, Monika Hassel2 and Maura Grealy3
(1)
Centre of Organismal Studies, University of Heidelberg, Heidelberg, Germany
(2)
Spezielle Zoologie, Universität Marburg FB Biologie, Marburg, Germany
(3)
Pharmacology and Therapeutics, National University of Ireland Galway, Galway, Ireland
6.1 Human Embryos and Model Organisms
6.1.1 Does Knowledge of the Development of a Fly Help to Understand Human Development?
Naturally, our main interest is to learn how we ourselves have once developed. How could information of the development of a mouse, or even of a frog or fly, help to understand human development?
The zoologist knows that human beings are members of the mammalian family of primates and, therefore, is not surprised to learn that early human embryogenesis exhibits considerable differences to that of mice, but shows only little differences to that of other primates. Self-evidently, disparities between the development of humans, frogs and flies are huge – in the final outcome. It is more astonishing that comparing embryonic development of forms such diverse as humans, frogs and flies discloses so many and fundamental commonalities. Modern molecular genetics has disclosed uncanny likeness and unexpected homologies. The common genetic basis will be dealt with in Chap. 12.
Experiments with model organisms introduced in the Chaps. 4 and 5 facilitated, or even enabled in the first place, the disclosure of many fundamental control mechanisms and genes involved also in human development. A pioneering finding was that many developmental malformations known to physicians since ancient times can be evoked in animal model organism experimentally. A classical historic example was the generation of “Siamese twins” in amphibians (see Fig. 13.1). Further examples are the many known hereditary symptoms of diseases that in similar form can be evoked “in animal disease models” by targeted mutagenesis and genetic crosses.
Although laws, ethical and religious reservations forbid experimental interference, the development of man is the subject of morphological, histochemical, molecular and genetic investigations. For example, the pattern of gene expression can be studied with in-situ-hybridization (see Fig. 12.5) in embryos lost from pregnant women. In addition to aborted embryos, living cells isolated from human teratocarcinomas are available for studies. The following description provides information on peculiarities of mammalian development in general and human development in particular, compared to traditional generalizing descriptions of animal development.
For a better understanding, the description of amphibian and avian development should be read first. On the morphological as well the molecular biological level many congruities have been found between mammals and amphibians, whose ancestors also gave rise to land vertebrates. And since a reptilian ancestor was inserted in the phylogenetic lineage leading from amphibian to mammalian ancestors, avian development can also confer information about our evolutionary heritage (zoologists conjoin reptiles and birds to a comprehensive taxon Sauropsida).
6.2 From Primordial Germ Cells up to Fertilization
6.2.1 Oogenesis: Long Before Their Own Birth, Females Already Possess a Large Store of Egg Cells; but Their Growth and Maturation Still Take Much Time
While still babies or embryos, girls store thousands of eggs which pause in meiotic prophase I for many years. Primordial germ cells (oogonia) destined to give rise to the next generation are generated and laid aside early in embryo development; they can be identified in the pre-natal female embryo in the 3rd week of development. By this time, the primordial germ cells immigrate from the yolk sac by amoeboid movements into the gonadal ridges (see Figs. 8.3f and 15.2). In the 5th month of pregnancy the female embryo harbours some seven million oogonia in her ovaries. By the 7th month of development the majority have perished. The surviving 0.7–2 million oogonia stop mitotic multiplication and become primary oocytes. These enter the prophase of meiosis I (Fig. 6.1) but pause at diplotene (Figs. 6.1 and 8.4). There follows an interval lasting 12–40 years, in which lampbrush chromosomes and multiple nucleoli (Fig. 8.5) may be present in oocyte nuclei (not firmly established for human oocytes). Much transcription takes place, many ribosomes are produced, and the oocyte is fed with yolk material by the surrounding follicle cells. Even the relatively small human oocyte undergoes a 500-fold increase in volume.
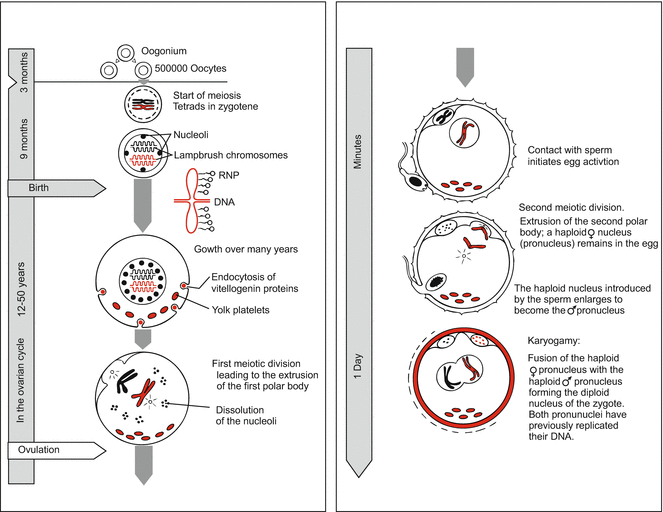
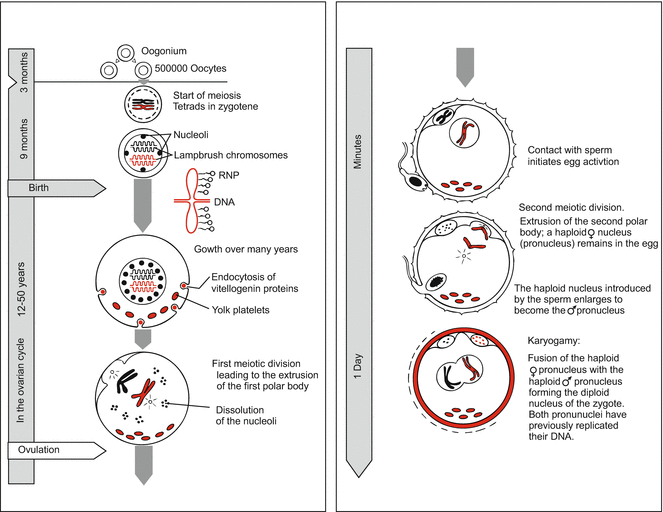
Fig. 6.1
Human. Oogenesis in review. Occurrence of lampbrush chromosomes is typical for vertebrates but not yet definitively established for humans
During childhood, many more oocytes perish. At puberty some 40,000 are left; they are enveloped by nourishing primordial follicles. Stimulated by the pituitary gonadotropin follicle-stimulating hormone (FSH), 5–12 oocytes begin to mature in each ovarian cycle. Later, the follicles are exposed to another gonadotropin, the luteinizing hormone LH (Fig. 6.2). These hormones signal the egg to resume meiosis and to initiate the events culminating in the release of the mature egg from the ovary. As a rule, in one ovarian cycle, which lasts some 28–30 days, only one (or up to three) of the follicles attains the state of a mature Graafian follicle. In the middle of the menstrual cycle, about 14 days after the last menses, one of the oocytes finishes the first meiotic division by extruding the first polar body, and the egg is released from the ovary (ovulation). In mammals including man the second polar body is not constricted off until the egg is fertilized.
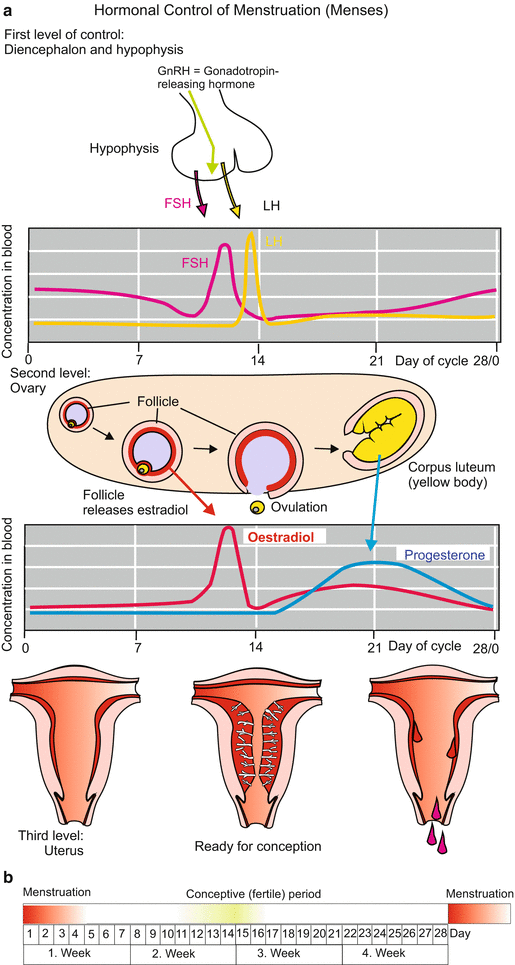
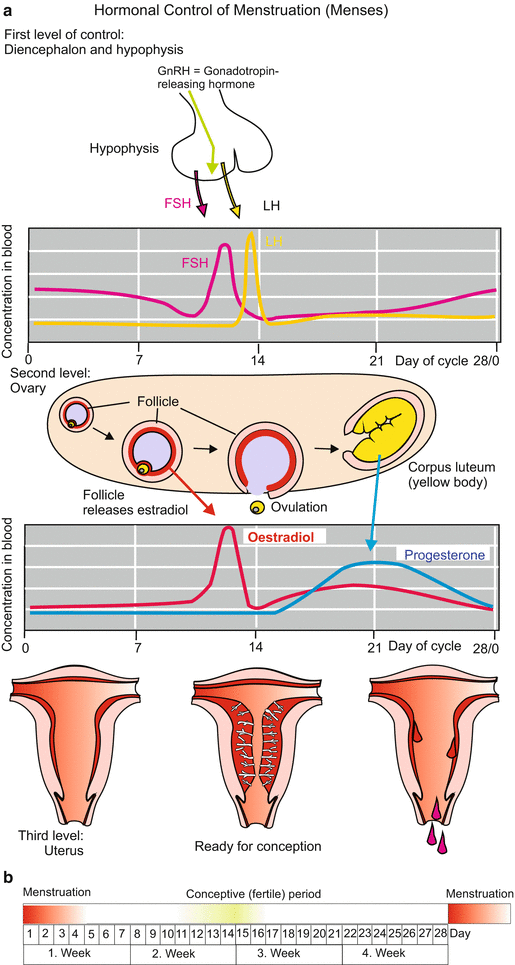
Fig. 6.2
(a) Human. Hormonal control of the ovarian cycle in preparation of pregnancy. Provisions are made at three levels: Level I: Pituitary gland, the hormones FSH and LH trigger the final maturation of the egg cell and its release from the ovary (ovulation). Level II: In the ovary the female sexual hormones are produced, oestradiol in the follicle, progesterone from the yellow body (corpus luteum). The hormones are released into the circulation and reach the uterus. Level III: The sexual hormones induce the growth of the nutritive mucosa which is shed if no germ lodges itself in it. (b) Conceptive period
Before fertilization takes place, the egg is caught at the mouth of the oviduct. If not, a fertilized egg that falls into the abdominal cavity dies or may give rise to a malformation exhibiting tumour-like features, called a teratocarcinoma.
The time point of ovulation, the short window of 6–12 h in which the egg can be fertilized, and the short, at the maximum 3 days, life span of the sperm, restrict the conceptive period in the cycle of a women to about 4 days in the middle of the menstrual cycle (Fig. 6.2b). Physicians assume the optimal time point for conception to be the day before ovulation.
An Annotation to Present Medical Reproduction Biology
In industrial countries about 12 % of the women suffering breast cancer are still in the reproductive age of 20–34 years, and even young girls can develop breast cancer, boys may develop other kinds of cancer. Yet, the unavoidable chemotherapy destroys the ovaries or testes, respectively. Therefore, present reproduction biology develops methods (in animal experiments) to remove tissue from the ovary or testis before cytostatic agents are given, to store the removed tissue deep-frozen (cryopreservation) and to re-implant it upon successful therapy.
6.2.2 Only One of Millions of Sperms Gets a Chance
Once caught by the mouth of the oviduct, the egg awaits the sperm with reservation: it is surrounded by a tough envelope, called the zona pellucida, and by a corona of mucous material, called the corona radiata. Both these envelopes have been produced by the ovarian follicle, and must be penetrated by the sperm.
From 200 to 300 million sperm released into the vagina in each ejaculation about 1 % advance to reach the egg. On their long journey through the vagina, uterus and oviduct the sperm cells undergo capacitation, that is, they acquire the competence or capacity to fertilize through the influence of female secretory products. Only one sperm succeeds in entering the egg cell. The other sperm may help to dissolve the egg’s envelopes by releasing acrosomal enzymes (Chap. 3).
Whether in humans pregnancy can occur naturally without the contribution of a male individual (parthenogenesis) is discussed in Box 6.2.
6.3 From Fertilization up to the Phylotypic Stage
6.3.1 The Egg First Develops “Extraembryonic” Organs to Tap the Mother, Therefore the Embryo Can Grow Enormously
Pre-implantation development (Fig. 6.3). Because the embryo will be provided with nutrients by the mother early, the egg can be void of yolk, and it is in fact almost free of yolk. The human egg is amazingly small, just 0.1 mm in diameter and thus not larger than the egg of sea urchins, and considerably smaller than the egg of the small fruitfly. The egg and early embryo can sit enthroned on the tip of a needle (Fig. 6.3).
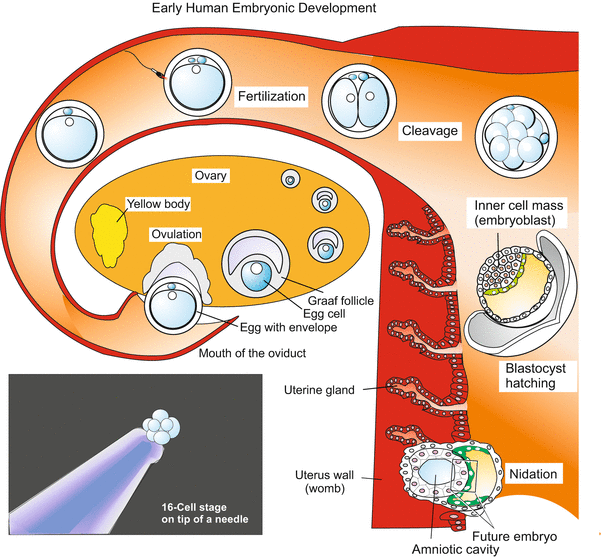
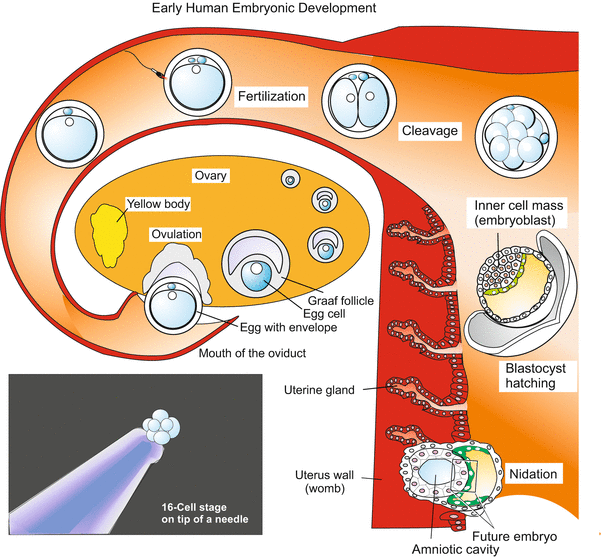
Fig. 6.3
Human: From ovulation to implantation. A mature egg contained in a Graafian follicle of the ovary is liberated and picked up by the tube of the oviduct. Fertilization, formation of the second polar body and cleavage take place in the oviduct, which leads into the uterus. Once arrived in the uterus, the embryo has reached the blastocyst stage. The blastocyst hatches out from its envelope (zona pellucida) and attaches to the wall of the uterus. Now the blastocyst begins to invade the uterine wall
Although the mammalian egg lacks significant quantities of yolk and has reverted to holoblastic cleavage, cleavage in mammals is extremely slow. This apparently retarded development is correlated with an early start of transcription of zygotic genes; no midblastula transition as it occurs in amphibians (and other animals) is known from mammals. Not until the third day is the 12–16 cell stage of the morula reached. By the 6–7th day the blastocyst stage is attained. The blastocyst looks just like a blastula, but it isn’t a blastula that would proceed to form an archenteron through invagination as do the true blastulae of sea urchins and amphibians. Rather, the cellular wall of the blastocysts undergoes differentiation to become the trophoblast (trophectoderm). This is an ‘extra-embryonic’ organ destined to invade the wall of the maternal uterus, absorb maternal nutrients, and dispose of waste. After implantation the trophectoderm undergoes several rounds of endoreplication and develops polyploid giant cells. Part of the trophoblast will eventually give rise to the placenta, a foetal organ formed to optimize all of these functions (Sect. 6.4). The trophoblast encloses a cavity and the inner cell mass, also called the embryoblast because those cells, which remain diploid, eventually will generate the embryo proper. The inner cell mass is a group of cells eccentrically concentrated at a location beneath the ‘polar’ trophoblast. The blastocyst hatches, attaches to the uterine wall and sinks into it, a process known as implantation or nidation.
If the mouth of the oviduct fails to catch the egg, and the blastocyst settles down in the abdominal cavity (ectopic abdominal pregnancy) the embryo dies or develops to a tumour-like teratoma, a risk a woman has to bear; a teratoma eventually must be surgically removed.
After extracorporeal fertilization the fertilized egg can, appropriate culture media provided, develop in vitro up to the blastocyst stage. Then, according to the current state of reproductive medicine, further development must take place in the uterus.
Post-implantation development (Figs. 6.4, 6.5, 6.6, 6.7, 6.8, 6.9, 6.10, 6.11, and 6.12). The hatched blastocyst attaches with its polar trophoblast to the wall of the uterus. Entry is facilitated by lytic enzymes released from the trophoblast. The outer layer of the trophoblast proliferates into the uterine tissue. While doing so it transforms into a multinucleated syncytial trophoblast; the inner layer of the trophoblast, called the cytotrophoblast (Figs. 6.4 and 6.5), remains cellular and encloses the blastocoel. Later the wall of the blastocyst is supplemented by an inner layer, called the parietal mesoderm. The cavity of the blastocyst will not be enclosed in the embryo and is therefore called the exocoel or chorionic cavity.
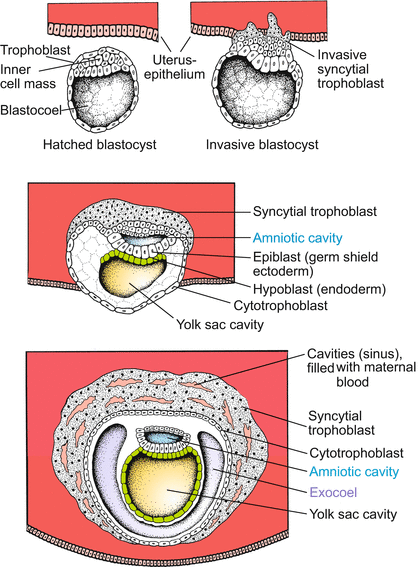
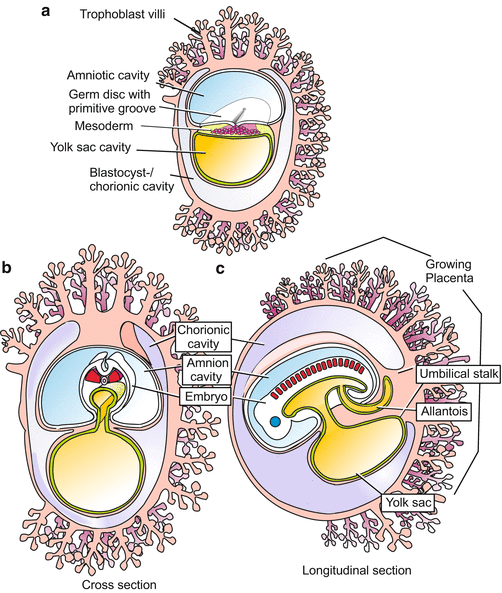
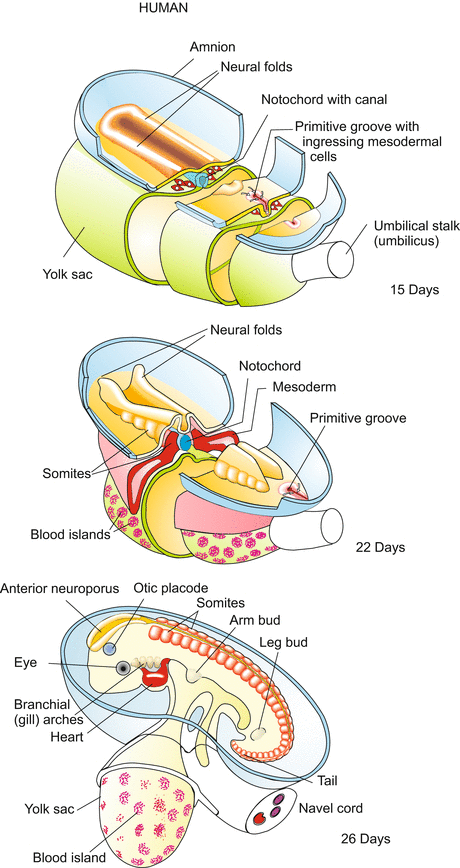
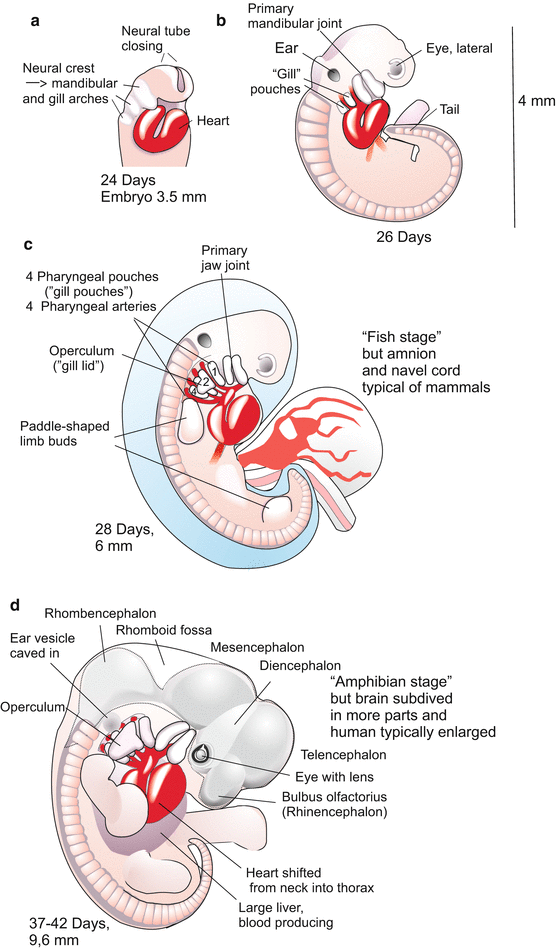
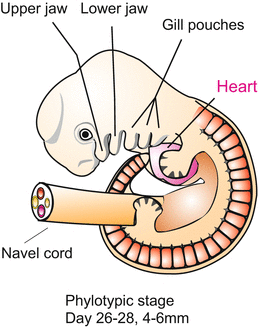
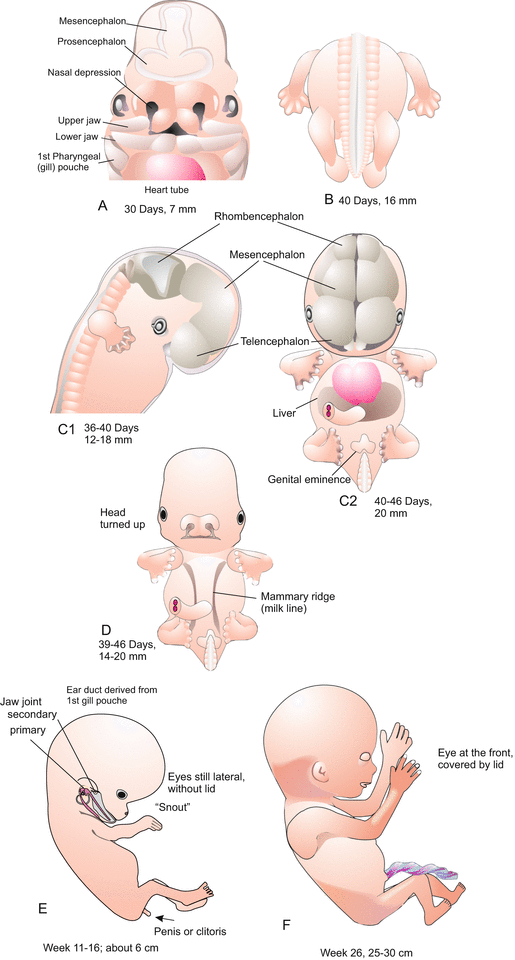
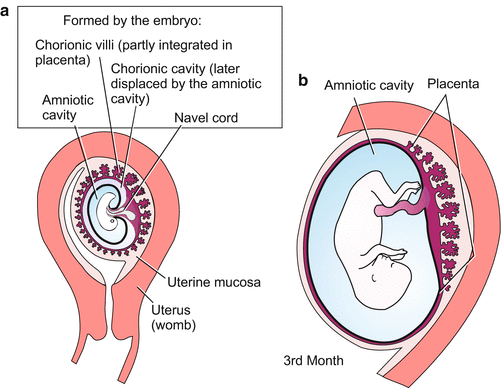
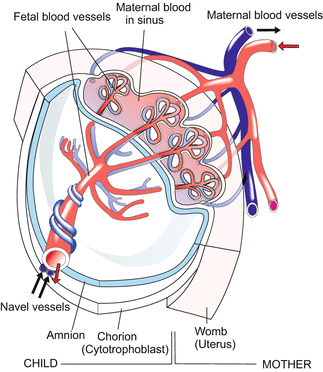
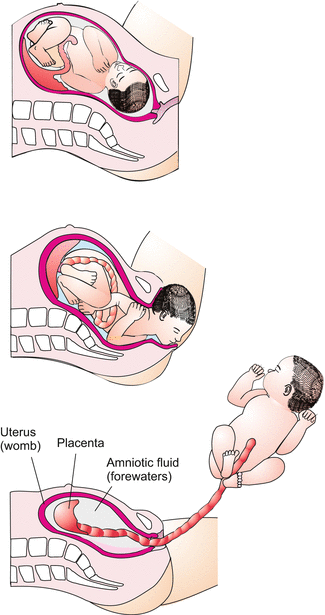
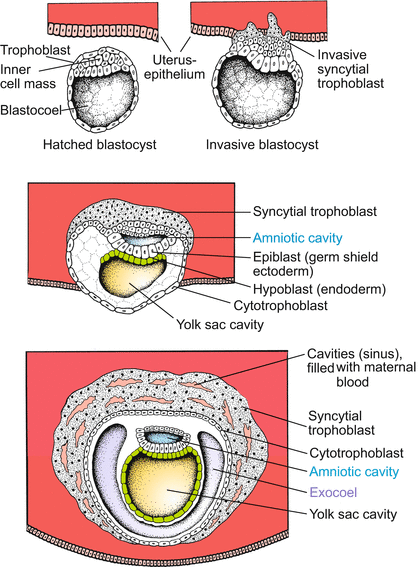
Fig. 6.4
Human: Implantation (nidation), amnion, yolk sac. The invasion of the uterine wall is accomplished by an egg-derived ‘extraembryonic’ tissue or organ, the trophoblast. It shows features of a terminally differentiated tissue, including polyploidy. The peripheral invasive trophoblast forms a syncytium by dissolving cell membranes. The amniotic cavity is not formed by amniotic folds (as in the avian and insectivore embryo) but by the separation of cells of the inner cell mass. Hypodermal (endodermal) cells surround the yolk sac cavity, forming the (empty) yolk sac. An embryo proper is not yet formed
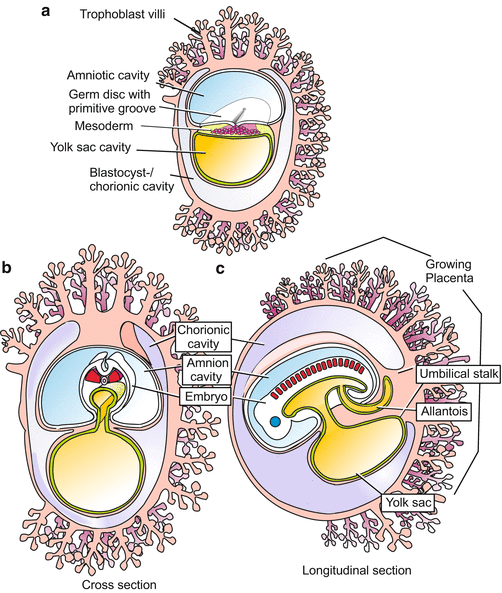
Fig. 6.5
(a–c) Human. Embryonic development up to the somite stage (phylotypic stage). The embryo is formed by a blastodisc on the floor of the amnion. The blastodisc is composed of epiblast and hypoblast. Gastrulation occurs by a process highly reminiscent of gastrulation (primitive streak formation) in birds (see Fig. 5.25c, d). Extraembryonic organs are yolk sac, allantois (partially), trophoblast-derived villi and eventually the placenta
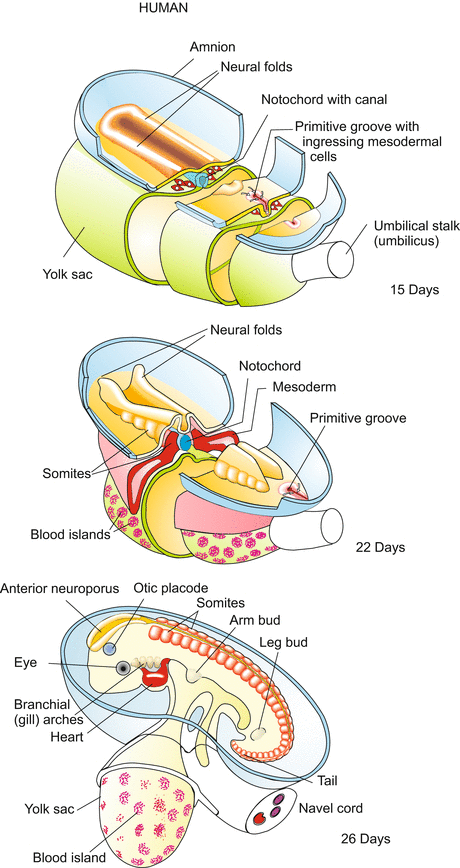
Fig. 6.6
Human: Neurulation, organogenesis. The surrounding envelopes (trophoblast and chorion) are removed and the amniotic cavity opened to show the blastodisc. The neural folds form in front of the primitive groove, just as in the avian embryo (compare Fig. 5.25c, d). The notochord is formed by the roof of the archenteron; in an intermediate stage of its formation the tubular notochord encloses the notochordal canal. The rudimentary allantois and the yolk sac become integrated into the umbilical cord. During the entire development shown the embryo increases in length from about 1 to 5 mm
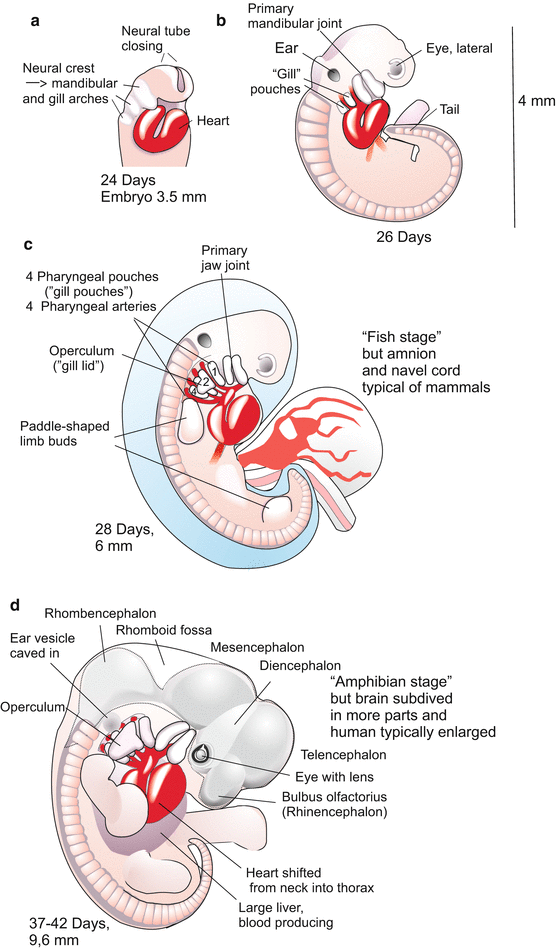
Fig. 6.7
(a–d) Human. Development of the embryo in the first 6 weeks post fertilization
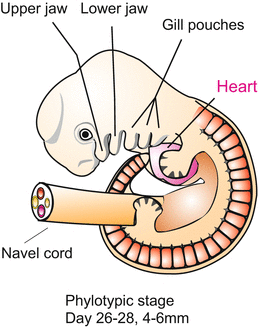
Fig. 6.8
Human. Phylotypic stage, length 4–6 mm. The global organ anlagen are present; but later subdivided, elaborated and refined through different growth rates and different paths of terminal differentiation
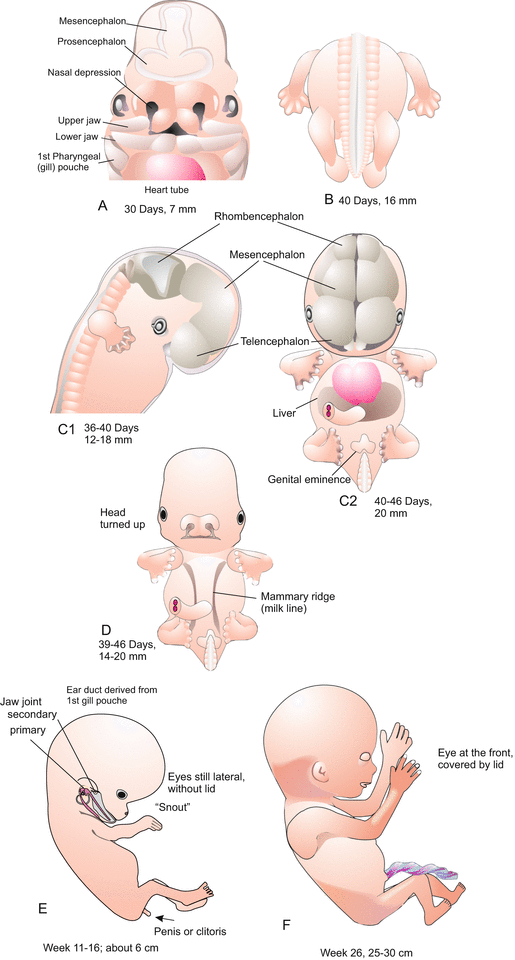
Fig. 6.9
(a–d) Human. Development from day 30 to day 46. (e–f) Human. Development from week 11 to 26
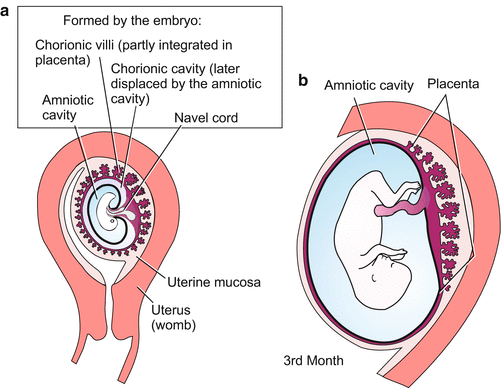
Fig. 6.10
(a, b) Human: Placentation 1. The chorionic villi (tree-like structures) are reduced on one side but enlarged on the side that faces the maternal uterine wall. They concentrate in a disc-shaped area called placenta. The amniotic cavity ousts the chorionic cavity
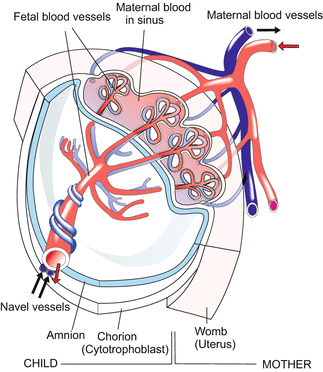
Fig. 6.11
Human: Placentation 2. The villi are provided with blood vessels (umbilical vessels) and together constitute the major part of the placenta. The maternal endometrium contributes to the placenta in a negative way as it partially dissolves. The resulting spaces (lacunae) are filled with maternal blood. The chorionic villi of the foetal placenta dip into the maternal blood
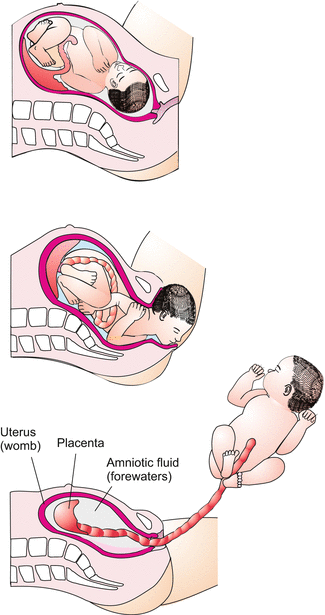
Fig. 6.12
Birth. Note: the “waters” flowing out consist of amniotic fluid
The invasive syncytial trophoblast prepares the space into which the embryo can grow. The solid epithelial cytotrophoblast becomes reinforced and supplemented by mesodermal cells that immigrate from the embryo: the whole organ is known as the chorion. From its outer surface primary chorionic villi (Fig. 6.5) project and extend through the loose syncytiotrophoblast to establish contact with the surrounding maternal uterine tissue. With these chorionic villi the embryo takes up nutrients and O2 from maternal blood lacunae of the decidua (layer of the uterus surrounding the germ). In human embryological illustrations the term trophoblast usually is now replaced by the term chorion. The nutritive organ of the chorionic villi is designated extra-embryonic, meaning that the villi are not organs of the embryo proper; this does not yet exist at this stage. The chorionic villi are the functional precursors of the much larger villi that are later formed by the placenta.
6.3.2 In Human Embryonic Development Evolutionary Ancient Structures Emerge: Primitive Groove, Yolk Sac, Amnion and Allantois
Amnion, Yolk Sac, Umbilical Cord.
Before the embryo develops, the human blastocyst, like most mammalian blastocysts, makes some preparations for the benefit of the coming embryo. The embryos of reptiles and birds have enclosed themselves with a self-made, water-filled amniotic cavity, and they wore a yolk sac. The mammalian embryos conserved these innovations. However, the germs of most mammals establish an amniotic cavity and a yolk sac before an embryo proper exists at all, and so it is in humans, too. By the 9th day the amniotic cavity appears as a cleft in the midst of the inner cell mass. From this inner mass an epithelial layer called the hypoblast separates. It expands, lining the central cavity and forming the parietal endoderm or yolk sac (a misnomer since it does not contain yolk!).
The volume of the amniotic cavity increases with the increasing size of the embryo. Its fluid is known as waters or forewaters. Unlike in the mouse, in humans the evolutionary old primary yolk sac bursts open and becomes replaced by a secondary sac. Eventually, the yolk sac is enclosed by extraembryonic endoderm and mesoderm, integrated into the umbilical stalk and accompanied by blood vessels; all these structures together are twisted to yield the umbilical cord. Into the umbilical cord also the rudimentary allantois is integrated. The allantois, in reptiles and birds serving as embryonic urinary bladder, can in mammals be rudimentary because the embryo can hand over oxidation water and its metabolic end products to its mother for disposal.
Embryogenesis.
What happens inside the (former) blastocyst? Where is the embryo formed? The embryo proper is formed from two minute epithelial areas: from an area in the floor of the amniotic cavity, now called the epiblast, and the roof of the yolk sac, known as the hypoblast. Together these two adjoining areas, one on top of the other, represent the blastodisc.
From this point on, generation of the actual embryo follows the path paved by our non-mammalian ancestors.
Gastrulation, germ layer formation, and neurulation are similar in many ways to avian embryo development (Figs. 6.5 and 6.6; compare Fig. 5.25). In gastrulation, epiblast cells move to a primitive groove and pass through it, colonizing the cleft between the epiblast and the hypoblast. In front of the groove, a primitive streak appears. Underneath, the invading cells give rise to the mesoderm, and perhaps contribute also to the endoderm which essentially derives from the hypoblast. The mesoderm subdivides itself in the traditional manner giving rise to notochord, somites, and lateral plates. Atop the notochord the epiblast forms neural folds that close to form the neural tube (Fig. 6.6). Thus, gastrulation (through a primitive groove), neurulation, and formation of notochord and mesodermal primordia, follow traditional basic patterns of vertebrate development.
There are, however, mammalian-specific peculiarities. The anterior part of the notochord, known as the “head process” pushes anteriorly and contacts a prechordal plate which will give rise to head muscles and to the pharyngeal membrane. Initially and temporarily, the notochord is hollow. The chordal canal begins with an open pore in the primitive groove close to the node and ends with another open pore in the yolk sac. A similar chordal canal (canalis neurentericus) is found in reptiles and some birds.
When the neural folds merge above the notochord to form the neural tube, this tube also remains open at its anterior and posterior ends. Both the anterior and posterior neuropores connect the neural canal with the amniotic cavity. The pores are closed when the majority of the somites, the optic vesicles, the ear placodes and the pharyngeal pouches (“gill arches”, Figs. 6.7, 6.8, 6.10, 6.11, 6.12, 6.13, 6.14, 6.15, 6.16, 6.17, 6.18, 6.19, 6.20, and 6.21) are visible, and the heart beats.
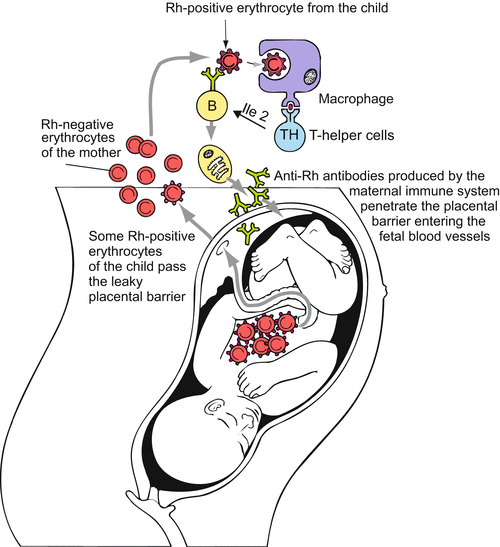
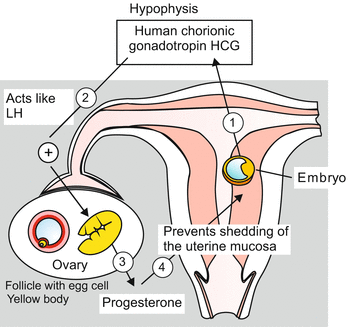
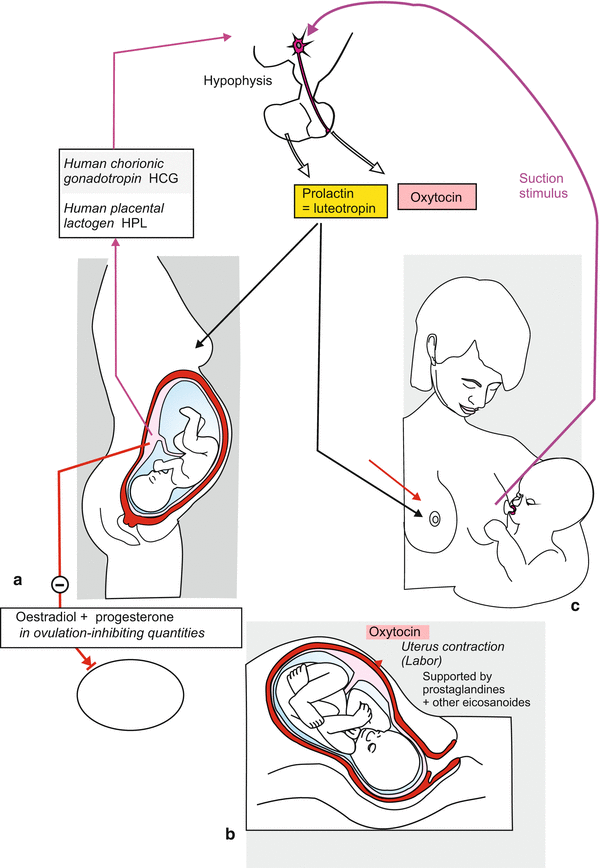
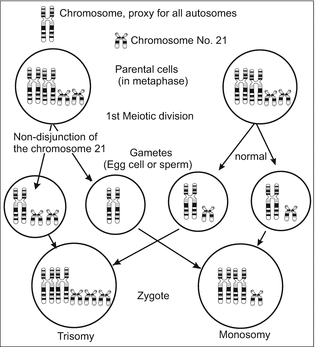
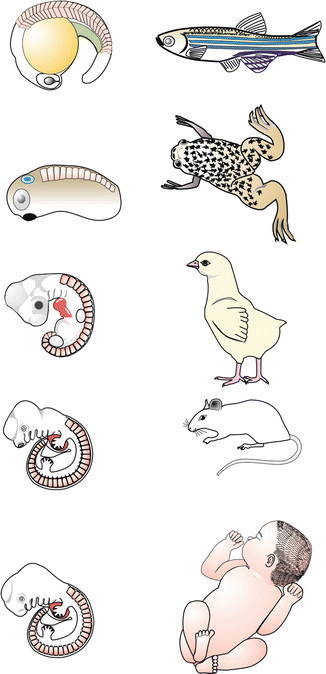
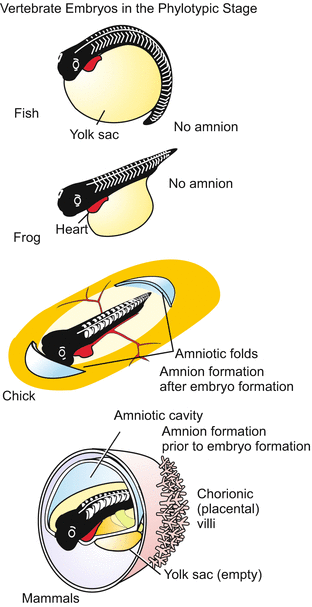
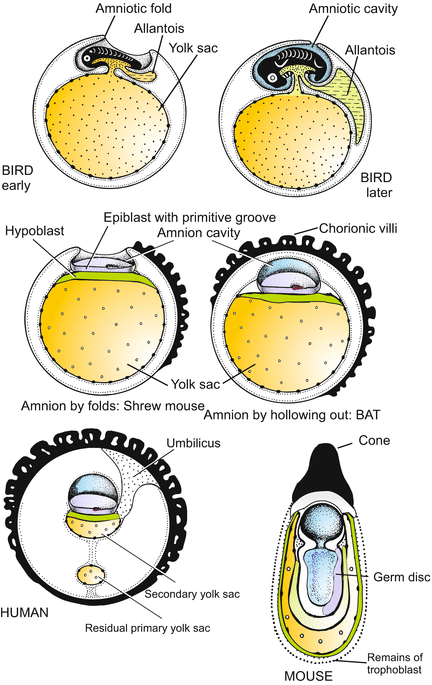
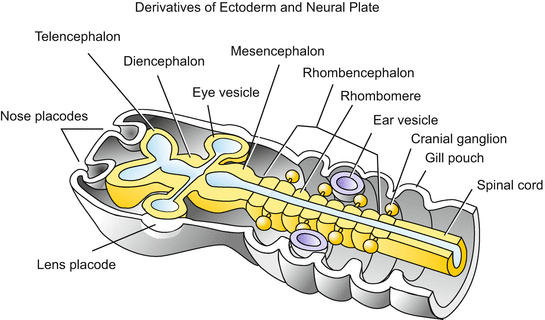
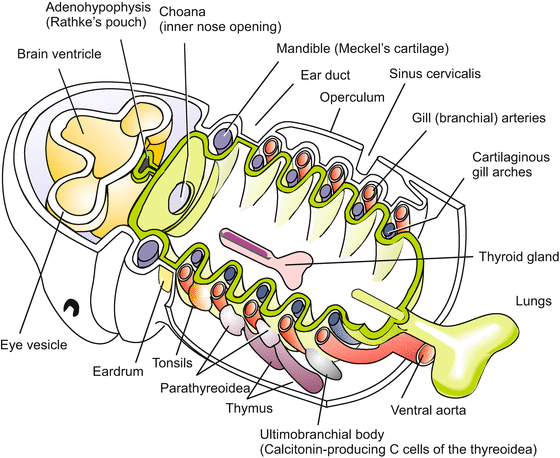
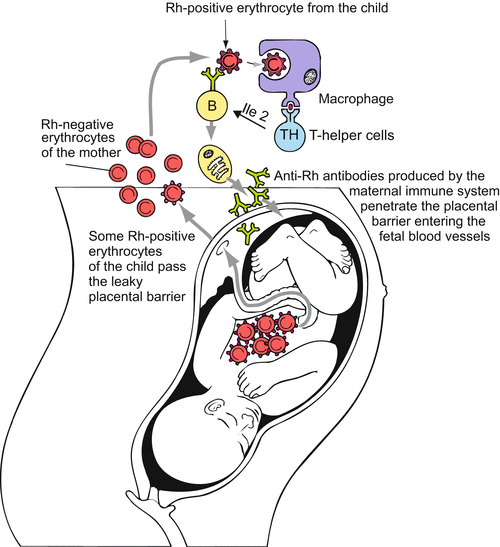
Fig. 6.13
Danger for the child by the immune system of the mother exemplified by the immune response elicited by the Rhesus factor. The Rhesus factor is a complex of glycoproteins on the surface of the red blood cells consisting of 50 defined blood-group antigens, among which the D antigen is the most important. The commonly used terms Rh factor, Rh positive and Rh negative refer to the D antigen. Rh positive erythrocytes of the child bearing the D antigen are dealt by the immune system of a Rh negative mother as foreign. When Rh positive erythrocytes get into the blood of a Rh negative mother via a leaky placental barrier (or on occasion of the birth of a former Rh positive sibling) the maternal immune system generates anti-RH antibodies (= anti-D antibodies). These can infiltrate the child causing haemolytic disease of the fetus and newborn
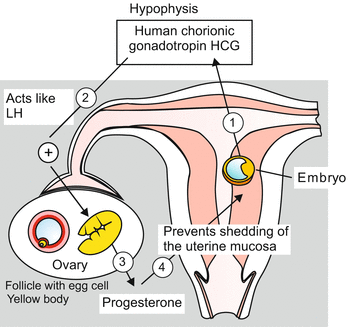
Fig. 6.14
Hormones preventing further menstruation after a germ has implanted in the uterus
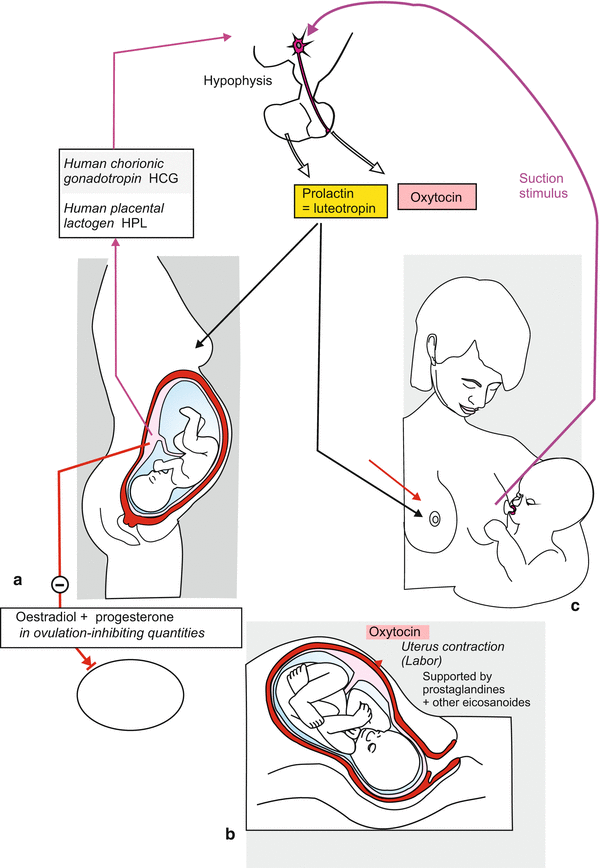
Fig. 6.15
Hormonally controlled events before, during and after birth. Hormones of the child and the mother interplay
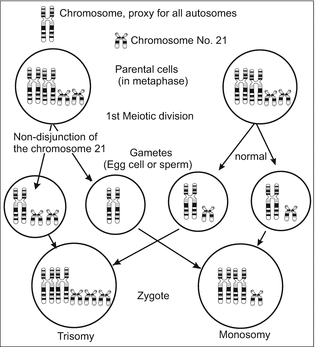
Fig. 6.16
Trisomy 21 caused by meiotic nondisjunction. The small chromosome symbolizes chromosome No 21
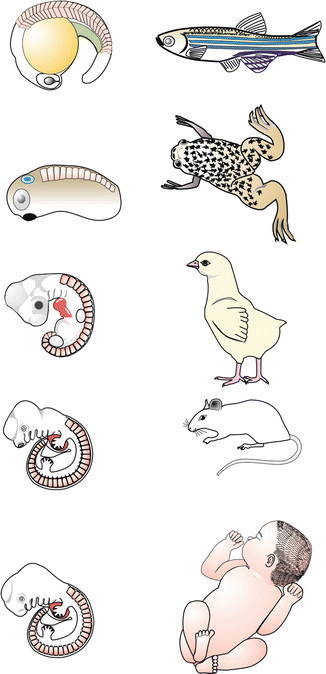
Fig. 6.17
Embryos and juvenile stages of various vertebrates illustrating an observation of Carl Ernst von Baer that a certain early embryonic stage (now termed the phylotypic stage) is very similar among the various vertebrates, while divergence occurs during later development. The phenomenon prompted Haeckel to formulate his ‘biogenetic law’
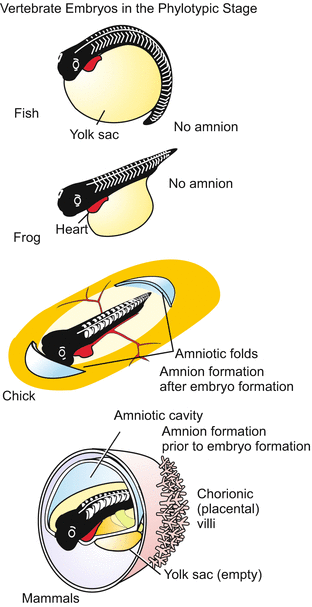
Fig. 6.18
Embryos of non-amniotes (fish and frog) and of amniotes (chick and mammals). Note that in chick amniotic folds do not emerge until the embryo has reached the phylotypic stage, while in mammals embryonic development commences within a previously formed amniotic cavity
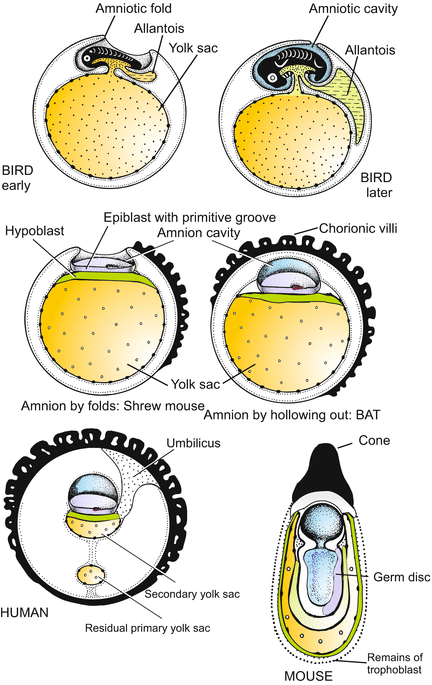
Fig. 6.19
Amniotes: Differing formation of the amniotic cavities and other extraembryonic organs in different land vertebrates
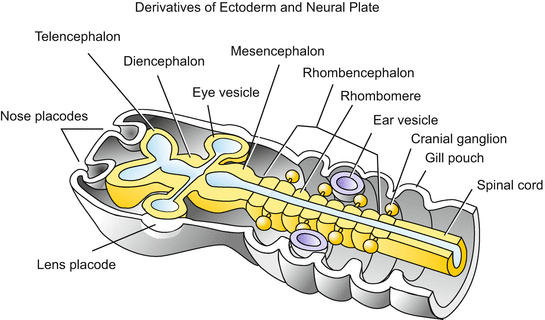
Fig. 6.20
Ectodermal and neural plate derivatives in the anterior body showing the segmental structure of the hindbrain and pharyngeal region. In the hindbrain (rhombencephalon, medulla oblongata) seven segmental constrictions mark off 7–8 repeating units called rhombomeres or neuromeres. Branches originating from the first even-numbered rhombomeres (r2, r4, r6) and the associated ganglia give rise to cranial (trigeminal, facial, vestibuloacoustic) nerves. The subsequent posterior branches and ganglia are known as the glossopharyngeal nerve and vagus; the subsequent nerves are called spinal nerves (dorsal and ventral roots, dorsal root ganglia) in that part of the central nervous system known as the spinal cord. The segmental organization of the medulla oblongata corresponds to the segmental organization of the pharyngeal pouches and organs
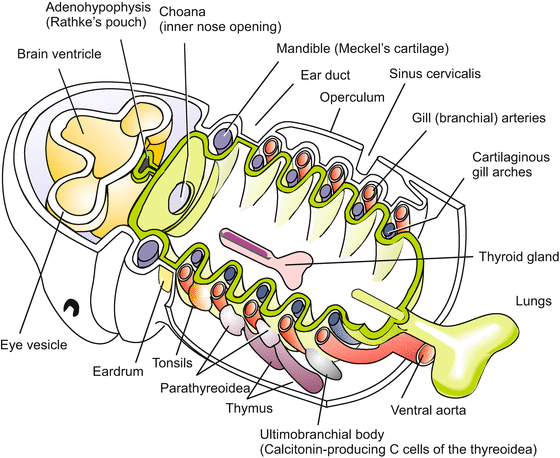
Fig. 6.21
Phylotypic stage of vertebrates, showing the derivation of the branchiogenic organs in the anterior body (head and pharyngeal region). The lymphatic organs (tonsils and thymus) and the hormone glands (parathyroids and ultimobranchial bodies or C cells) derive from the endodermal walls of the pharyngeal pouches. The thyroid arises from the floor of the pharynx and is considered homologous to the hypobranchial groove of the tunicates, Branchiostoma and cyclostomes. Also, the trachea and lungs are evaginations of the foregut and are thought to have evolved from the last pair of pharyngeal pouches. The operculum that in fishes and amphibians covers the gills is only a transient structure in mammals
6.3.3 A Peculiar Feature of the Human Development Is the Enormous Prenatal Growth of the Brain
In human development embryologists find unique features compared to other mammals. One peculiarity of the human embryo is the extremely slow cleavage. Compared to other vertebrates cleavage is slow in all mammalian species. The germ commences transcription of its (zygotic) genes shortly upon fertilization, a first wave starting at the two-cell stage. Transcription takes time. In subsequent development the mammalian human passes stages that can be understood only owing to its evolutionary past. Also in the outward appearance (Fig. 6.7) such traits become apparent, for instance gill pouches (Figs. 6.6, 6.7, 6.8, and 6.21), eyes positioned at lateral sides and for long time uncovered by a lid, arms and legs laterally protruding like in amphibians and reptiles, tail and milk lines (Fig. 6.9d).
A further, significant feature is the enormous growth of the cerebral hemispheres (Fig. 6.9), which for their part expand the still malleable head enormously. The diameter of the head, which principally increases in the frontal region, sets limits to length of time the child can stay in the womb of the mother. The birth canal cannot be expanded to any width, and it is indeed rather often too narrow, compelling surgical intervention (Caesarian delivery).
Another peculiarity occurs on the side of the mother. Especially in human mothers, in the uterus wall-less lacunae are formed, filled with maternal blood, that facilitate the exchange of substances between the mother and the child (see following section).
6.4 Interface Mother/Child: The Placenta
6.4.1 The Placenta Is the Organ by Which the Embryo Is Anchored and Through Which the Embryo Exchanges Substances with Its Mother
The developing child lies within a double-layered cyst. Both layers are of embryonic origin: the inner layer is the wall of the amniotic cavity and the outer layer is the chorion, largely a derivative of the trophoblast (Fig. 6.5 and 6.10).
In a region of the chorion, now called the placenta, the tiny primary chorionic villi are replaced by larger secondary villi, and eventually by tertiary villi. The branching, tree-shaped villi grow into cavities inside the uterine wall. The villi become vascularized: Two arteries (umbilical arteries) leave the body of the foetal child at the point of the future navel, traverse the amniotic cavity inside the umbilical cord, enter the villi, ramify and re-join to form the umbilical vein, which returns to the embryo to supply it with nutrients and oxygen. In exchange for maternal nutrients and oxygen, waste products such as CO2 and urea are transferred to the mother across the villi.
All this is of benefit to the child, indeed the mother has to pay a blood price because in birth the lacunae are not immediately closed. When a mother gives birth (Fig. 6.12) maternal blood gets lost. In other mammalian species, especially in non-primates, birth is not accompanied with much blood loss, and appears to be less painful.
6.4.2 There Are Further Dangers: The Immune System of the Mother Must Successfully Be Suppressed
From a pure biological view, and disregarding all joyful anticipation, the human embryo like any viviparous embryo might be considered a parasite that has to overcome the host’s defence. In fact, the removal of tissue barriers between mother and child is not entirely beneficial. The fetus comes into dangerous contact with the immune system of the mother; therefore, reasonable compromises must be found.
Although a child of its mother the fetus is not molecularly identical because half of the maternal genes have been singled out in the meiosis of the oocyte, and were replaced by paternal genes in fertilization. This applies also to the genes of the MHC (Major Histocompatibility Complex I, (in humans also known as human leukocyte antigen HLA). The complex codes for multimeric proteins that are exposed on the surface of somatic cells as an individual identity card. The barcode characterizing each individual encompasses a set of 14 different MHC proteins. Checking this card the immune system identifies foreign cells and tissues, for instance transplants, as being foreign. Foreign tissue is destroyed by cytotoxic T cells at the boundaries of transplants taken from a genetically different donor (heterologous transplants). The residual transplant is rejected. Why not the embryo?
One reason is that the cells of the trophoblast do not express the set of classical MHC molecules. On the other hand, cells lacking the classic MHC, as those of parasites, would evoke aggressive behaviour of the natural killer cells. To dampen the aggressive behaviour of the maternal natural killer cells the embryo expresses a set of peculiar embryonic HLA-G molecules. The mechanism by which these molecules locally shut down the immune system of the mother is currently under investigation.
But even when protection of the ‘transplant child’ against rejection by the maternal immune system is successful, the immunological coexistence of mother and child remains endangered; because a reliable barrier against antibodies, which are much smaller than immune cells, does not exist.
We refer to the Rhesus factor (Fig. 6.13). In the case of incompatibility it can lead to haemolytic disease of the fetus and newborn. This occurs when foetal Rh-positive erythrocytes find their way into the blood system of a Rh-negative mother; their immune system produces anti-Rh antibodies and these enter the foetal blood, passing the placental barrier. The antibodies release an immune response, the attacked red blood cells release haemoglobin and this causes a yellow colour of the skin (jaundice). Problems raised with chromosomal disorders in the child, such as trisomy 21 (Fig. 6.16) are discussed in Box 6.3.
Mother-Child-Chimeras
In this context a bewildering new discovery may be mentioned. In examining cells from diverse tissue samples of woman occasionally cells were found bearing a Y chromosome. All these women had once given birth to a boy, in some cases years ago. Apparently, foetal cells able to divide (putative stem cells) found their way through the placental barrier into the body of the mother, had been tolerated by her immune system on unknown grounds, and survived for years by self-renewal, as it is known from stem cells.
On the other hand in male offspring sporadically cells with XX chromosomes derived from the mother were found, testifying in retrospect to an imperfect placental barrier. Another source of XY cells in women and XX cells in men might have been blood stem cells once introduced by transfusion from donors belonging to the opposite sex.
6.4.3 Human Beings Live 9 Months Under Water, and During This Period the Unborn Child Has a Circulatory System Similar to That of a Fish
Teaching experience, gained in elementary and high schools as well, tells us that most children (and adults) are not aware that before birth they lived completely under water without any access to air, for 9 months. (As far as children in the womb hear voices or music, the voices and sounds are acoustically heavily distorted; therefore, some popular allegations by psychologists and the media about prenatal communication should be heard with due reservation).
The fluid in which we lived was the water of the amniotic cavity. Life under water and without atmospheric oxygen was possible because we got oxygen and disposed of carbon dioxide through the umbilical cord. With the increasing size of the fetus also the placenta grows so that efficient exchange is always possible.
The child is connected with the placenta by three main vessels. Two umbilical arteries leave the child at the site of the future navel, cross the amniotic cavity within the umbilical cord, and ramify in the placental villi into capillaries to facilitate exchange of substances. Enriched with oxygen the foetal blood returns to the embryo through the umbilical vein.
Thus, oxygen-loaded blood does not arrive from the lungs. Blood enriched with oxygen flows through the venous system into the undivided heart, is distributed in the body, collected and pumped back through the umbilical arteries to the villi of the placenta. The fetus has a circulatory system in the form of a single circle, like a fish, with the placental villi serving as gills. Because the lungs of the fetus do not yet function, but have to take over their vital task immediately after birth, the circulatory system must be prepared for a rapid conversion into a double-circle (body and lung) system. The solution to this serious technical problem is described in Chap. 17.
6.4.4 Pregnancy and Birth Are Attended with Great Dangers
In humans, the human blastocyst leaves the oviduct and settles itself into the uterine wall about one week after fertilization. The time point of nidation defines the start of pregnancy (gestation). The embryo is usually called fetus with beginning of the 10th week of pregnancy, that is 56 days after fertilization (about 10 days after Fig. 6.25d). At this age the heart beats already since a month. The embryo has attained a size of about 45 mm, is recognizable with ultrasound and begins to move its arms. However, prior to this stage 40–50 % of the implanted embryos perish as consequence of faulty development. Most disappear undetected with some blood during menses. Presumably a considerable part of mal-development is caused by the immune system of the mother.
The child is ready to be born after 38 weeks (266 days) of pregnancy. Birth represents another serious risk.
It is a risk to the mother: when the placental villi are pulled out from the uterus, blood flows out from the lacunae and is lost. The mother might be in danger of bleeding to death. Will the uterus succeed in sealing the large openings in time?
It is also a risk to the baby: in an instant, the lungs must be blown up and blood pumped through the lungs. At this time point a short circuit in the circulation (ductus botalli, see Fig. 17.10) must be closed, so that the pulmonary circulation is completely separated from the great body circulation. If it fails to close a congenital heart condition results, called patent ductus arteriosus. In spite of all these risks, the benefits of being harboured within a protecting and nourishing mother is worth these risks. The evolutionary success of the placental mammals, and of man in particular, is a testament to the value of this developmental innovation.
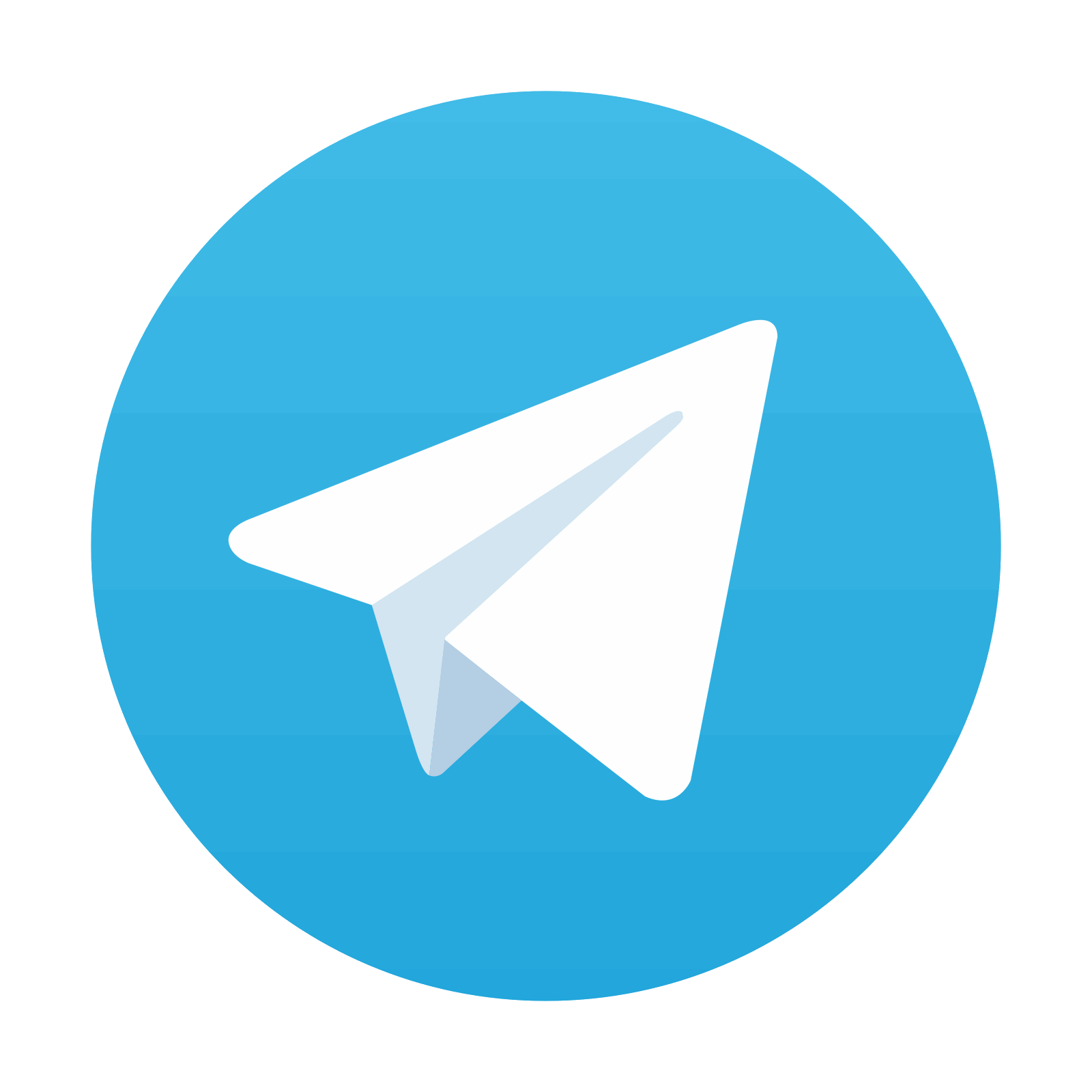
Stay updated, free articles. Join our Telegram channel

Full access? Get Clinical Tree
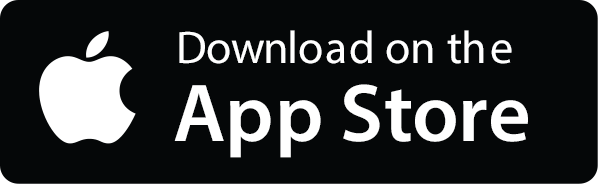
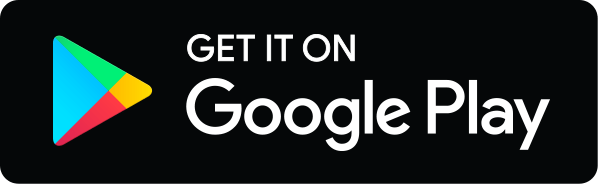