal’s body following exposure. The toxicodynamics of a given toxic xenobiotic are dependent on the mechanism of action of that toxicant and the relationship between toxicant concentration and the observed effects of the toxicant on biological processes in the animal (i.e., the dose-response relationship).1 The disposition and/or toxicokinetics of a particular xenobiotic also play a role in determining the organs or tissues affected by a toxicant, and the clinical presentation and time course of a toxicosis resulting from excessive exposure to that compound.1,2
TOXICOKINETICS/DISPOSITION
Xenobiotic absorption
With the exception of caustic and corrosive toxicants that cause adverse effects at the site of exposure, a toxic xenobiotic is generally first “absorbed” or taken up into the body.3 Absorption involves crossing cellular membranes, which are typically composed of phospholipid bilayers containing various sized pores and embedded proteins.2 The route of exposure and physiochemical properties of a toxicant, such as its resemblance to endogenous compounds, its molecular size and relative lipid and water solubilities, the magnitude of a molecule’s association constant, and whether a compound can be classified as a weak acid or as a weak base, all determine the manner and quantities in which a xenobiotic is absorbed across cell membranes.
Routes of xenobiotic exposure and xenobiotic bioavailability
The most common routes of exposure for xenobiotics in small animal toxicology are oral (gastrointestinal), dermal (percutaneous), and inhalation (pulmonary). In rare instances of iatrogenic intoxications, xenobiotics can be injected subcutaneously, intramuscularly, intraperitoneally, or even intravenously.3 There are unique aspects to the absorption of xenobiotics associated with each route of exposure, especially with regard to the bioavailability of potential toxicants.
“Bioavailability” (often represented by “F” in toxicokinetic equations) represents the fraction of the total dose of a toxic xenobiotic that is actually absorbed by an animal.2 In intravenous exposures, the bioavailability of a toxic xenobiotic is 100% since the entire dose of the toxicant reaches the peripheral circulation. The absorption of gases and vapors in the respiratory tract is largely dependent on the ratio (blood-to-gas partition coefficient) between the equilibrium concentrations of the toxicant dissolved in the blood and the gaseous phase of the toxicant in the alveolar spaces.2,3 The size of aerosolized particles will determine to a large degree whether a xenobiotic is deposited in the nasopharyngeal region (particles > 5 μm) or within the alveoli of the lungs (<1 μm).2 The stratum corneum and its associated keratinized structures often impede the percutaneous absorption of xenobiotics, and there are variations in the absorptive ability of skin in different anatomical locations.4 Dermal absorption is frequently dependent on the vehicle in which a toxicant is dissolved and is generally greater for lipid soluble compounds as compared with chemicals that are highly soluble in water.2–4 The bioavailability of toxic xenobiotics that are ingested can be negatively impacted by acidic degradation in the stomach and/or enzymatic breakdown in the small intestine.2 Decreased gastrointestinal transit time can diminish xenobiotic bioavailability by limiting the access of toxicants to those regions of the digestive tract where rates of absorption are greatest. Some potential toxicants, especially certain heavy metals (e.g., lead and cadmium), resemble essential minerals such as calcium and zinc, respectively. The gastrointestinal absorption of these toxic nonessential metals involves interactions with dietary levels of the corresponding essential metals and regulated mechanisms of gastrointestinal uptake designed for these required minerals.
Hepatic biotransformation of xenobiotics, which will be discussed in greater detail later in this chapter, can also influence the apparent bioavailability of ingested toxicants. Following oral exposure, xenobiotics absorbed from the gastrointestinal tract are transported to the liver via the hepatic portal circulation. For some xenobiotics, rapid hepatic degradation (and in some instances prior biotransformation in gastrointestinal cells) prevents access of the compound to the systemic circulation, resulting in an apparently decreased bioavailability from what is termed the “first-pass effect” or “presystemic elimination.”3,4 In contrast, the bioavailability of some chemicals is enhanced by a cycle of biliary excretion and subsequent reuptake from the intestines referred to as “enterohepatic recirculation.”4
Mechanisms of xenobiotic absorption
The passage of xenobiotics through cellular membranes can be either energy-independent (“passive” transport) or can require the expenditure of energy through “specialized” or “active” transport systems. Passive transport of xenobiotics can be accomplished through simple diffusion or filtration. Specialized, energy-dependent, cellular transport systems include the process specifically referred to as “active transport,” along with facilitated transport and pinocytosis.2,3
Passive transport of xenobiotics
Simple diffusion and filtration are nonsaturable processes, which do not require the expenditure of energy to transport xenobiotics across cellular membranes.2,3 Both of these mechanisms of passive transport are dependent on the concentration gradient for a given xenobiotic, with the rate of transport being proportional to the difference in that chemical’s concentration between the two sides of a particular membrane (Fick’s law).2 Simple diffusion is the most common mechanism by which xenobiotics cross cellular membranes. Uncharged (nonionized), lipid-soluble molecules, especially those which are small, are more readily diffusible across the phospholipid bilayers of biological membranes than charged (ionized) molecules, which are generally less lipid-soluble.2,3 The Henderson-Hasselbalch equation can be used to predict whether a particular xenobiotic will be in the nonionized or ionized state in a particular biological matrix. In this equation, the difference between the association constant (pKa), which is equivalent to the pH at which equal amounts of a xenobiotic are in the nonionized and ionized states, and the pH of the biological matrix in which the xenobiotic will exist (i.e., pKa − pH) is equal to the common log of the quotient of nonionized xenobiotic divided by ionized xenobiotic for weak acids and the log of the reciprocal quotient (ionized xenobiotic divided by nonionized xenobiotic) for weak bases.2–4 Filtration involves the passage of xenobiotics through patencies or pores within cellular membranes and is determined, in large part, by the size of the xenobiotic molecule and pore-size, which varies in different organs and tissues.2
Specialized transport of xenobiotics
Active transport is an energy-dependent, saturable process by which xenobiotics are transported across biological membranes against electrochemical or concentration gradients.2–4 Specific examples of active transport systems include the multidrug-resistant proteins (P-glycoproteins) and members of the organic cation transporter family.3 Facilitated or carrier-mediated transport can require the expenditure of energy, but, in contrast to active transport, xenobiotic transport by this mechanism is not against a concentration gradient.2,3 Pinocytotic transport involves cellular engulfment of small amounts of xenobiotic and the transfer of this amount of chemical through the cellular membrane.2
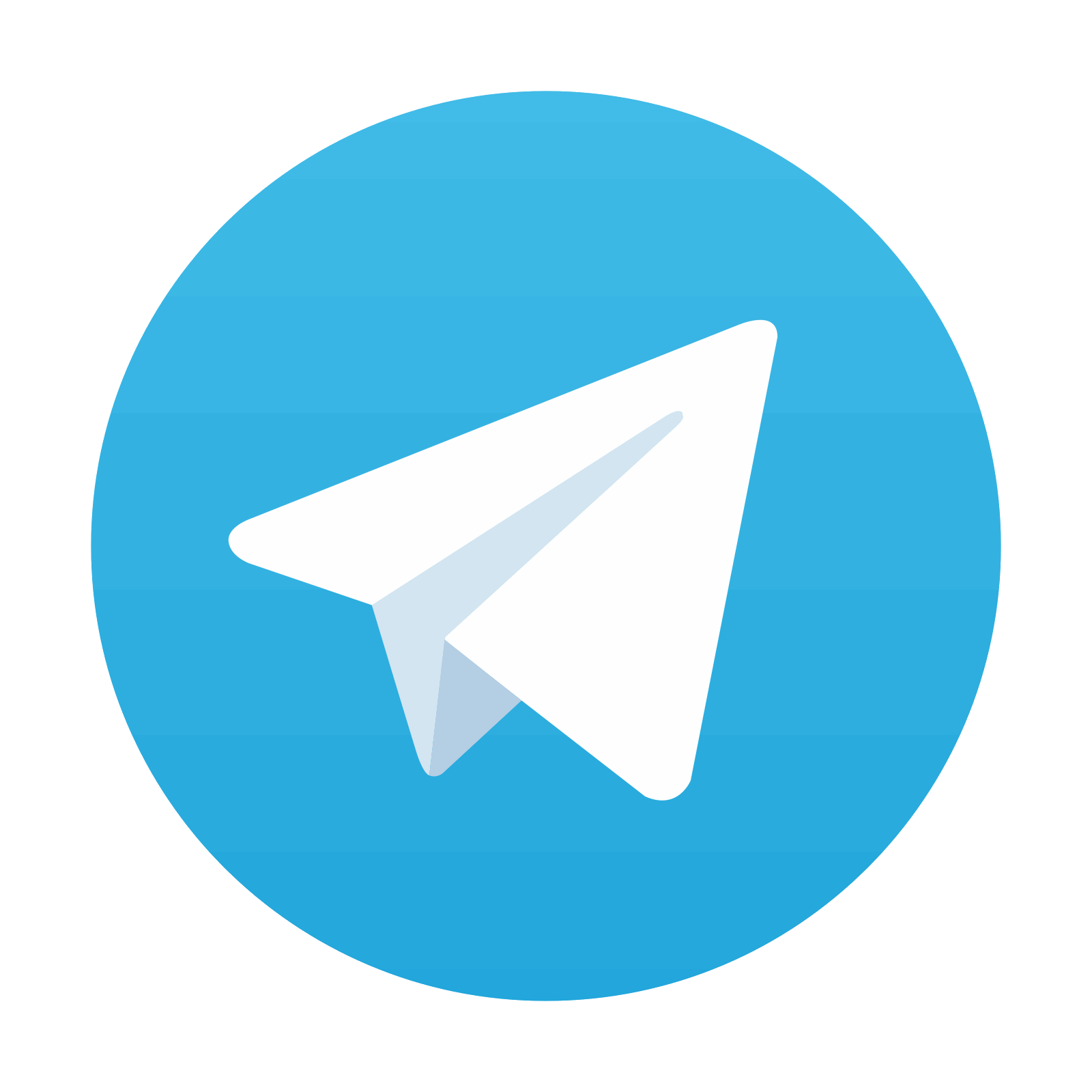
Stay updated, free articles. Join our Telegram channel

Full access? Get Clinical Tree
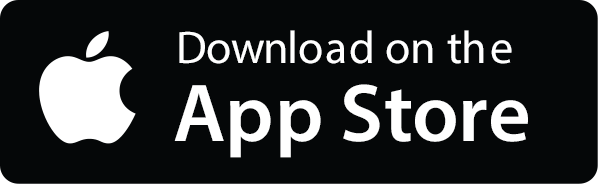
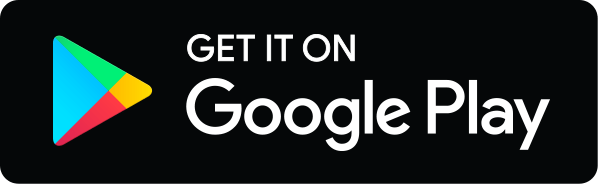