2. The erythrocytes: morphology and response to disease Erythrocytes, commonly referred to as ‘red blood cells’ (RBC), are the predominant cellular component of blood and fulfil several vital functions. Notably, they transport oxygen from the lungs to other tissues and transport carbon dioxide from tissues to the lungs, but they also participate in the regulation of acid–base status by buffering hydrogen ions. Erythrocytes typically comprise 30–50% of total blood volume and are present at a concentration of approximately 5 × 1012 cells/L, approximately 1000-fold greater than the concentration of other cell types (i.e. leukocytes and platelets). Mature mammalian erythrocytes are anucleated (Briggs, 1936) and simplistically consist of a cell membrane enclosing the cytosol. Erythrocytes are 7–9 µm in diameter in most species (Ponder et al., 1928; Harboe and Schrumpf, 1953; Ralston, 1985; Benga et al., 1992) (Table 2.1), although some species, such as the long-nosed potoroo (Moore and Gillespie, 1968) and Gould’s wattled bat (Cleland, 1915), have been reported to have smaller cells. The predominant shape is a biconcave disc (‘discocyte’) for most species of Australian mammals, although variant shapes may comprise a small proportion of cells. The concentration, size and morphology of erythrocytes may influence their function and these characteristics, in health and in response to disease, are discussed throughout the course of this chapter. The structure, function and pathophysiology of the cell membrane of animal erythrocytes have been well reviewed (Smith, 1987). The erythrocyte membrane consists of a lipid bilayer and a cytoskeleton. The lipid bilayer is composed of nearly equal parts of lipid and protein, with the main lipids being cholesterol and phospholipids. Cholesterol is equally distributed between the two layers of the lipid bilayer whereas the other lipids are unequally distributed. Glycolipids, phosphatidylcholine and sphingomyelin are located in the outer half of the bilayer and phosphatidylethanolamine and phosphatidylserine occur in the inner layer (facing the cytoplasm). Most of the proteins span the width of the membrane and are usually divided into an internal hydrophilic section, a membrane-spanning hydrophobic section and an external hydrophilic section with attached carbohydrate groups. The phospholipid composition of the membrane of erythrocytes has been quantified for several species of Australian marsupials (Nouri-Sorkhabi et al., 1996): the common wombat, black-striped wallaby, southern brown bandicoot and Tammar wallaby have a similar membrane phospholipid composition and the eastern grey kangaroo has greater amounts of sphingomyelin and phosphatidylcholine (~72%), and lesser amounts of phosphatidylethanolamine, phosphatidylinositol and phosphatidylserine, (~28%). The significance of these differences has not been studied. Table 2.1 Diameter of erythrocytes from selected Australian mammals
INTRODUCTION
ERYTHROCYTE CELL MEMBRANE
Species | Erythrocyte diameter (µm) | Reference |
Platypus | 4.8–7.2 | |
Echidna | 6.6–7 | |
Red kangaroo | 8.35 ± 0.17 | |
Red-necked wallaby | 8.05 ± 0.23 | |
Southern swamp wallaby | 8.57 ± 0.23 | |
Parma wallaby | 8.00 ± 0.29 | |
Whiptail wallaby | 8.38 ± 0.27 | |
Tammar wallaby | 7.76 ± 0.18 | |
Long-nosed potoroo | 5.5 ± 0.92 | |
Goodfellow’s tree-kangaroo | 7.30 ± 0.21 | |
Koala | 8.6 ± 0.31 | |
Tasmanian devil | 6.77 ± 0.29 | |
Northern brown bandicoot | 7.12 ± 0.22 | |
Bilby | 7.01 ± 0.36 | |
Gould’s wattled bat | 5.5–6.5 | |
Blue whale | 7.7 ± 0.7 | |
Humpback whale | 8.2 ± 0.7 |
The cytoskeleton consists of several proteins, including spectrin, ankyrin, actin and protein 4.1, which form a filamentous network under the lipid bilayer. They interact with the integral membrane proteins to maintain the integrity, and allow flexibility, of the membrane, which is important in determining the shape of erythrocytes. The composition of the cytoskeleton of erythrocytes from the red kangaroo, western grey kangaroo, euro, red-necked wallaby, Tammar wallaby and common brushtail possum was found to be similar (Ralston, 1985), comprising the proteins ankyrin (band 2.1), spec-trin (band 3), band (protein) 4.1 and actin (band 4.2).
OSMOTIC RESISTANCE/FRAGILITY OF ERYTHROCYTES
The cell membrane of erythrocytes is flexible but not elastic, and consequently the cell will rupture if the amount of water taken into the cell exceeds its critical volume. The resistance of the erythrocyte to haemolysis is measured by subjecting it to solutions containing decreasing concentrations of sodium chloride ions. Maximum resistance is the concentration at which all erythrocytes have been haemolysed. Minimum resistance is the concentration at which haemolysis is first detected. The mean corpuscular fragility (MCF) is used to express the concentration of sodium and chloride ions at which haemolysis of 50% of erythrocytes occurs. Erythrocytes from eastern grey and red kangaroos had similar osmotic resistance/fragility (MCF, 130 mosmol/kg) and were more resistant to osmotic stresses than ovine erythrocytes (MCF, 220 mosmol/kg) (Buffenstein et al., 2001), indicating an adaptation to an arid environment. The MCF in that study was not affected by prior water restriction.
BLOOD GROUPS
Blood groups are protein, glycolipid or oligosaccharide structures on the outer surface of the erythrocyte membrane. Clinically these are relevant in transfusion medicine where they may provoke an immune response by the recipient of the ‘foreign’ erythrocytes that results in the destruction of the transfused cells and initiates further inflammatory events. These cellular structures are largely undetermined for most species of Australian mammals. The only published study compared the frequency of several erythrocytic antigens in dingoes and breeds of dogs and found that dingoes possessed many of the blood groups present in the domestic dogs (Symons and Bell, 1992).
ERYTHROCYTE CYTOSOL
The cytosol of erythrocytes contains many substances, most importantly electrolytes and proteins. Electrolytes are involved in the osmotic regulation of the cell and control of cell volume with sodium and potassium ions typically having the largest effect. The concentrations of these electrolytes vary between species; for example, Tammar wallabies (101.2 ± 4.8 mmol/L) and red-necked pademelons (103.5 ± 1.4 mmol/L) have a much greater potassium concentration than Parma wallabies (10.5 ±0.5 mmol/L)(Agar et al., 1986). Interestingly, the intraerythrocytic concentration of electrolytes may also vary between populations of the same species, with common brushtail possums having either ‘high sodium, low potassium’ or ‘low sodium, high potassium’ erythrocytes (Barker, 1958). The significance of these differences within and between species has not been determined.
Also present in the cytosol are proteins, such as the oxygen-binding protein haemoglobin and a number of enzymes that are involved with carbohydrate metabolism, ATP production, the formation of antioxidants and the reduction of methaemoglobin (discussed in Chapter 3). Haemoglobin is the most abundant protein in the cytosol and is composed of a tetramer of globin proteins (two alpha and two beta chains), each with an attached haeme. The positioning of the haeme group allows the carriage of oxygen molecules. Haeme is a planar molecule composed of tetra-pyrrole protoporphyrin IX with a central ferrous molecule. Its production is a complex physiological process involving many sequential steps that occur, at varying stages, within mitochondria and in the cytoplasm (Harvey, 1997; Kaneko, 2000). The synthesis of the globin chains occurs within ribosomes in the cytoplasm of the erythrocyte. The production of haeme and globin are finely coordinated so that little or no ‘free’ product is present in the erythrocyte. The oxygen-binding characteristics of haemoglobin have been studied for many mammals and are discussed in Chapter 3.
Both the genetic and amino acid sequences of the globin chains have been studied for many species of Australian animals. The structure of globin is generally not of clinical interest, but has been used to determine the phylogenetic relationship of monotremes and marsupials to aid the understanding of the evolution of these animals and is considered here for completeness. The cDNA sequences for the alpha-and beta-globin genes from the eastern quoll (Wainwright and Hope, 1985) and for the beta-globin genes of the fat-tailed dunnart (Cooper et al., 1996) and short-beaked echidna have been reported (Lee et al., 1999). The structure of the beta-globin chain of haemoglobin has been most studied in macropodids and partial amino acid sequences have been determined for a number of species (Thompson et al., 1969; Air and Thompson, 1969; Beard and Thompson, 1970; Air et al., 1971; Thompson and Air, 1971; Beard and Thompson, 1971). Comparison of the amino acid sequence has shown that the eastern grey and red kangaroos differ by only a single substitution, whereas the long-nosed potoroo differs from the eastern grey kangaroo by 16 amino acids. The amino acid sequences of the globin chains of haemoglobin have also been reported for other Australian mammals, including the platypus (Whittaker and Thompson, 1974), short-beaked echidna (Whittaker et al., 1972), grey-headed flying-fox and black flying-fox (Kleinschmidt et al., 1988), ghost bat (Singer et al., 1991), common sheathtail-bat (Singer et al., 1992), Weddell seal (Lin et al., 1989), Australian sea-lion (Ikehara et al., 1996) bottlenose dolphin (Kleinschmidt and Braunitzer, 1983) and sperm whale (Abbasi et al., 1986).
SHAPE, STRUCTURE AND ULTRASTRUCTURE OF TYPICAL (‘NORMAL’) ERYTHROCYTES
The morphology of erythrocytes can be assessed by light microscopy and transmission electron and scanning electron microscopy, with light microscopy being the most commonly available method for clinical assessment. Under light microscopy, erythrocytes from Australian native mammals are anucleated (Briggs, 1936) and typically round with a biconcave shape (Ralston, 1985) (Plate 12). This shape results in a typical appearance; namely, an eosinophilic (haemoglobin-containing) zone around the margin of the cell that comprises approximately two-thirds of the cell and a central pale region (containing a lesser amount of haemoglobin). In most species there is a small amount of variation in the size of erythrocytes (i.e. anisocytosis, see later). A few species, most commonly cetaceans, possess erythrocytes that are uniconcave or non-concave in shape and when viewed by light microscopy these cells have a reduced or absent central pallor (Plate 13).
Ultrastructurally, erythrocytes have a multi-layered cell membrane, including an external glycoprotein layer, a phospholipid bilayer and an internal fibrillar protein layer that ‘limits’ the cells. The cytosol contains predominantly haemoglobin, which has a homogeneous to finely granular, moderately electron-dense appearance (Bessis, 1973) (Plate 14), and essentially no organelles are observed in mature cells. Immature, anucleated erythrocytes contain electron-dense aggregates that represent aggregated polyribosomes. Many arte-factual changes may occur in the shape of the erythrocyte during fixation, processing or sectioning.
Scanning electron microscopy is an important method for studying the shape of erythrocytes (Plates 15,16). One study of the morphology of erythrocytes from 11 species of marsupials showed that all had discocytes as the predominant cell shape (Benga et al., 1992). Mature erythrocytes have a smooth membrane, whereas immature, anucleated erythrocytes have characteristic infoldings, depressions and numerous pits in the surface of the cell membrane (Fernandez and Grindem, 2000).
MORPHOLOGICAL VARIATION OF ERYTHROCYTES IN BLOOD FILMS
As previously stated, the predominant shape of erythrocytes for most species of mammals is discoid, but a number of other cellular shapes and structures may also be identified in a blood film. The presence of significant numbers of these variant cells may represent physiological, pathological or artefactual changes. The following section describes cells with altered morphology, the circumstances under which they may be formed and their significance to the assessment of the health of the animal. Many of these morphological abnormalities of erythrocytes have been reported in domestic animals, but few have been specifically reported in Australian native mammals. Small numbers of variant cells may be found in most samples with diligent examination of the blood film and an occasional variant cell should not be over-interpreted. However, the presence of large numbers of variant cells may indicate a disease and should not be ignored.
Anisocytosis
Variation in the size of erythrocytes is termed anisocytosis. Some anisocytosis exists in healthy individuals. Anisocytosis may be visually evident on a blood film or may be identified from an increased ‘red blood cell distribution width’ (RDW) reported by automated haematology analysers. Pathologic anisocytosis may result from increased numbers of larger cells (macrocytes), increased numbers of smaller cells (microcytes) or increased numbers of both. Microcytes are small erythrocytes that maintain some central pallor. In marsupials, occasional microcytes may be noted in healthy individuals and the clinical significance of these cells remains uncertain. Increased numbers of microcytes may occur with some nutritional deficiencies, such as iron and copper deficiency. Macrocytes are erythrocytes that are larger than ‘normal’. The term should strictly be used for mature cells with a well-defined central depression (Bessis, 1973), but is commonly used to describe immature cells such as polychromatophilic erythrocytes.
Polychromatophilic erythrocytes and reticulocytes
Polychromatophilic erythrocytes represent the penultimate stage of erythrocyte maturation and in blood films stained with Romanowsky stains, they appear slightly larger and more basophilic in colour than mature erythrocytes (Plate 17). The colouration is because of the presence of remnant cytoplasmic ribosomal RNA. The extent of the polychromasia reflects the number of polychromatophilic erythrocytes in the peripheral blood, which in healthy animals varies with species and age, immature animals typically having more than adults.
The same penultimate stage of erythrocyte development, when stained with supra-vital stains, such as new methylene blue, show aggregations of RNA (‘reticulum’) and the cells are termed ‘reticulocytes’ (Plate 18). Ultrastructurally, these aggregates are composed of polyribosomes. The number of reticulocytes present in the peripheral blood of healthy adults varies between species. In some species, such as the platypus, there are ‘none’ (Whittington and Grant, 1983). In contrast, many reticulocytes have been seen in the blood of fattailed and stripe-faced dunnarts (400–800 × 109/L) (Haynes and Skidmore, 1991) and an intermediate number in samples from eastern quolls (130 40 × 109/L) (Melrose et al., 1987). The percentage of reticulocytes (of total erythrocytes) is commonly reported (0–1.0% in red-necked wallabies (Hawkey et al., 1982),0.8–2.4% in koalas (Booth and Blanshard, 1999), 0.2–0.6% in yearling common brushtail possums (Presidente and Correa 1981) and 0.1–1.8% in common wombats (Presidente, 1979b)), but may be influenced by a relative lack of mature cells (in anaemic animals), and whenever possible the absolute reticulocyte concentration should be used for clinical interpretation.
Reticulocyte concentration is also found to change with age in most species. Immature animals have increased concentrations of reticulocytes compared with adults, because of increased erythropoietic activity (Jain, 1986; Jain, 1993). In laboratory rats, ‘all’ erythrocytes at birth are reticulocytes. The proportion of reticulocytes subsequently decreases to 50% of erythrocytes at 10 days of age and adult levels are achieved by 40 days of age (Orten and Smith, 1934). To the author’s knowledge this has not been determined for any species of Australian mammals.
Determination of the reticulocyte concentration of an anaemic animal is the best way to quantify the response of the bone marrow to the anaemia in most species of animals (see the section on anaemia).
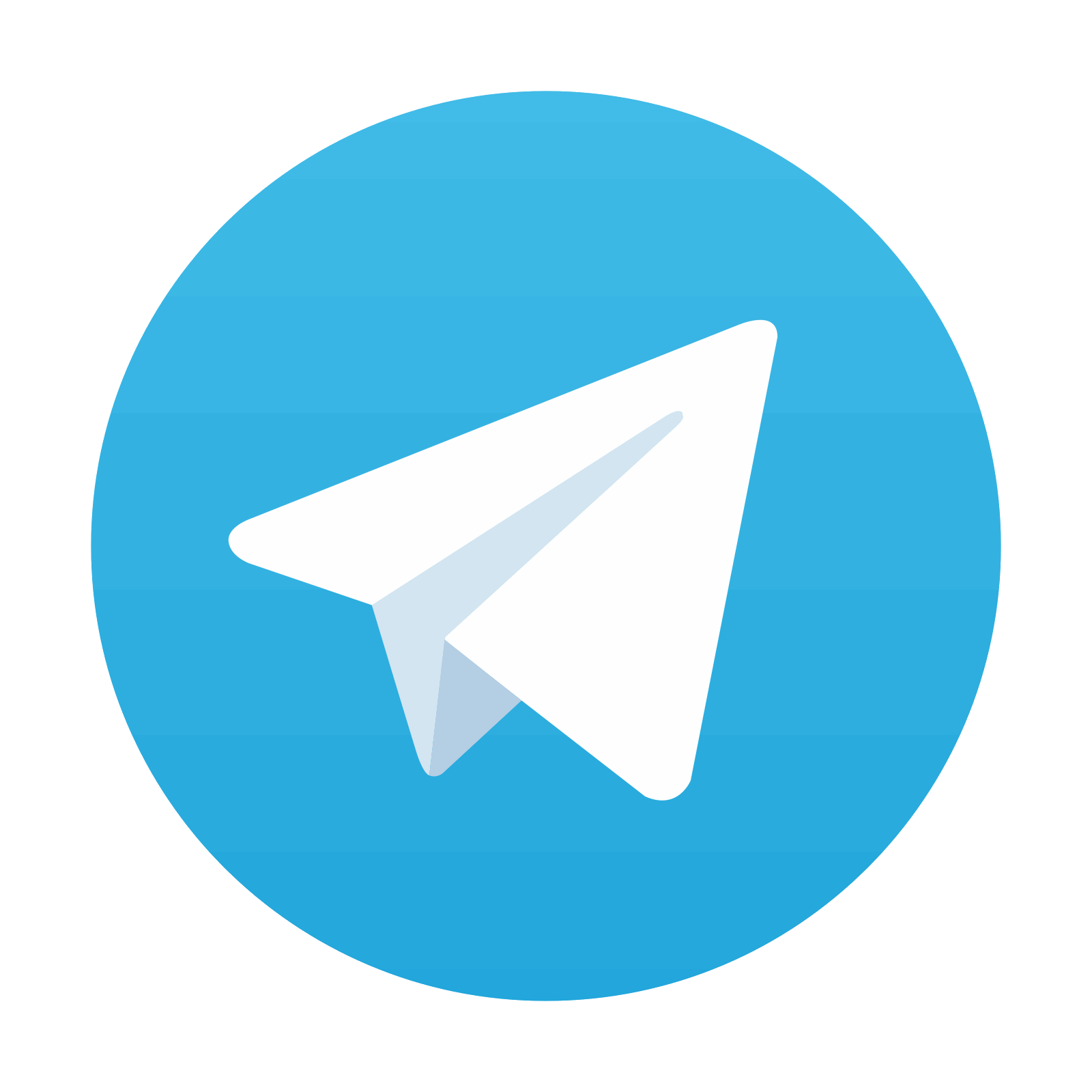
Stay updated, free articles. Join our Telegram channel

Full access? Get Clinical Tree
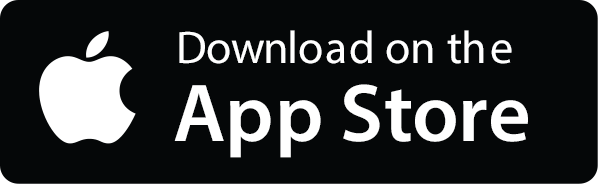
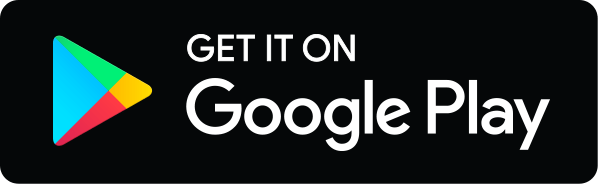