Chapter 12 The Glaucomas
The glaucomas are a diverse group of diseases united only by the fact that intraocular pressure (IOP) is too high to permit the optic nerve and, in some species, the retina to function normally. Characteristic changes of glaucoma include disrupted axoplasmic flow in the optic nerve head, death of retinal ganglion cells and their axons, cupping of the optic disc, and visual impairment or blindness.
AQUEOUS PRODUCTION AND DRAINAGE
Aqueous exits the eye via several routes. In the conventional or traditional outflow route aqueous humor passes from the posterior chamber, through the pupil, and into the anterior chamber. Because of temperature differences between the iris and cornea, thermal convection currents occur in the anterior chamber, with aqueous near the iris rising and aqueous near the cornea falling. This is one reason cells and particulate matter in the anterior chamber may settle on the inferior corneal endothelial surface. Aqueous humor then leaves the anterior chamber by passing between the pectinate ligaments to enter the ciliary cleft, which contains the trabecular meshwork (Figure 12-1). After filtering between the beams of the spongelike meshwork, aqueous crosses through the endothelial cell membranes of the meshwork to enter a series of radially oriented, blood-free collecting vessels collectively called the angular aqueous plexus. From there it enters an interconnected set of blood/aqueous-filled vessels (the scleral venous plexus) before draining either anteriorly via the episcleral and conjunctival veins or posteriorly into the vortex venous system and into the systemic venous circulation (Figure 12-2). Contraction of smooth muscle fibers of the ciliary muscle that insert into the trabecular meshwork are probably capable of increasing drainage of aqueous from the eye by enlarging the spaces in the trabecular meshwork. In most species the majority of aqueous humor (about 50% in horses, 85% in dogs, and 97% in cats) leaves the eye via the traditional outflow route.
The remainder of the aqueous humor leaves the eye via the uveoscleral pathway (see Figure 12-1). In this route aqueous humor passes through the root of the iris and interstitial spaces of the ciliary muscle to reach the supraciliary space (between the ciliary body and the sclera) or the suprachoroidal space (between the choroid and the sclera). From these locations aqueous humor may pass through the sclera into the orbit either via pores in the sclera where blood vessels and nerves enter the eye or between the scleral collagen fibers themselves. Outflow via this route may substantially increase in certain disease states and in response to certain antiglaucoma drugs, such as the prostaglandin derivatives.
Balancing Aqueous Production and Outflow
IOP is the result of a delicate balance between production and outflow of aqueous humor (Figure 12-3). In glaucoma both production and outflow are altered. Usually a large percentage of the outflow pathway (perhaps as much as 80% to 90%) needs to be impaired before IOP starts to rise. If the outflow system is impaired to the point that IOP begins to increase, the eye usually attempts to compensate by reducing the passive production of aqueous humor. Active secretion, however, typically continues at a relatively normal rate, perhaps because if it did not, the avascular tissues of the eye that rely on aqueous humor for their nutrition would starve. Because the glaucomatous eye is functioning on a greatly diminished percentage of its normal levels of aqueous humor outflow and production, and because it has exhausted its usual compensatory pathways, pathologic processes or drugs that alter production or outflow only a small amount can have dramatic effects on IOP. This characteristic is one reason that glaucomatous eyes are typically more responsive to antiglaucoma drugs than normotensive eyes, but it also explains why IOP can rapidly rise to very high levels in a matter of 1 to 2 hours in some patients.
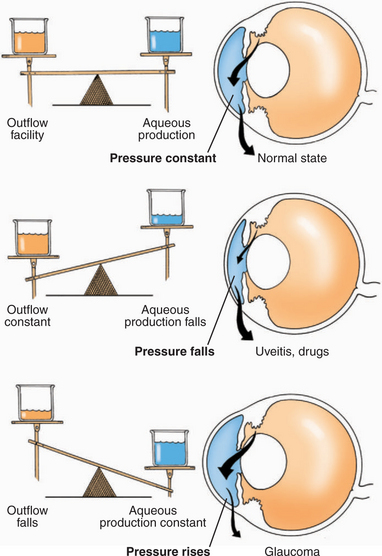
Figure 12-3 Common alterations in aqueous production and outflow facility and their effects on intraocular pressure.
DIAGNOSTIC METHODS
Tonometry
Measurement of and normal values for IOP are discussed in Chapter 5. It is suggested that the reader refer to that discussion before proceeding with this chapter. Despite its disadvantages, the most economical instrument in general veterinary practice is the Schi∅︀tz tonometer with the human conversion tables. Surprisingly, dog-specific conversion tables for the Schi∅︀tz tonometer do not agree as well with the more accurate applanation and rebound tonometers, and dog specific tables should not be used to convert Schi∅︀tz scale readings to IOP estimates in dogs or cats. Two handheld tonometers that are more accurate and easier to use than the Schi∅︀tz instrument are the Tono-Pen applanation tonometer and the TonoVet rebound tonometer. The ability to perform tonometry is essential to every veterinarian engaged in small animal practice. Tonometry minimizes the chances of making an important or even catastrophic error in diagnosis.
Gonioscopy
Gonioscopy is a very useful technique for examining the iridocorneal (filtration) angle and managing glaucoma. It is discussed in detail in Chapter 5. Gonioscopy allows the clinician to differentiate between open-angle and closed-angle glaucoma, to estimate the severity of the obstruction of the iridocorneal angle, and to evaluate the response to therapy (Figure 12-4). It does, however, require considerable practice to recognize the many normal variations and hence gonioscopy tends to be performed almost exclusively by veterinary ophthalmologists. Examples of gonioscopic findings are shown in Figures 12-5 to 12-11.
CLINICAL SIGNS
The clinical signs of glaucoma in the dog are summarized in Figure 12-12. The signs present in a particular animal depend on the duration, intensity, and cause of the pressure elevation. In general the most obvious signs are associated with end-stage disease in which there is no hope of preserving vision. In the very early stages of glaucoma, in which there is a chance of preserving vision, the eye may appear normal and IOP may or may not be elevated. In some patients there is only a history of intermittent episcleral injection (especially in the evening) that spontaneously resolves, and IOP is normal on examination in the office. Glaucoma may be detected in these animals only by performing tonometry when the eye is red or, occasionally, by repeatedly measuring IOP over 24 hours. In other patients the eye may appear to be essentially normal and the only finding is increased IOP on tonometry. In these patients it is essential to differentiate glaucoma from increased IOP measurements associated with an uncooperative patient, technical problems with measuring IOP (excessive tension on the eyelids, a collar that is too tight, compression of the jugular veins during restraint, etc.), and malfunction of the instrument. Specialist assistance may be required to make the diagnosis of glaucoma in its early stages.
Engorged Episcleral Vessels
Engorgement of episcleral veins (see Figure 12-2, B) is one of the more common signs of increased IOP. Episcleral engorgement arises because the increased IOP reduces flow through the ciliary body to the vortex veins, and increased flow passes forward via anastomosing episcleral veins at the limbus (see Figure 12-1). Conjunctival capillaries may also be engorged, but usually to a lesser degree. Episcleral vascular engorgement is a sign of intraocular disease (anterior uveitis or glaucoma) and may be differentiated from superficial conjunctival vessel engorgement (which indicates ocular surface disease) by the following features:
Corneal/Scleral Changes
Edema
Increased IOP impairs the function of the corneal endothelium, resulting in corneal edema. Typically the entire cornea is diffusely edematous in glaucoma, and the edema can be quite dramatic in acute glaucoma when IOP is very high (Figure 12-13). In advanced cases subepithelial bullae may form, which can lead to corneal ulceration if they rupture. In chronic glaucoma both superficial and deep vascularization, scarring, and pigmentation are common.
Buphthalmos and Descemet’s Streaks
Chronic increases in IOP results in stretching of the cornea and sclera and enlargement of the globe (buphthalmos; Figure 12-14). Buphthalmos may be especially pronounced in young animals and in shar-peis, who have a more easily distended cornea and sclera than most adult dogs. Buphthalmic eyes are almost invariably blind, although limited vision may be retained for a while in some puppies and shar-peis. Buphthalmos is irreversible even if the pressure is later reduced, although a variety of surgical procedures are available to restore a cosmetically acceptable appearance.
By the time severe stretching has occurred, atrophy of the ciliary body may have reduced the IOP to normal and pain may be lessened. As the cornea stretches, linear ruptures in Descemet’s membrane, called Descemet’s streaks (Haab’s striae), may occur (Figure 12-15).
Fixed Dilated Pupil
As IOP rises, the pupillary constrictor muscle becomes ischemic and the pupil dilates to midrange or larger (Figure 12-16). A dilated pupil, along with episcleral injection and pain, may be among the first signs noticed by the owner. Mydriasis is not an invariable sign of glaucoma— the pupil may be normal in mild IOP elevations, and miosis may be present in uveitis-induced glaucoma. In these latter cases, a careful examination is necessary to distinguish glaucoma from uveitis, and it is possible for both to be present in the same eye. In chronic glaucoma, or when IOP is acutely markedly elevated, the direct and consensual pupillary light reflexes are usually greatly impaired or absent. The longer glaucoma remains unresolved, the greater the chance that peripheral anterior synechiae will form and permanently block the drainage angle by fixing the peripheral iris in position.
Lens Changes
Lens luxation or subluxation may be recognized from the following signs:
The recognition of how the final state was reached is important in determining which combination of therapeutic methods is required. History and signalment are critical factors in differentiating between these various possibilities. In all three pathways the lens may be displaced anteriorly or posteriorly or may be in the plane of the iris (either superiorly or inferiorly). An aphakic crescent is formed when the lens zonules have broken for a portion of the circumference of the lens, and it is possible to visualize the tapetal reflex through a crescent-shaped space between the lens equator and the pupillary border (Figure 12-17). After luxation the lens frequently, but not invariably, becomes cataractous.
Primary lens luxation, as occurs in terriers and certain other breeds (Box 12-1), may result in pupillary block with acute elevations in IOP. The presence of vitreous strands in the anterior chamber in the absence of buphthalmos suggests primary lens luxation. In these animals the lens may be completely luxated or only partially luxated (subluxation), and usually the lens is not cataractous until it becomes luxated (Figures 12-18 and 12-19).
Box 12-1 Inherited and breed predisposition to lens luxation in dogs
Modified from Gelatt KN, Brooks DE (1999): The canine glaucomas, in Gelatt KN (editor): Veterinary Ophthalmology, 3rd ed. Lippincott Williams & Wilkins, Philadelphia.
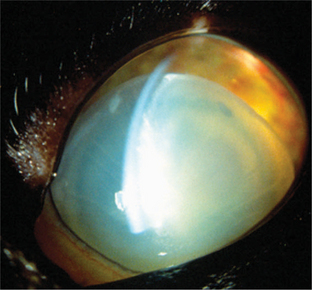
Figure 12-19 Complete anterior lens luxation associated with chronic uveitis in a cat. Secondary glaucoma was also present.
Primary glaucoma tends to occur in middle-aged to somewhat older dogs of certain breeds (Box 12-2), and the lens subluxation or luxation does not occur until the globe has become buphthalmic and the lens zonules are stretched beyond the breaking point (secondary luxation). Similarly, primary cataract formation in a wide variety of breeds is frequently followed by lens luxation and glaucoma. Lens-induced uveitis from a secondarily luxated lens that has become cataractous from elevated IOP, and decreased IOP from the uveitis further complicate diagnosis and treatment. Thus the combination of glaucoma, cataract, and lens luxation in any particular eye may occur through several mechanisms and may be associated with a variety of IOP values at any given moment.
Box 12-2 Breeds of dog most commonly affected with different types of glaucoma
From Miller PE (1995): Glaucoma, in Bonagura JD (editor): Kirk’s Current Veterinary Therapy XII: Small Animal Practice. Saunders, Philadelphia. Breeds are listed in descending order of frequency as recorded by the Veterinary Medical Data Base over a 20-year period.
Fundus Changes
Impaired Vision
Loss of some or all vision is a common sequela of glaucoma. In the early stages peripheral vision may be lost (Figure 12-20), and it is difficult, if not impossible, to detect these changes in most animals. Complete vision loss can occur in a very short period (hours to a day) if the increase in IOP is very high, or over a period of weeks to months if the pressure increase is more insidious. Preservation of vision depends on control of IOP.
Optic Disc Cupping
Cupping, or posterior bowing of the optic disc through the lamina cribrosa, is the hallmark of glaucoma. Retinal nerve fibers run parallel to the surface of the retina and then turn 90 degrees to enter the multilayered, fenestrated meshwork of the lamina cribrosa before exiting the eye. Glial cells, blood vessels, and collagen beams form variably sized pores through which the optic nerve fibers pass. When IOP rises the scleral lamina cribrosa bows posteriorly, distorting the alignment of the pores and compressing the optic nerve fibers. Although this change may initially be so subtle as to not be detected ophthalmoscopically, it is sufficient to mechanically interfere with axonal axoplasmic flow and also probably with blood supply to the optic nerve head. Very large increases in IOP may also interfere with blood flow to the choroid and produce vision loss through ischemic damage to the photoreceptors and outer retinal layers. In acute glaucoma the optic disc may appear swollen in response to ischemia. Within a day or two the increased pressure may cause the disc to appear pale and compressed. As ganglion cell axons die, optic nerve head tissue is lost and pressure forces the lamina cribrosa outward (Figures 12-21 to 12-23). This change indicates irreversible damage to the optic nerve. Wallerian degeneration of the optic nerve follows (Figure 12-24).
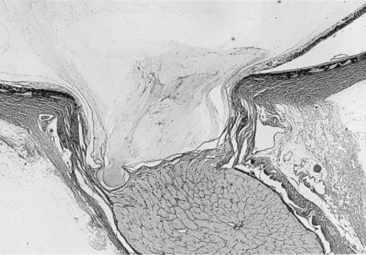
Figure 12-21 Cupping of the optic disc with loss of tissue anterior to the lamina cribrosa, which is bowing posteriorly.
(From Slatter D [2003]: Textbook of Small Animal Surgery, 3rd ed. Saunders, Philadelphia.)
Retinal Degeneration
In advanced glaucoma, profound retinal atrophy with increased tapetal reflectivity occurs together with attenuation or complete loss of retinal vessels, atrophy of the pigment epithelium in the nontapetal fundus, and optic atrophy (grayish-white appearance; Figure 12-25). These findings are also present in advanced progressive retinal degeneration (progressive retinal atrophy). In progressive retinal degeneration the other signs of glaucoma are lacking, the disease is usually bilateral, the optic disc is not cupped, and differential diagnosis may be determined by the breed of dog and lack of other clinical signs of glaucoma. Ophthalmoscopically visible retinal and optic nerve lesions of glaucoma are irreversible.
Elevation of IOP decreases blood flow in the choroid, resulting in ischemia. This ischemia can be demonstrated functionally by depressed electroretinograms, and in some patients it is possible to visualize wedge-shaped defects in the retina that correspond to pressure-induced infarction of the choroidal blood supply (Figure 12-26). Early in glaucoma, if the pressure elevation is acute and very large, the photoreceptors in the retina undergo necrosis. In the next few days they begin to die by apoptosis as well. Ophthalmoscopically the cell death is seen as increased tapetal reflectivity. As in any other severe retinal atrophy, the condition is irreversible.
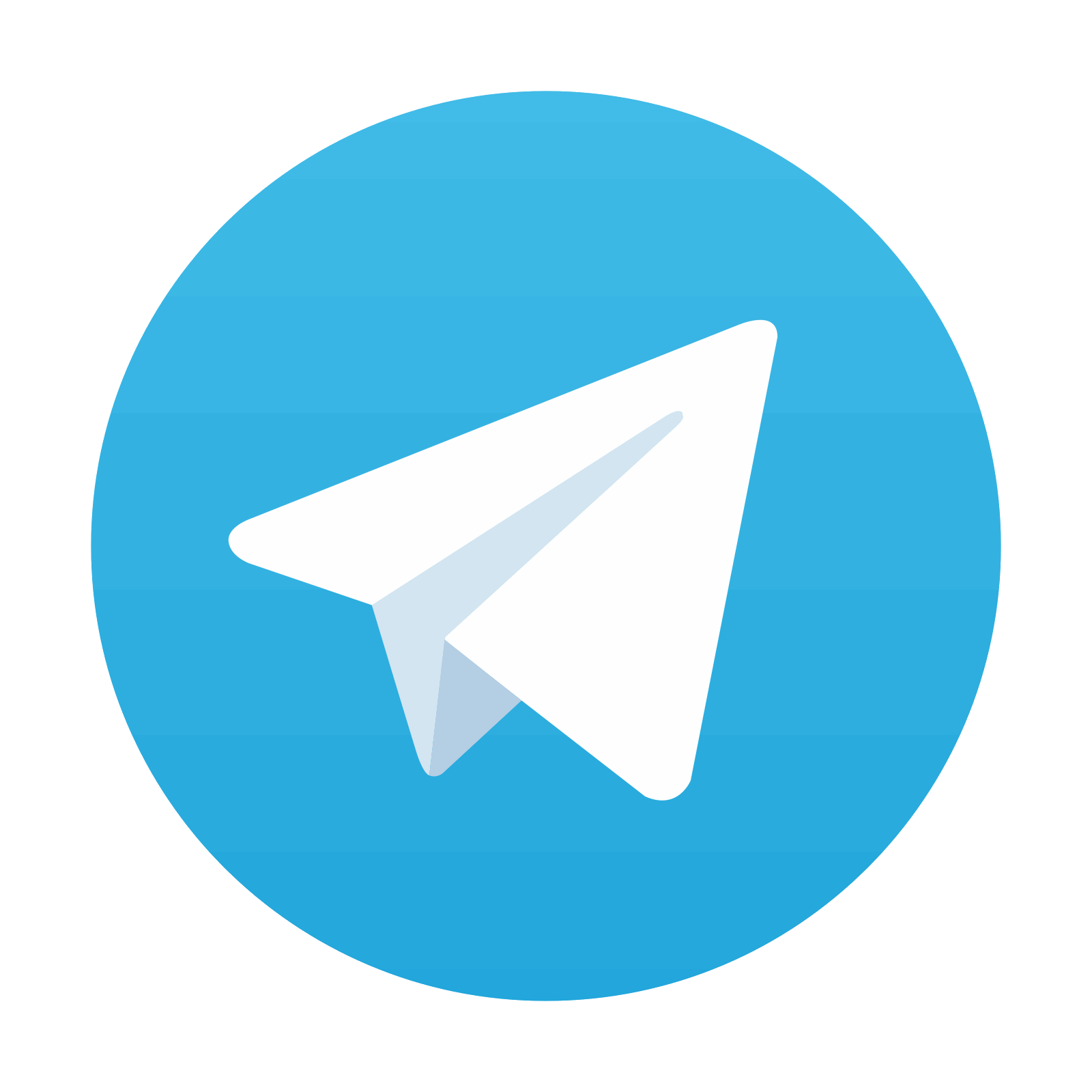
Stay updated, free articles. Join our Telegram channel

Full access? Get Clinical Tree
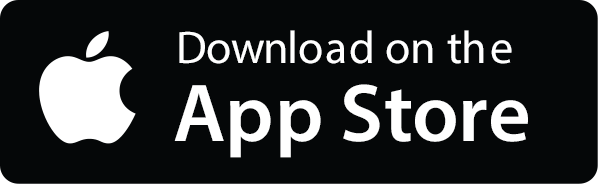
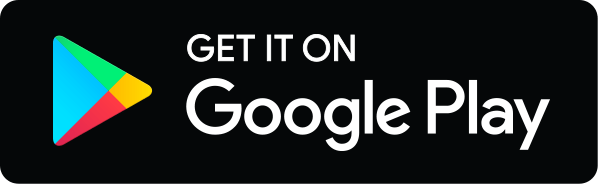