, Monika Hassel2 and Maura Grealy3
(1)
Centre of Organismal Studies, University of Heidelberg, Heidelberg, Germany
(2)
Spezielle Zoologie, Universität Marburg FB Biologie, Marburg, Germany
(3)
Pharmacology and Therapeutics, National University of Ireland Galway, Galway, Ireland
18.1 Continuous Renewal of Our Organism by Stem Cells
18.1.1 At the Very Least Each Organism Has to Renew Its Stock of Macromolecules
When we think of regeneration we usually imagine the reconstruction of lost body parts. However, this is only one among several regenerative events that organisms perform. Re-generation means generation anew. In this general sense, regeneration occurs at all levels of life, including the level of macromolecules. Proteins suffer irreversible alterations with time (denaturation) and must be replaced by newly synthesized ones. Without renewal, slow loss of function in essential enzymes would eventually result in the death of the cell. Experimental evidence suggests that renewal of the molecular inventory and resulting rejuvenation takes place routinely in the course of cell divisions. Division-mediated rejuvenation confers potential immortality to the single-celled protist. In contrast, terminally differentiated cells, incapable of dividing, will sooner or later die. We will investigate this observation and its cause in Chap. 21. Rejuvenation by mitotic division can be supplemented or replaced by rejuvenation through sexual reproduction.
18.1.2 Stem Cells Have Two Dominant Functions: They Enable Growth and Supply Replenishment for Used Up Cells
Stem cells are undifferentiated or partially differentiated cells capable of dividing; their descendants give rise to further stem cells on the one hand, and to cells determined to mature as differentiated cells on the other hand.
According to our inquiries the oldest scientific work in which the term stem cells (in German “Stammzellen”) can be found is a comprehensive treatise of the zoologist August Weismann (1883): “The origin of sexual cells in hydromedusae” (“Die Entstehung der Sexualzellen bei Hydromedusen”). In this treatise not only medusae (jellyfishes) are dealt with but also species in which polyps generate germ cells such as Hydractinia echinata (see Figs. 4.18 and 18.12). Actually the word “Stammzellen” is used for the first time in the section dealing with this species and it is used to designate the (putative) precursors of germ cells. At other places of the treatise primordial germ cells (“Ur-Keimzellen”) are defined as “stem cells of germ cells”. Weismann also speaks of “undifferentiated cells entailing several possibilities for differentiation” (“indifferenzirte Zellen, die mehrere Differenzirungs-Möglichkeiten in sich bergen”, p. 279). Besides stem cells and primordial germ cells (“Ur-Keimzellen”), also the terms germ line (“Keimbahn”) and the term pole cells (“Polzellen”), designating the primordial germ cells of Drosophila, were coined by Weismann. This hint to this widely unknown treatise should also be a hint that the principle of stem cells as source for germ cells and replenishment is evolutionarily very old – in fact as old as are multicellular organisms.
The issue of stem cells has led a shadowy existence in biology and medicine as well for many years, but is in the spotlight today and appeals to a broad public. In the multitude of information, with advertising appeal enclosed in therapeutic promises, the basic function of stem cells is often missed. Their primary function in the embryo to be founders of various cell lines only interests specialists in embryology. Only in the context of potential medical applications the term embryonic stem cells became familiar to a wide public. Yet, for actual therapeutic procedures and regenerative medicine post-embryonic, so called “adult” stem cells are of higher importance. To lay scientific foundations for discussing practical issues first two essential functions of fetal and post-embryonic stem cells may be outlined:
1.
Fetal and juvenile stem cells enable many organs to grow. Thus the enormous growth of the human central nervous system, in particular of the forebrain, is based on a layer of stem cells (called ependyma) lining the canal of the spinal cord and the ventricles of the forebrain. By symmetrical division the population of stem cells is increased. By asymmetrical division the stem cells also give rise to new neurons and glial cells (like the fish ependyma cells shown in Fig. 16.14). (Because with progressing development many embryonic stem cells lose stem cell characteristics it has been proposed to call those cells “founder cells” whereas the term “stem cell” should be reserved for those cells which retain stem cell characteristics life long. However world wide published literature cannot be rewritten, and so we follow current use.)
2.
Stem cells known as adult stem cells that retain stem cell characteristics life long enable many organs to renew and to regenerate. This will be specified in the following sections.
The renewal of the stock of cells has in older treatises been called “physiological” regeneration in contrast to “reparative” regeneration by which lost body parts are restored.
18.1.3 Short-Lived Cells Must Be Replaced by Newly Generated Cells; Replacement Is Provided for by Stem Cells Ready to Divide
As a rule, cells, tissues and organs generated in early embryogenesis must be enlarged in the course of larval and juvenile growth. But even after obvious growth has ceased, processes of proliferation persist in most organisms, in vertebrates as well as in Hydra, because many cells live for only a short time and must repeatedly be replaced by new ones.
Occasionally, even fully differentiated cells such as the hepatocytes in our liver retain the ability to divide. Normally hepatocytes are long-lived and divide slowly. However, in response to injury or poisoning they divide rapidly to replace damaged neighbours.
Most kinds of cells lose their mitotic potential in the course of terminal differentiation. Some even lose their nucleus, such as the keratinocytes of our skin or the erythrocytes of our blood. In all such cases a population of cells must be present that remains able to divide and to terminate differentiation after being multiplied. Such cells are defined as stem cells. When stem cells retain their ‘embryonic’ characteristics permanently, and can even be multiplied without limit in culture, they are designated immortal stem cells.
Stem cells in the adult organism are characterized by the following features:
1.
They serve the permanent self-renewal of a group of cells, of a tissue or organ.
2.
They retain the ability to divide, but usually divide only slowly and only on demand.
3.
As a rule their division is asymmetric. One daughter cell remains stem cell, the other undergoes differentiation. However, in several cases the proportion of 50 % stem cells, 50 % differentiating cells in the descendants is maintained only in the statistical average, while in other cases this apportionment is strictly observed. Frequently in asymmetric division the fate of the daughter cell sent onto a differentiation path is strictly determined; the cell is said to be committed to a task.
Dependent on whether the descendants of a stem cell can run all potential differentiation paths, or many different (but not all) paths, or only one single path, one speaks of totipotent (=omnipotent), pluripotent, multipotent or unipotent stem cells.
In the medical literature further criteria for distinction came into general use:
Totipotency (omnipotency) (Latin: totus = complete, omnis = all, potentia = power, potens = having power) is the ability of a single cell to give rise to a complete organism. In mammals this implies, that the cell in question can give rise to all cell types including germ cells and cells of the embryonic trophoblast and placenta, respectively. They have the ability to replicate in unlimited numbers without losing their total potency. In the human embryo totipotency is restricted to the cells of the cleaving embryo up to the 8-cell stage, testified by the existence of up to eight monozygotic super twins (octuplets). Cells of the germline, especially of the female germ line, are potentially totipotent until meiosis, thereafter oocytes are totipotent only if they regain a diploid set of chromosomes in fertilization (in mice one set maternally, and one set paternally imprinted).
In molecular terms totipotent cells and the cells of the germ line contain mRNA or the protein encoded by the gene vasa (Chap. 8) and express factors essential for maintenance totipotency such as NANOG (homeodomain protein), Oct-4 (transcription factor with POU domain), SOX-2 (transcription factor) and PIWI proteins (interacting with small piRNA).
Pluripotency (Latin: plus, pluris = greater, higher, more) refers to a progenitor or founder cell that has the potential to give rise to a multitude of cell types that belong to any of the three germ layers. Whether pluripotent cells are able to give rise to germ cells is not explicitly included in the definition but implicitly it is, because they derive from the inner cell mass of the blastocyst the cells of which are classified as pluripotent. In the mammalian/human embryo cells of the inner cell mass go on to create all the cells and tissues of the postnatal human body, but cells of the trophoblast, and hence of the placenta, are no longer included in the program. If removed and kept in culture cells of the inner cell mass are called embryonic stem cells or shortly ES cells or ESC (Fig. 18.1). Postnatal examples of pluripotent stem cells are the stem cells in the intestinal crypts (see Fig. 18.3).
Fig. 18.1
Mammalian blastocyst with inner cell mass, the source of embryonic stem cells
In terms of molecular biology, pluripotent cells are featured by the activity of particular pluripotency genes. Some genes such as Chd1 are responsible to keep the chromatin in a relaxed, accessible state. Of particular significance are the genes Nanog, Oct-4 and Sox-2. In cooperation with Sox-2, Oct-4 maintains a positive feed back loop of self activation (comparable to Fig. 12.20) and keeps the cell in the state of pluripotency. After cell division Oct-4 continues to be active in (at least) one of the daughter cells keeping it in the pluripotent state. More about pluripotency genes in Sect. 18.5.
Multipotency (Latin: multi = many) progenitor cells have the potential to give rise to cells from multiple, but a limited number of lineages that belong to one and the same classic germ layer. The classic paradigm of a multipotent stem cell is a hematopoetic stem cell of the bone marrow (see Fig. 18.6) – a blood stem cell that can develop into several types of blood cells but cannot develop into brain cells or liver cells.
Oligopotency (Greek: oligos = few) is a comparably seldom used expression to designate stem cells that give rise to only a few cell types, for example the lymphoid stem cells of the hematopoetic system. Also neural progenitor cells can be assigned to this category; they are kept in the incompletely differentiate state by the gene Sox-2 and ready to divide.
Unipotency is the restricted potency of a stem cell to give rise to only one cell type. The paradigm is the stem cells in the stratum germinativum of our skin that give rise to the horny cells (keratinocytes) of the epidermis (Fig. 18.2). Among the neural progenitor cells stem cells for a certain odorant class of the olfactory epithelium can be included in this category.
Fig. 18.2
Stem cells and carcinogenesis in the skin. The stem cells of the stratum germinativum produce descendants that differentiate into keratinocytes and are eventually sloughed off. In carcinomas, the differentiation is incomplete and cells derived from the stem cells continue to divide, thus forming a tumour (carcinoma). Transformed cells may dissociate from the tumour and enter the blood stream to establish colonies (metastases) in other places (after Alberts et al. 1994, redrawn and extended)
In practise this proposal for categorization is not strictly observed, and cannot be strictly applied to invertebrates and lower vertebrates. The definition, especially the distinction between pluripotency and multipotency, is tailor-made for placental mammals, as trophoblast and placenta are structures only found in placental mammals. In addition, linguistically the subdivision is not well founded as “pluri” and “multi” are almost synonymous (“pluri” = more, more than one, “multi” = many).
18.1.4 Unipotent Stem Cells Have Only One Option: Renewal of the Skin and the Muscle as Examples
During fetal and juvenile stages skin must be expanded; throughout the whole life skin must be renewed. The skin is exposed to stress caused by mechanical strain, chemical irritation, UV irradiation, and microbial attack. Unceasing growth and renewal are accomplished through the mitotic activity of unipotent stem cells. These lie upon the basal lamina; their descendants are displaced outwards, and on their outward journey they differentiate to keratinocytes, die and finally are sloughed off after 2–4 weeks (Fig. 18.2). Stem cells are able to continue dividing in contact with laminin of the basal lamina. Stimulating growth factors such as KGF (Keratinocyte Growth Factor), EGF (Epidermal Growth Factor) and TGFα (Transforming Growth Factor alpha) and inhibitory signal substances, such as the stress hormone adrenaline (epinephrine), participate in the control of skin proliferation. Figure 18.2 in addition points to the danger of stem cell-derived tissues converting into tumours. This aspect is discussed below in Sect. 18.4.3 and in a more general context in Chap. 19.
Skeletal muscles are renewed only in the event of particular need, for example when the muscle is injured. Residual myoblasts, called satellite cells, are left over from embryo development attached to bundles of muscle fibres. If needed, the quiescent satellite myoblasts are reactivated and multiplied. They fuse to myotubes and stop dividing. The myotubes fill their interior with bundles of actin and myosin, enlarge and take over the function of damaged muscle fibres.
18.1.5 Replenishment of Permanently Used Up Cells in the Gut and the Concept of Stem Cell Niches
Numerous protuberances in the small intestine, called villi, are composed of cells that move to the top of the villi, where they eventually disintegrate releasing many enzymes that supplement digestion. During their displacement, they serve the function of absorptive brush-border cells, of mucus-secreting goblet cells or of neurosecretory cells. When they arrive at the top of the villi, the cells sacrifice themselves to set free digestive enzymes; the residuals are shed into the lumen of the gut. Also the producers of the mucous layer, the goblet cells, are soon depleted and die. New cells are supplied by stem cells which lie in a protected position in the depths of crypts that descend into the intestinal wall in between the villi (Fig. 18.3a). As villi consist of several cell types their renewal depends on pluripotent stem cells. The term pluripotent is justified as the crypts contain not only derivatives of the endoderm but also neurosecretory cells that once in embryonic development derived from the neural crest cells (in traditional teachings assigned to the ectoderm).
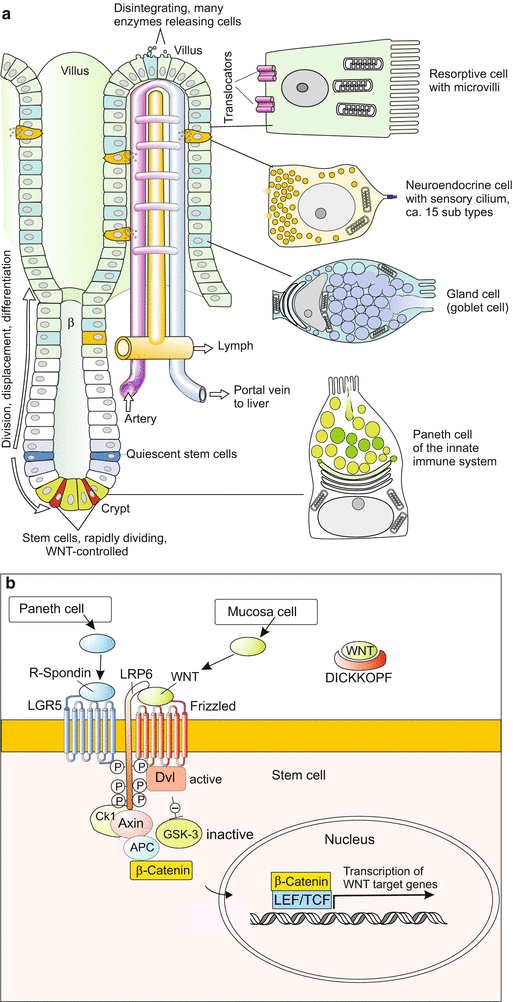
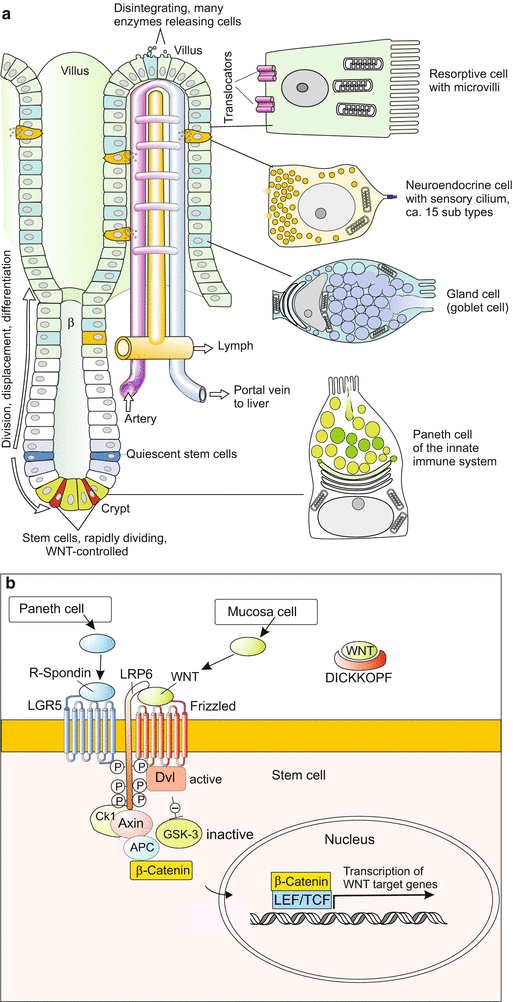
Fig. 18.3
(a) Renewal of the villi from stem cells in the small intestine. The multipotent stem cells are found not in the villi but at the bottom of adjacent crypts. The descendants of the stem cells are displaced in a distal direction, enter the villi and eventually are sloughed off at the tip of the villi. Sloughed off cells release digestive enzymes. Rapidly dividing stem cells between the Paneth cells are thought to supply cells for permanent renewal of the villi while quiescent stem cells are thought to be activated upon injury (illustration by WM, based on Merlos-Suarez et al. 2011). (b) Model of proliferation control. Activation of WNT target genes requires the arrival of two external signalling molecules: (1) R-spondin, the ligand of the LGR5 receptor, supplied by Paneth cells, and (2) WNT, the ligand of the Frizzled receptor, supplied by mucosa cells (Illustration by WM, based on Schuijers and Clevers 2012)
Investigations on the crypts corroborated a concept that is currently put forward in stem cell biology, the concept of stem cell niches in tissues and organs composed of several different cell types. The villi are composed of a mosaic-type pattern of cell types including two compartments containing stem cells. Their derivatives are considered to differentiate in response to local signals presented by the local niche. Also the stem cells themselves must be subjected to local influences that ensure proper balance between self-renewal and differentiation. In controlling stem cell proliferation at the basis of the crypts the WNT signalling system is involved, in cooperation with the related r-spondin signalling system that enhances the signal strength in the deepest compartment to accelerate cell division (Fig. 18.3b).
18.2 The Hematopoetic System: Formation and Renewal of Blood Cells
In humans, each second millions of blood cells are born to replace millions of dying cells. The bloodstream carries many cell types with different functions ranging from the storage of oxygen to the combat of infections and the production of antibodies. An astonishing finding is that all these diverse cells are the offspring of a single type of stem cell. The production and differentiation of blood cells is known as hematopoeisis (Greek: haimat = blood, poiein = to make).
18.2.1 Hematopoietic Stem Cells Emerge in Embryonic Blood Islands; They Emigrate Into Niches of the Bone Marrow Provided by Mesenchymal Stroma Cells Where They Survive Life-long
Hematopoiesis, the production of blood cells (Figs. 18.4 and 18.5) takes place during embryonic development in scattered mesodermal blood islands, where the first blood vessels are also formed from angiogenic founder cells (see Fig. 17.2). Accordingly, in these islands one finds
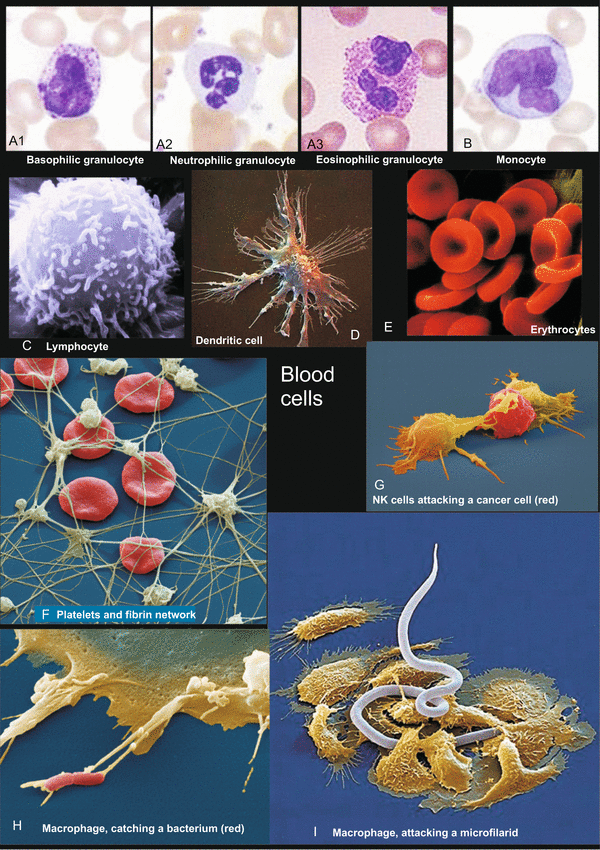
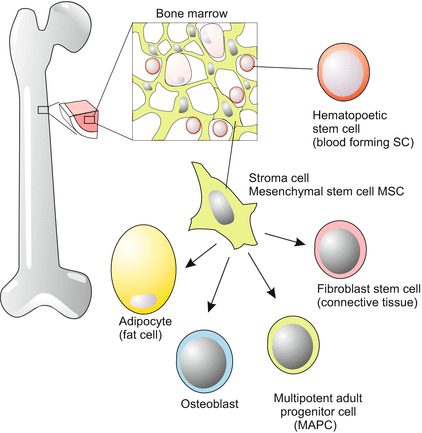
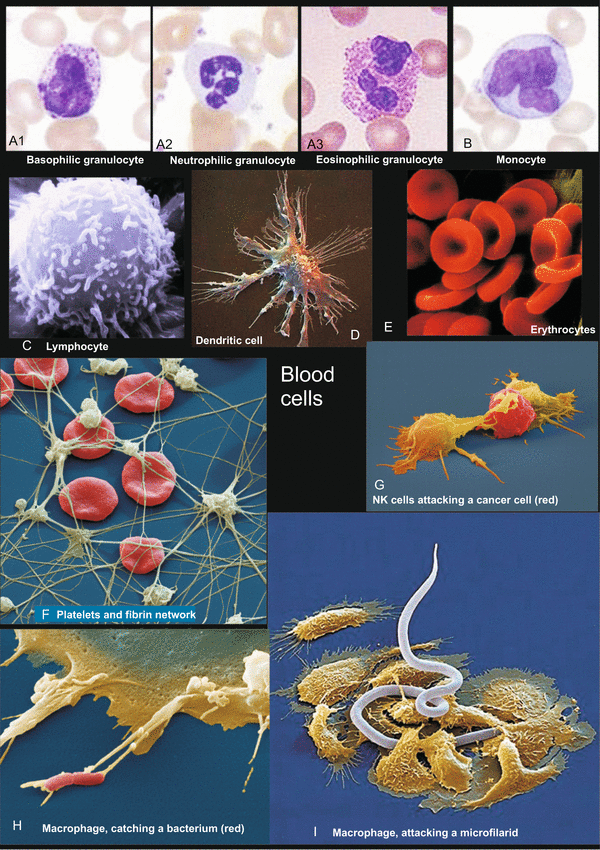
Fig. 18.4
(A–I) Cells of the human blood, illustration from Müller and Frings, Tier- und Humanphysiologie, Springer 2009. A, B Study collection of WM, C Rights reserved by Dr. Triche, National Cancer Institute; reproduced with his kind permission. F–I www.eyeofscience.de, purchased through Agentur-focus.de
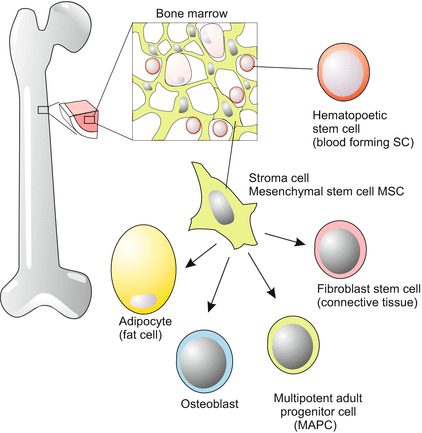
Fig. 18.5
Stem cells of the bone marrow with pluripotent stroma cells
hemangioblasts, founder cells which give rise to
angiogenic progenitor cells and
hematopoietic (blood-forming) stem cells (Fig. 18.6).
Fig. 18.6
Pedigree of the blood cells. Haematopoesis, the formation of blood cells, starts in the bone marrow from multipotent stem cells. Natural killer cells arise from lymphoid stem cells; the intermediate stepsare still a matter of debate. Dendritic cells are considered to be phenotypes of macrophages. Other types of dendritic cells are assumed to derive from lymphoid precursors
The first blood forming islands are found in the chick outside the blastodisc in the extraembryonic mesoderm covering the yellow yolk ball, and in humans in a corresponding position – the mesodermal layer covering the yolk sac (see Fig. 17.3). Later the stem cells migrate into the liver, the spleen and possibly the thymus. Ultimately, they populate the bone marrow. In the postnatal mammal significant numbers of hematopoietic stem cells are found only in the bone marrow. A surprisingly small number of multipotent stem cells (1 per 10,000 cells in the bone marrow) provide all of the blood cells.
A Glance at the Versatile Mesenchymal Stem Cells.
Adult stem cells occupy particular niches. This applies also for the bone marrow. In the bone marrow mesenchymal stroma cells provide a spongy three-dimensional network with many niches where hematopoietic stem cells reside life-long (Fig. 18.5). In the present scientific literature stroma cells usually are addressed with the abbreviation MSC, standing for mesenchymal stem cells. Surprisingly, stroma cells turned out to be stem cells themselves, and to be a highly versatile type of stem cells. They can give rise to connective tissue, cartilage cells, cross-striated and smooth muscle cells, heart muscle cells and endothelial cells of blood vessels. Whether and to what extent this wide potential is actually used for tissue renewal in the adult body must still be explored. The paradigm of multipotent stem cells in the bone marrow remains the hematopoietic stem cells.
History and Medical Importance.
Modern hematopoiesis research began with the search for means to protect against the disastrous effects of whole body X-irradiation. Mice whose blood forming system had been destroyed by high doses of X-rays could be rescued by injection of suspensions of bone marrow cells into the circulating blood. This was the pioneering experiment leading to procedures to cure leukaemia in humans. Malignant precursors are destroyed with high doses of X-rays or cytostatics and replaced by stem cells of a donor (genetic restrictions are discussed in Sect. 18.3).
However, stem cells only represent 0.01 % of the total mass of bone marrow, and much effort had to be invested to isolate and identify stem cells. Monoclonal antibodies were key tools, as they could be selected for their ability to recognize cell type or lineage-specific surface antigens.
A first indication as to whether a biopsy sample taken from the bone marrow contains stem cells, and how many, is provided by a procedure using a non-toxic dye (Hoechst 33342). Stem cells take up the dye as do other cells as well, but thereafter stem cells bleach by exporting the dye. For separation and isolation the cells removed from the bone marrow are dissociated and labelled with monoclonal antibodies raised to bind to characteristic surface components of the cells. The antibodies are coupled with fluorescent dyes, different antibodies are linked with dyes displaying different fluorescence spectra. This allows separation of the cells with a machine called FACS (Fluorescence-Activated Cell Sorting).
The surface structures recognized by the antibodies are generally designated CDx, with CD standing for “Cluster of Differentiation” and “x” standing for a number. For example progenitor cells of B-lymphocytes are characterized by the combinatorial label CD34 + CD19, T-cell progenitors are characterized by the surface structures CD34 + CD7 + CD5, whereas mature T-cells expose the surface structure CD3.
To isolate multipotent stem cells the distinguishing markers CD34 and CD90 are used. However, with these markers not only multipotent stem cells proper are isolated but predominantly lineage restricted descendants which can undergo only a limited number of cell divisions. As a consequence the balance between the diverse blood cell types can soon be disturbed. A newly discovered marker CD49f enables to isolate the tiny fraction of the true basal multipotent stem cells. Methods are being developed to replicate these cells in bioreactors and, if necessary, to cure genetic defects before they are reintroduced into the patient. Methods for correcting genetic defects such as homologous recombination are described in Chaps. 12 and 13.
18.2.2 The Turnover Is Huge: Up to 6 Million Erythrocytes Die Per Second and Must Be Replaced by New Ones
Without bone marrow stem cells, we would soon die because the life span of blood cells is restricted to hours, days or weeks. In humans, the half-life of
neutrophil granulocytes is 7–14 h, of
macrophages 5–7 days, for
erythrocytes is 120 days. This may appear quite long, but considering the amount of blood, from this value a turnover of 2–6 million erythrocytes per second has been estimated.
Aged and dying blood cells are sorted out in the lymphatic organs, notably the spleen, and removed by macrophages through phagocytosis. The process of sorting out is called sequestration.
Most cells of the adaptive immune system, namely lymphocytes are also short-lived. Among the immune cells only ‘memory cells’ (B-memory cells and T-memory cells) are long-lived.
18.2.3 Descendants of Stem Cells Committed to Become Blood Cells Are Multiplied by Restricted Numbers of Amplification Divisions
Stem Cells, Blasts, and Terminal Differentiation.
The rare multipotent stem cells of the bone marrow are endowed with the capability of dividing unceasingly. Part of their offspring remain multipotent stem cells (self renewal), while the remainder become committed to be progenitors (precursors) of more specialized cells with restricted developmental potential. The progenitor cells designated by the suffix –blast are still able to divide, but the number of rounds of divisions they can undergo is restricted. The limited reproduction of the progenitor cells serves to regulate the number of specialized blood cells on demand. One speaks of amplification divisions. After their last division, the cells undergo terminal differentiation. The number of permitted cell divisions is in many precursor cell types predetermined.
18.2.4 In Mammals a Decision Tree Leads to More Than Ten Categories of Blood Cells
The pedigrees of blood cells could be traced using engineered retroviral vectors carrying a selectable marker gene (neo, Chap. 12). The marker virus, like other retroviruses, inserts its own DNA into the genome of the host cells and is multiplied during cell divisions together with the host genome. The virus was engineered to remove genes that would enable it to spread from cell to cell. Bone marrow cells were first removed and infected in vitro with the retroviral vector, then transferred into lethally X-irradiated mice. Offspring of the retrovirus-marked donor cells were predominantly found in the spleen, but also in the bone marrow. Clusters of blood progenitor cells in the spleen are known as colony forming units (CFU). Such units were derived – statistically – from one founder cell, but could give rise to one or several types of blood cells, depending on the position of the founder cell in the tree-like pedigree.
Blood progenitor cells can be cultured in vitro, where various factors can be added and single cells and their offspring monitored. A few cells behave like multipotent stem cells, forming colonies of various types of granulocytes, macrophages and other cell types classified below as the ‘myeloid lineage’. Other isolated and seeded cells reveal more restricted potencies, generating only neutrophil granulocytes and macrophages. Still other seeded cells give rise to a single cell type such as neutrophil granulocytes or macrophages. From such studies, the whole pedigree has been reconstructed.
Starting from one basic multipotent stem cell (called Colony Forming Unit CFU-M, L) at least eleven types of blood cells are generated (Fig. 18.6):
A
The myeloid lineage supplies the following cell types:
1.
Erythrocytes that carry haemoglobin and, in mammals, lose their nucleus;
2.
Megacaryocytes. Unlike the other blood cells these “large nucleus cells” (literal translation) remain in the bone marrow, become very large and polyploid, and bud off numerous platelets into adjacent blood capillaries.
3.
Basophil granulocytes which emit alarm substances such as histamine, leukotrienes and prostaglandins during inflammatory responses.
4.
Eosinophil granulocytes which also fulfil tasks in inflammatory and allergic responses.
5.
Neutrophil granulocytes, sometimes also called microphages;
6.
Macrophages. Neutrophil granulocytes and macrophages together devour a large variety of infectious invaders and much waste produced in the body. Monocytes circulating in the blood are considered to be phenotypic modifications of the macrophages found in the interstitial spaces of tissues and in the lymphatic organs (lymph nodes, tonsils, and spleen). In the spleen the macrophages eat infectious bacteria as well as aged erythrocytes. In the liver they are known as stellate Kupffer’s cells. In their morphology these are intermediate between macrophages and dendritic cells.
7.
Myeloid dendritic cells are considered by several researchers to represent another phenotypic edition of macrophages or monocytes, respectively. (Another type of dendritic cells is thought to derive from the lymphoid stem cells which also give rise to B-cells and therefore is listed below under B). Dendritic cells share branched projections, the ‘dendrites’ that give them their name (but are structures distinct from the dendrites of neurons). Like macrophages dendritic cells sample the surrounding environment for pathogens such as viruses and bacteria. This is facilitated by pattern recognition receptors (PRRs) such as the toll-like receptors (TLRs). Like macrophages dendritic cells engulf the antigenic materials and insert peptide residuals into their MHC which subsequently is exposed on their surface. The MHC + antigen peptide complex is then presented to T-cells for examination, a process called antigen presentation. Besides macrophages and B lymphocytes, dendritic cells are the main population of the professional antigen-presenting cells APC. Dendritic cells are found in large numbers in the thymus; but are met in many other places as well, especially in tissues in contact with the external environment, such as the skin (where there is a specialized dendritic cell type called Langerhans cells) and the inner lining of the nose, lungs, stomach and intestine.
B
The lymphoid lineage supplies:
8.
Dendritic cell of putative lymphoid origin, among them plasmacytoid dendritic cells; they share characteristics of lymphocytes and dendritic cells.
9.
B-lymphocytes. In the form of plasma cells, the B-cells produce and release antibodies.
10.
T-lymphocytes are subdivided into several subtypes (T4 = T-helper cells; T5 = T-suppressor cells; T8 = cytotoxic T cells), and together constitute a system to discriminate between self and non-self and to adjust the mechanisms of defence to the current needs.
11.
Natural killer cells presumably also derive from the lymphoid stem cells and fulfil tasks in the innate immune response attacking viruses and, under certain conditions, also cancer cells. NK cells are unique, as they are endowed with the ability to recognize stressed cells in the absence of antibodies and antigen-presenting MHC recognition molecules, allowing for a much faster immune reaction.
The various non-erythrocytes are often summarized as leucocytes (white blood cells). When, as a result of disease, the blood contains too few erythrocytes physicians speak of anaemia. If the blood is enriched with too many but immature leucocytes physicians speak of leukaemia.
18.2.5 In the Development of Lymphocytes a Process of Irreversible Somatic Recombination Enables Production of a High Number of Diverse Antibodies
Recombination – the rearrangement of genes – is characteristic of sexual reproduction and takes place during meiosis. Surprisingly, a similar event occurs in the development of lymphocytes, when lymphoblasts combine their individual genetic programs for preparing the future production of antigen receptors or antibodies (which are liberated antigen receptors). This process, called somatic recombination, occurs at the ‘birth place’ of these cells: the bone marrow, the thymus or in lymph nodes (Fig. 18.7).
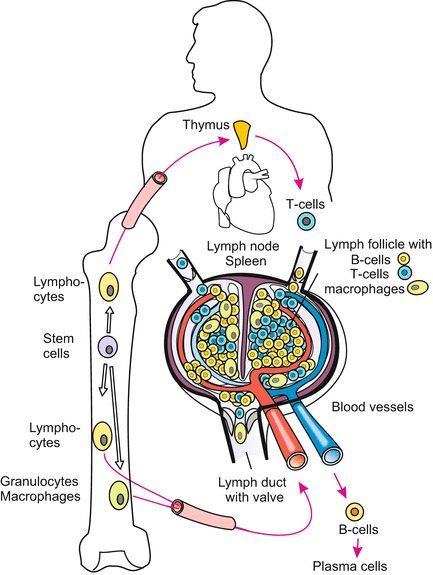
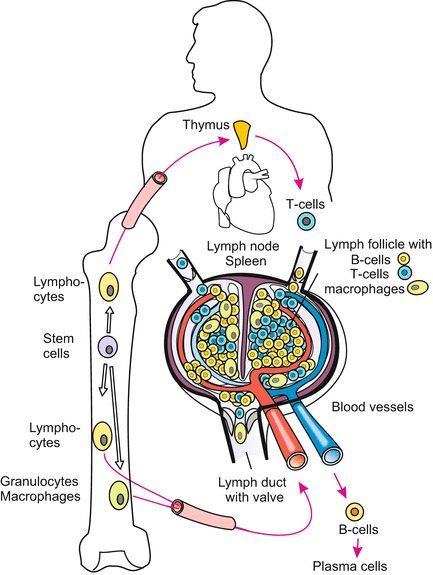
Fig. 18.7
Locations in which cells of the immune system are prepared for their task. The lymph node and thymus represent the entire lymphatic system
Lymphoblasts are genetic gamblers. They try their luck when they combine various short DNA sequences at random to program the variable region of their receptors or antibodies, increasing the chance of creating a combination appropriate to recognize and bind a future foreign antigen. In each B-cell or T-cell a different random combination is tried (Fig. 18.8). Subsequently, the T-lymphoblasts emigrate and settle in the thymus; the B-lymphoblasts remain in the bone marrow for some time. Wherever lymphoblasts stay, they learn to discriminate between self and non-self. A strict negative selection eliminates all those lymphoblasts whose receptors would bind molecules belonging to the self. The surviving cells are those that can bind only non-self molecules and are thus potentially capable of recognizing foreign substances. The surviving lymphocytes migrate through the body, colonize other places such as spleen and lymph nodes, and eventually serve as T-cells or antibody producing B-cells.
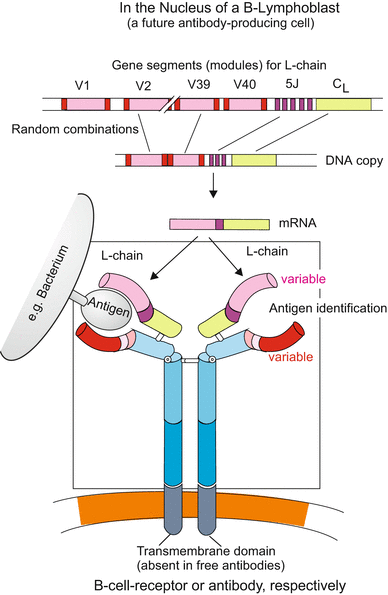
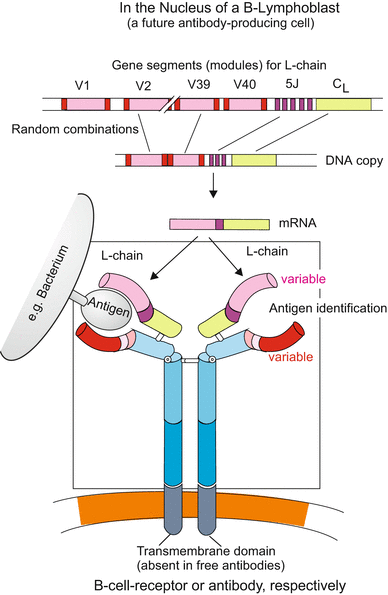
Fig. 18.8
Somatic recombination exemplified for the light chain (L-chain) of the B-cell receptor BCR. Without a transmembrane domain the BCR is released as an antibody. In programming the variable, antigen-binding domains of the BCR chromosomal gene segments are combined by somatic recombination according to the random principle. If by chance in a certain lymphoblast a combination is found that fits a given antigen closely this combination is retained, the lymphoblast multiplies by proliferation, and the combination is used to produce antibodies. The cell releasing mature antibodies is called a plasma cell
Somatic recombination, to date found only in lymphocytes, leads to an irreversible alteration in the genetic constitution of the cell.
18.2.6 In Lymphoblasts Cell Death Serves a Process of Learning
Programmed cell death of lymphoblasts is an essential part of the learning process that enables the immune system to discriminate between self and non-self. It may seem strange, but there is good reason for nature to use a strategy based on cell death. Millions of lymphoblasts, the future B-cells and T-cells of the immune system, rearrange DNA segments at random to produce a huge variety of antigen receptors or antibodies (antibodies are released antigen receptors). This strategy will inevitably not only generate lymphocytes whose receptors fit onto foreign antigens, but also self-reactive lymphocytes with receptors that bind self-molecules. This would be fatal if such lymphocytes were not quickly eliminated because the immune system is extremely aggressive.
Most of the self-reactive lymphocytes are eliminated in humans around the time of birth when antibodies taken up with mother’s milk no longer suffice. Due to this process of recombination followed by elimination, the immune system recognizes foreign substances rather reliably. How all these learning processes are accomplished in detail is subject to intensive investigations. Knowledge about these control mechanisms will be very important in understanding autoimmune diseases. Intolerance to self antigens in later life is thought to be due to continued production of self-reactive lymphocytes from stem cells.
Steroid hormones of the adrenal cortex, in particular cortisol and cortisone, promote the collective suicide of T-lymphocytes in the thymus. Birth and death in the immune system support the life of the entire cell community of our body.
18.2.7 Blood Cell Manufacture Is Controlled by Many Cytokines and Hormones
Many soluble, extracellular polypeptides influence types and numbers of blood cells manufactured. Many of these proteins have been isolated, identified and their genes cloned. A term designating such factors in general is cytokine. Examples of cytokines are the
Stem Cell Factor (SCF) that stimulates the stem cells of the myeloid pedigree to proliferate. Other cytokines do not manure the root of the tree but stimulate branching and growth of special branches. Such secondary cytokines are:
GM-CSF, the Granulocyte and Macrophage Colony Stimulating Factor,
G-CSF, the Granulocyte Stimulating Factor, and
M-CSF, the Macrophage Stimulating Factor.Stay updated, free articles. Join our Telegram channel
Full access? Get Clinical Tree
