The horse Y chromosome varies considerably (more than twice as compared to the smallest known size) in length among normal individuals (Hansen, 1984; Power, 1988; our unpublished observations). This polymorphism is primarily attributed to the various amount of Y heterochromatin, though earlier studies also suggest variation in the euchromatic portion of the chromosome (Power, 1988). Size polymorphism of the horse Y chromosome is not considered to be breed dependent or related to any clinical or behavioral abnormalities (Hansen, 1984; Power, 1988).
Compared to autosomes and the X chromosome, relatively few numerical or structural cytogenetic abnormalities have been reported for ECAY. This might be because rearrangements in the gene-poor Y chromosome have milder effect on the phenotype, as a result of which the affected individuals escape attention and are not subjected to cytogenetic analysis. Also, the lack of molecular tools for the study of ECAY has considerably hindered the detection of fine-scale cytogenetic aberrations. Majority of abnormalities involving the Y chromosome are mixoploid, mosaic, or chimeric conditions where different cell populations, such as 63,X/64,XY (Hughes & Trommershausen-Smith, 1977; Trommershausen-Smith et al., 1979; Dunn et al., 1981), 64,XX/64,XY (McIlwraith et al., 1976; Dunn et al., 1981), 64,XX/96,XXY (Power & Leadon, 1990), or even 63,XO/64,XX/65,XXX/65,XXY/66,XXXY/66,XXYY (Klunder et al., 1990) are present in the same individual. Most of these conditions are associated with abnormal sex determination and/or sexual development (for review, see Villagomez et al., 2009). However, the XX/XY blood chimerism that has been found in equine heterosexual co-twins does not affect fertility (Miyake et al., 1982; Bugno et al., 2007; Juras R. et al., 2010). This is contrasts the freemartin condition in cattle where blood-chimaeric female co-twins are typically sterile (Padula, 2005). Additionally, there are two reports about infertile stallions having XXY sex chromosomes – an equine counterpart to human Kleinfelter syndrome (Kubien et al., 1993; Makinen et al., 2000).
The only true Y chromosome structural rearrangements, reported so far, are large-scale ECAY deletions in 64,XY sex reversal mares (Raudsepp et al., 2010a), which are discussed in detail later in this chapter, and the presence of Y isochromosome (Herzog et al., 1989; our unpublished data). The latter is a Y chromosome that has lost the short arm and replaced it with an identical copy of the long arm. The resulting isochromosome i(Y) is metacentric with both arms corresponding to Yq (Figure 4.1d). Since the long arm of ECAY carries the MSY and the PAR, the cells with i(Y) are genetically similar to those with Y chromosome disomy (YY). The two individuals carrying isochromosome Y had mosaic karyotypes 63,XO/64,Xi(Y), and both animals were phenotypically abnormal. The horse described by Herzog et al. (1989) was a pseudo-hermaphrodite, and the most recent case (our unpublished data) was an intersex with ambiguous external genitalia. It is, therefore, not clear whether haploinsufficiency for Xp genes in XO cells or the overdose of MSY and PAR genes in Xi(Y) cells is the cause of abnormal sex determination and differentiation in these animals.
Molecular Probes
An important breakthrough in horse Y chromosome research came with the availability of new genomics tools and resources, such as large-insert genomic bacterial artificial chromosome (BAC) libraries (for review, see Rubes et al., 2009), chromosome flow sorting and microdissection technology, and various genomic DNA amplification systems (Telenius et al., 1992; Sorensen et al., 2007). This allowed isolation of ECAY sequences and generation of Y-specific molecular probes. The probes have been used for molecular hybridizations (fluorescence in situ hybridization, or FISH) in clinical cytogenetics, Y chromosome mapping, sequencing, gene discovery, and comparative Y chromosome studies between the horse and other equids/ Perssodactyls.
The first ECAY-specific whole-chromosome painting probes were generated by chromosome microdissection (Raudsepp and Chowdhary, 1999; Kubickova et al., 2002) and used for quick identification of ECAY in cytogenetic preparations (Kubickova et al., 2002), for sperm FISH to detect ECAY aneuploidy (Bugno-Poniewierska et al., 2009), and for horse-donkey Zoo-FISH (Raudsepp and Chowdhary, 1999). Typically, only 10–20 copies of ECAY are microdissected and amplified by DOP-PCR (Telenius et al., 1992). Due to the largely heterochromatic nature of ECAY and repeat-biased amplification of DOP-PCR, microdissected probes tend to be enriched with repetitive sequences, while euchromatic sequences remain underrepresented. Therefore, microdissected Y-chromosome probes are good for FISH but not suitable for cloning and sequencing of the male-specific or pseudoautosomal regions of the chromosome.
An alternative method for the generation of Y-chromosome-specific probes is chromosome flow sorting, which allows isolation of thousands of Y chromosomes in one run. Compared to other domestic species, such as pig and dog, where flow karyotypes were available already in mid-1990s (Langford et al., 1993; Yerle et al., 1993; Langford et al., 1996), flow sorting of horse chromosomes had a late start. According to our best knowledge, the only horse flow karyotype, including the Y chromosome, was generated in 2004 and used for comparative chromosome painting on human, donkey, and mule chromosomes (Yang et al., 2004), and later for karyotype comparisons with other equids and Perssiodactyls (Trifonov et al., 2008). Most importantly, flow-sorted horse Y chromosome (provided by M. A. Ferguson-Smith, Cambridge Resource Centre for Comparative Genomics) has been instrumental for the discovery of Y-linked genes and for generating a comprehensive map for horse MSY (Raudsepp et al., 2008; Paria, 2009; Paria et al., 2011) as discussed later in this chapter.
Horse Y chromosome research has essentially benefitted from the fact that all three available equine BAC libraries, namely CHORI-241 (http://bacpac.chori.org/equine241.htm), INRA (Milenkovic et al., 2002) and TAMU (http://hbz7.tamu.edu/homelinks/bac_est/bac.htm), (see Chapter 3 in this volume), have been constructed from male individuals. Genomic clones containing ECAY sequences have been used for the discovery of Y-linked genes and microsatellites, and as “building bricks” for BAC contig maps of the male specific (Raudsepp et al., 2008; Paria, 2009) and the pseudoautosomal regions (Raudsepp & Chowdhary, 2008). Large-insert clones containing ECAY sequences are also the best probes for FISH analysis either for mapping (Raudsepp et al., 2004; Raudsepp et al., 2008), identification of multicopy sequences (Raudsepp et al., 2004; Raudsepp et al., 2008; Paria, 2009; Paria et al., 2011), or for the detection of large-scale ECAY deletions (Raudsepp et al., 2010a).
Genes
The genes present in the Y chromosome fall into two broad categories: the pseudoautosomal genes, which are shared between the X and the Y chromosome and are discussed later in this chapter, and the male-specific genes that are present only in the Y chromosome.
The study of male-specific genes in horses started about a decade ago, though significant progress in this research has been made only during the past few years. The first gene loci were assigned to ECAY indirectly by PCR showing that primers for TSPY (Manz et al., 1998), ZFY (Senese et al., 1999), and SRY (Hasegawa et al., 1999) amplify exclusively from male genomic DNA and have no amplification in females. Amelogenin primers amplified two distinct products corresponding to AMELY and AMELX in males and only the AMELX product in females (Hasegawa et al., 2000), thus assigning AMELY to the Y chromosome. A few years later, SRY, ZFY, and STS were synteny mapped to the horse Y chromosome by somatic cell hybrid analysis (Shiue et al., 2000). The location of SRY on Yq13 and ZFY on Yq15 was further refined using FISH (Hirota et al., 2001) (Fig. 4.2a). Systematic discovery of ECAY genes started in 2004 when the location and linear order of eight MSY and one PAR gene (Figure 4.2b) was determined using a combination of radiation hybrid analysis, FISH, and BAC contig mapping (Raudsepp et al., 2004). Since then, the ECAY gene catalog has significantly expanded. Recent cDNA selection experiments (Del Mastro & Lovett, 1997) from horse testis, combined with sequencing Y-specific BACs and BAC ends, resulted in the discovery, functional analysis, and mapping of 37 MSY genes (Table 4.1) (Paria, 2009; Paria et al., 2011). Together with the 19 PAR genes (Raudsepp & Chowdhary, 2008), there are currently 56 genes and ESTs assigned to the horse Y chromosome (Figure 4.2c). This makes the ECAY gene catalog one of the most comprehensive Y-specific gene collections in eutherian mammals, standing next to those of human (Skaletsky et al., 2003), chimpanzee (Hughes et al., 2010), and mouse (Yamauchi et al., 2009).
Figure 4.2 Evolution of the horse Y chromosome gene map: (a) ECAY GTG-banded ideogram (ISCNH, 1997) showing the location of the first three synteny (Shiue et al., 2000) and FISH (Hirota et al., 2001) mapped genes; (b) ECAY gene map from 2004 (Raudsepp et al., 2004). Roman numerals mark the seven BAC contigs; (c) current status of ECAY gene map comprising of 37 MSY (Paria, 2009) and 19 PAR (Raudsepp and Chowdhary, 2008) genes. Black rectangles in the PAR and MSY Contig I indicate segmental duplication between the two regions; (d) detailed sequence map of a 146.4 kb region around the SRY locus. Two 100% identical 28 kb directional repeats (black block arrows) flank a 21 kb region that contains the SRY gene. This region is deleted in XY SRY-negative mares.

According to the origin of sequences, the horse MSY genes fall into three categories: (1) X-degenerate, (2) ampliconic, and (3) acquired (Table 4.1). The X-degenerate genes are typically single-copy sequences with an ancestral homologue or a gametologue, on the X chromosome. Examples of such gene pairs are AMELY-AMELX, ZFY-ZFX, or USP9Y-USP9X, and the horse Y chromosome carries 20 X-degenerate genes. A characteristic to X-degenerate genes is ubiquitous expression, so their transcripts are found in most of the body tissues.
Table 4.1 Gene catalog of the horse Y chromosome
The most unique and distinctive feature of MSY is the presence of ampliconic or multicopy genes. Many of those are Y-borne (Murphy et al., 2006; Paria, 2009; Paria et al., 2011), but some have moved to MSY from autosomes or the X chromosome. Amplification of these sequences is the result of gene conversion and/or duplication, which are typical functional features of the non-recombining Y chromosome (Skaletsky et al., 2003). Compelling evidence from human and mouse studies suggest that amplification of these sequences has occurred because they play a critical role in male fertility (Skaletsky et al., 2003; Ellis et al., 2007; Cocquet et al., 2009). Indeed, the majority of the 15 equine Y-linked ampliconic genes are expressed exclusively or predominantly in testis. It is also noteworthy that 10 of these genes are novel and have not been found yet in any other mammalian species. Finally, demarcation between the ampliconic, X-degenerate, and acquired genes is not always distinct. For example, four X-degenerate genes, namely CUL4BY, RBMY, TSPY, and UBE1Y, and an acquired gene ZNF33bY have become multicopy in the course of evolution.
The Y chromosome is also the target of gene acquisition by transposition from other genomics locations. The horse MSY has acquired five such genes from various autosomes and one from the mitochondrial genome. All are expressed ubiquitously, except ZNF33bY, which is testis specific (Paria, 2009; Paria et al., 2011). The horse MSY gene sequences have been studied for the presence of open reading frames and protein-coding ability, and full-length cDNA sequences have been generated for TSPY and a few novel testis-specific transcripts (Table 4.1).
Comparison of ECAY gene catalog with other mammalian species shows that only the X-degenerate genes can serve as comparative loci, whereas the majority of ampliconic and acquired sequences are horse specific (Table 4.1).
Maps
As described in the previous section, the first ECAY maps showed Y-linkage of a few genes without defining their precise location or relative order. Thereafter, the first Y-specific microsatellite sequences were isolated using representation difference analysis (RDA; Wallner et al., 2004). These “seed” sequences were instrumental for initiating systematic analysis of the horse Y chromosome leading to the construction of the first detailed physical map of MSY (Raudsepp et al., 2004). The first-generation composite map (Figure 4.2b) (Raudsepp et al., 2004) combined genotyping on the 5,000 rad radiation hybrid (RH) panel (Chowdhary et al., 2002), BAC contig mapping, BAC fingerprinting, and FISH analysis. The use of four different mapping approaches was necessary because of the unusual and unique features of ECAY of which the most outstanding is the presence of multiple copies of gene-specific and non-genic sequences. This sets limitations to PCR-based mapping approaches such as RH genotyping and sequence tagged site (STS) content analysis. Both methods must be supported by metaphase- (Figures 4.3a, 4.3b), interphase- (Figure 4.3c), and fiber FISH (Figure 4.3d) in order to anchor markers to specific regions, identify multicopy sequences, and validate physical distances and overlaps between the BAC clones on the map.
Figure 4.3 Examples of metaphase (a, b), interphase (c), and fiber FISH (d) to study the horse Y chromosome using (a) a pool of ECAY and PAR BAC clones; (b) BAC clone containing RBMY, SRY, TSPY, and YIR2; (c) BAC clone containing novel multicopy genes ETY4 and ETSTY5; and (d) BAC clones containing KDM5D (green) and SRY (red).

The first-generation ECAY map (Figures 4.2a, 4.2b) shows that the euchromatic region of the chromosome lies in the distal one-quarter of the long arm, where the PAR is located terminally. The rest of the chromosome is predominantly heterochromatic, which is in agreement with cytogenetic studies (Figure 4.1c). Analysis of the 5,000 rad RH panel provided a baseline map spanning 88 centirays with 8 genes and 15 STS markers. Isolation of BAC clones for markers mapped by RH, end sequencing of the BACs, STS development from the end sequences, and bidirectional chromosome walking yielded 109 markers (100 STS and 9 genes) contained in 73 BACs. STS content mapping grouped the BACs into seven physically ordered contigs (Figure 4.2b) that were verified by high-resolution FISH and BAC fingerprinting. It was estimated that the BAC contigs in this map provided ∼20–25% coverage of the euchromatic region of the chromosome.
The first-generation composite map served as an important foundation for expanded studies aimed at developing a minimum tiling path of BACs over the MSY that could finally be used for obtaining complete sequence of the region. The second-generation map of the horse MSY was completed recently (Figure 4.2c) (Raudsepp et al., 2008; Paria, 2009) and comprises of 197 BAC clones that are arranged into a physical map over the male-specific region of ECAY. The five contigs of overlapping BAC clones contain 267 linearly ordered markers of which 230 are STSs and 37 are specific genes or ESTs. Proximally, the map extends into Y chromosome heterochromatin, while the most distal clones span the pseudoautosomal boundary (PAB) and join MSY with the PAR (Raudsepp and Chowdhary, 2008). At the distal end of Contig 1 there is a region containing multiple copies of known genes (CUL4BY, RBMY, TSPY, UBE1Y), several novel equine-specific expressed sequence tags (ESTs), and non-genic sequences. Notably, the horse SRY, despite being a single-copy gene (Paria et al., 2011), is embedded in the multicopy region (Figure 4.2c,d). Unique to the horse Y chromosome is also the presence of a few functional genes in the heterochromatin. The size of MSY, including the four gaps that collectively span about 4–5 Mb, is approximately 16 Mb. Given that the heterochromatic portion counts for about two-thirds of the chromosome, the horse Y chromosome contains about 60 Mb DNA.
While the map gives the most comprehensive overview about the organization of ECAY, the contig of overlapping BAC clones serves as an ideal template for complete sequencing of MSY in the horse. The minimum tiling path (MTP) of MSY comprises of 54 BACs (our unpublished data). However, because of the unusual organization of the Y chromosome, its complete sequencing needs higher redundancy of clones than is necessary for autosomal or X-linked regions (Skaletsky et al., 2003). Currently, more than 100 MSY BAC clones have been selected for next-generation sequencing at Texas A&M University.
The Pseudoautosomal Region (PAR)
The pseudoautosomal region is a region of true sequence homology between the Y and X chromosomes (Skaletsky et al., 2003; Galtier, 2004; Ross et al., 2005; Blaschke & Rappold, 2006). The PAR is essential for sex chromosome pairing and segregation in male meiosis (Ellis & Goodfellow, 1989; Blaschke & Rappold, 2006; Flaquer et al., 2008), and is critical for spermatogenesis and male fertility. Furthermore, recent studies with human embryonic stem cells suggest that the PAR genes might have an important role in early development (Urbach & Benvenisty, 2009). Despite these outstanding biological roles, the physical domain of the PAR, which lies between the terminal end of the sex chromosomes and the pseudoautosomal boundary (PAB), has been determined in only a few mammalian species, including the horse (Raudsepp & Chowdhary, 2008; Raudsepp et al., 2011).
Synaptonemal complex analysis of meiotic prophase chromosomes in stallion spermatocytes shows that at diakinesis, the Y chromosome is associated with the short arm of the X chromosome (Scott & Long, 1980; Safronova & Pimenova, 1988; Power et al., 1992). The exact location of the PAR in ECAY, however, remained unclear (von Nett et al., 1996) until the first pseudoautosomal loci were physically mapped to the terminal regions in ECAYq and Xp (Raudsepp & Chowdhary, 2008).
Using the foundation provided by the first-generation map of the Y chromosome (Raudsepp et al., 2004), a high-resolution BAC contig map has been constructed for the entire horse PAR, extending also into the X- and Y-specific regions (Raudsepp & Chowdhary, 2008). The map consists of 71 BAC clones that are arranged into two contigs, shows linear order of 19 genes (Figure 4.2c) and 110 STS markers, and, for the first time, demarcates the pseudoautosomal boundary (PAB) on both sex chromosomes. The size of equine PAR is about 1.8 Mb. Notably, the draft assembly of the horse genome (Wade et al., 2009) contains only proximal part of the PAR, up to SFRS17A locus (Figure 4.2c), thus missing about 800 kb most distal sequences and six PAR genes.
The physical domain of the PAR is proximally determined by the PAB – a border across which sequence homology between the sex chromosomes reduces and recombination stops (Blaschke & Rappold, 2006; Mangs & Morris, 2007). Equine PAB is located between PRKXY and EIF1AY loci on the Y (Figure 4.2c), and between PRKXY and NLGN4X on the X chromosome. More recently, the complete sequence of the equine PAB was obtained (Raudsepp et al., 2010b). While sequences of the X and Y chromosomes are 98–100% similar in the PAR, this homology disappears after the PAB because a massive LINE L1 element starts immediately proximal to the PAB on the X chromosome. Interestingly, the equine PAB spans a gene (an ortholog of human autosomal XKR3) such that part of the gene is in the PAR and part in the Y-specific region. The gene is truncated and probably nonfunctional on the X chromosome (our unpublished data).
The most unusual discovery regarding the equine PAR is the presence of a ∼200 kb duplication between the middle region of the PAR and the proximal part of MSY (Raudsepp & Chowdhary, 2008) (Figure 4.2c). In situ hybridizations with BAC clones from the two regions consistently show three signals on male metaphase chromosomes: one each on the terminal ends of ECAXp and ECAYq (i.e., the PAR) and one on the most proximal part of the MSY (Figure 4.4). This might be the result of duplications combined with unequal crossover events between the sex chromosomes during evolution. Interestingly, similar duplications between MSY and the PAR were detected in two other equids – donkey and Hartmann’s mountain zebra (our unpublished data) – suggesting that these evolutionary rearrangements happened before the divergence of extant equids. The likely impact of the observed duplication on pairing between the sex chromosomes during meiosis and the possible effect of the presence of three copies of functional sequences in males are intriguing.
Figure 4.4 FISH on metaphase (a) and interphase (b) chromosomes showing the duplication between the proximal part of MSY and the PAR.

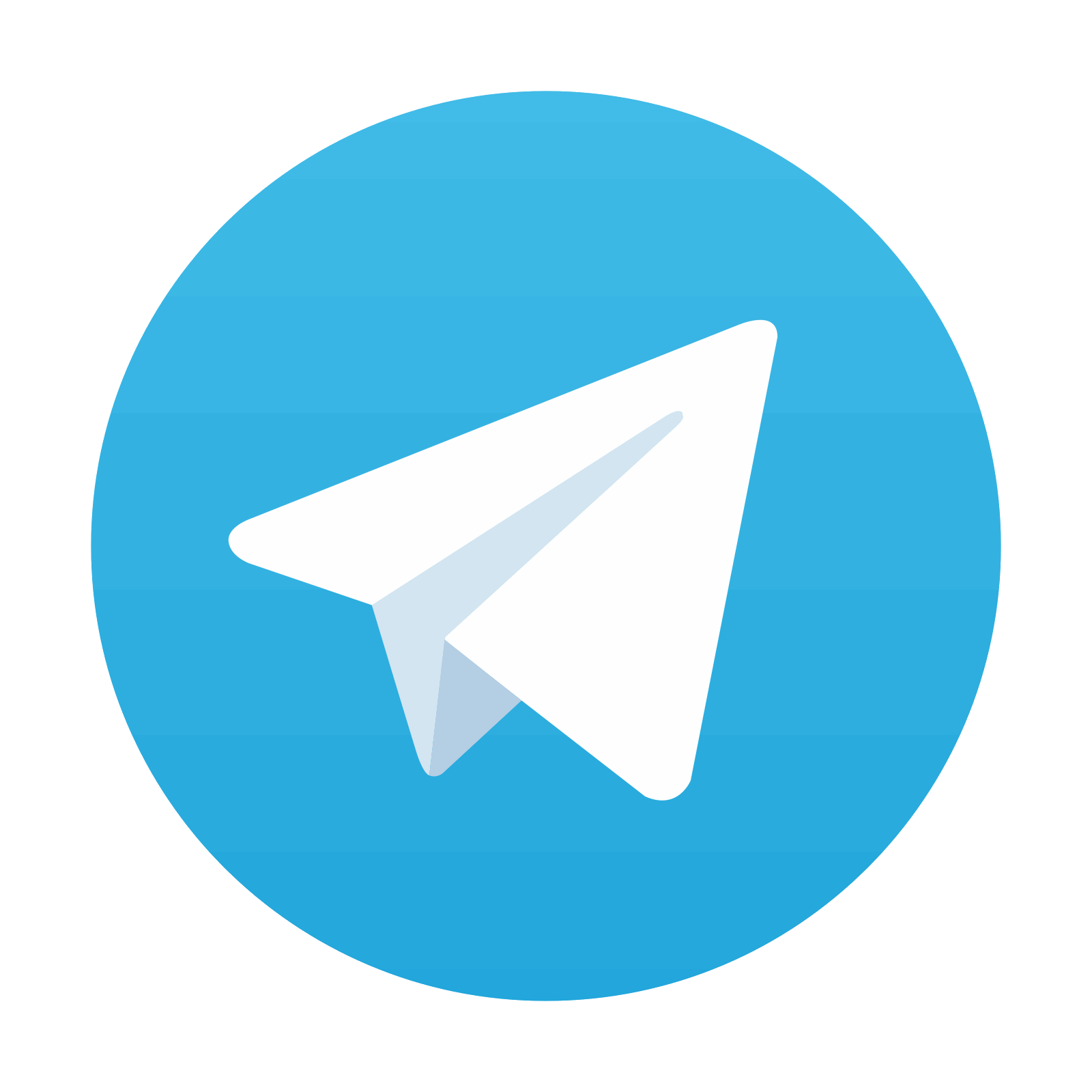
Stay updated, free articles. Join our Telegram channel

Full access? Get Clinical Tree
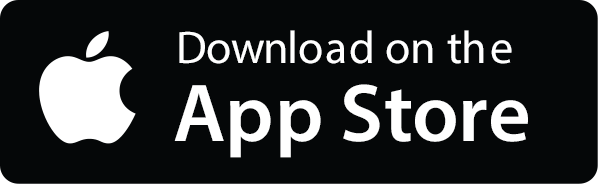
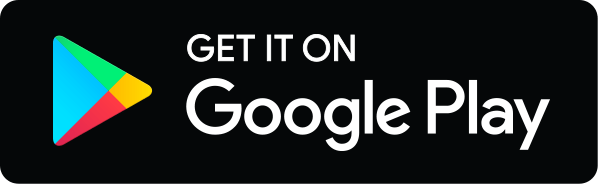