3. Biochemistry of erythrocytes The primary roles of erythrocytes, during their relatively long lifespan, is to transport oxygen and carbon dioxide and buffer hydrogen ions, despite the physical and chemical insults to which they are exposed. Mature erythrocytes have limited synthetic and repair capacities, but to effectively function they must have biochemical pathways that generate enough energy to support cellular function and allow haemoglobin to be maintained in a state that can effectively bind oxygen. Anaerobic metabolism of carbohydrate provides for the relatively low energy needs of erythrocytes without consuming the oxygen that is being transported. Erythrocyte carbohydrate metabolism has been adapted to provide two additional pathways through which metabolic intermediates can be shunted or diverted as the need arises for the products of these shunts. The products of these shunts are 2,3-diphosphoglycerate (2,3DPG) and reduced nicotinamide adenine dinucleotide phosphate (NADPH). The 2,3DPG functions to control the affinity of haemoglobin for oxygen and NADPH is a major source of the reducing equivalents needed to protect haemoglobin against oxidative injury. The biochemical characteristics of erythrocytes are sufficiently distinct from their morphological characteristics to warrant special consideration. This chapter provides an overview of the metabolic pathways of erythrocytes in humans and domestic animals and considers the known characteristics of Australian mammals. The measurement of enzymes and metabolites within erythrocytes requires specialised biochemical techniques and consequently is rarely undertaken as part of a routine clinical haematological investigation. When performed, these assays are typically part of an investigation into a familial haemolytic disorder or more commonly, used as research tools to provide an important insight into the function of erythrocytes of Australian mammals. The general principles used to assess the amounts of enzymes and metabolic intermediates within erythrocytes are outlined in the following section and those interested in obtaining further information should consult specialist texts (e.g. Beutler, 1975). Analytes that may be of interest include intra-erythrocytic enzymes, reduced glutathione (GSH), adenosine-5-triphosphate (ATP) and 2,3DPG. After collection, the blood for analysis must be mixed with an anticoagulant, such as heparin or acid-citrate-dextrose, to prevent clotting (Agar et al., 1975; Beutler, 1975; Melrose et al., 1990). Additional samples that have been mixed with EDTA are used for measurement of routine haematological analytes, such as haematocrit, erythrocyte concentration and haemoglobin concentration. Intra-erythrocytic enzymes are typically measured in a haemolysate prepared after erythrocytes have been washed to remove plasma, leukocytes and platelets (Agar et al., 1975; Beutler, 1975) and the results are typically reported as ‘international units per gram of haemoglobin’ (IU/gHb). The amount of reduced glutathione is also measured in the haemolysate using the 5,5’-Dithiobis-(2-nitrobenzoic acid) (DTNB) method (Agar and Stephens, 1975; Beutler, 1975) and is reported as ‘micromoles per gram of haemoglobin’ (µmol/gHb). A semi-automated method for the measurement of ATP and 2,3DPG in the supernatant, obtained by the addition of an equal volume of trichloroacetic acid (80 g/L) to whole blood or washed erythrocytes in isotonic saline (to precipitate protein) and centrifugation of the mixture (Godwin et al., 1983), has been used for measurement of these substances in a wide range of Australian mammals. The results of ATP and DPG assays are typically reported as µmol/gHb. Erythrocytes require energy in the form of ATP for the maintenance of shape and deformability, phosphorylation of membrane phospholipids and proteins, active membrane transport of various molecules, synthesis of nucleotides by the salvage pathway and synthesis of GSH. In addition to energy, erythrocytes require ‘reducing power’ in the form of nicotinamide adenine dinucleotide phosphate (NADP) and NADPH to counteract the oxidative processes in their oxygen-rich environment. The requirement for ATP is met by metabolism of glucose. Comparative aspects The metabolic needs of erythrocytes are met by anaerobic metabolism of glucose in humans and most domestic animal species. Cell membranes are poorly permeable to glucose and in most cells glucose is transported via transmembrane proteins called GLUT transporters (Bell et al., 1990). These differ in their affinity for glucose (as measured by their Michaelis-Menten constant (Km)) and their sensitivity to control by insulin. In humans, erythrocytes have the GLUT-1 transporter, which has a high affinity for glucose (low Km) and is not sensitive to control by insulin. This means that erythrocytes have a steady supply of glucose, even at very low glucose concentrations and regardless of insulin levels. The erythrocytes of domestic animals vary in their permeability to glucose (Harvey, 2000). In general, foetal erythrocytes have higher rates of glucose transport than adults. Adult pigs lack GLUT transporters, but most domestic species appear to be intermediate in their ability to transport glucose in comparison with humans. Erythrocytes do not consume the oxygen that they transport because mature erythrocytes lack the mitochondria needed for aerobic metabolism. A net of two molecules of ATP can be produced for every molecule of glucose that is metabolised to lactate through anaerobic glycolysis (Embden-Meyerhof pathway). This provides erythrocytes with a rapid, but inefficient source of ATP because anaerobic glycolysis releases only approximately 7% of the energy generated when glucose is completely oxidised to water and carbon dioxide. The rate-limiting steps are similar to those of glycolysis in other cell types, with phosphofructokinase-1 being the major regulatory enzyme controlling the rate of glycolysis. The other rate limiting enzymes are hexokinase and pyruvatekinase. As glycolysis is the only source of ATP in the mature erythrocyte, genetic defects that affect either the level or activity of any of the glycolytic enzymes have significant effects on ATP production and therefore on erythrocyte survival. The steps in anaerobic glycolysis are summarised in Figure 3.1 Characteristics of Australian mammals The concentrations of glycolytic metabolites and activities of various glycolytic enzymes have been measured in many marsupials and the monotremes. These are reviewed by Agar et al. (2000) and include the Tammar wallaby, red-necked pademelon and Parma wallaby (Agar et al., 1986), bilby and rufous hare-wallaby (Agar and Godwin, 1991), koala and whiptail wallaby (Baker et al., 1995), burrowing bettong, common wallaroos from both the mainland and Barrow Island (Billiards et al., 1999), eastern quoll (Melrose et al., 1990), spectacled hare-wallaby (Agar and Spencer, 1993a), Tasmanian devil, Tasmanian pademelon and common brushtail possum (Isaacks et al., 1984), and bridled nailtail wallaby, Proserpine rock-wallaby, allied rock-wallaby, black-striped wallaby, eastern grey kangaroo and red kangaroo (Parkinson et al., 1995). Lactate is the end-product of anaerobic glycolysis and the rate of lactate production when erythrocytes are incubated with autologous plasma, glucose or other potential substrates has been measured in a wide range of Australian native species. When erythrocytes are incubated in autologous plasma rather than an individual substrate, lactate production should reflect the potential rate of production in vivo whereas incubation with an individual substrate should help to define which compounds may be contributing to lactate production in vivo. The rate of lactate production by erythrocytes incubated in autologous plasma is very variable, ranging from0.64 mmol/mL RBC per hour for the bridled nailtail wallaby up to 16.61 mmol/mL RBC per hour for the black-striped wallaby (Parkinson et al., 1995). The common wombat also has a low rate of production (Agar et al., 2000), whereas the spectacled hare-wallaby (Agar and Spencer, 1993a), grey kangaroo and Proserpine rock-wallaby (Parkinson et al., 1995) have high rates of lactate production when incubated with plasma. All marsupial species and monotremes tested have been able to metabolise glucose to lactate and glucose appears to be a good substrate for erythrocyte energy production in native animals. The highest rate of lactate production from glucose occurs in erythrocytes of the brown antechinus (Agar and Godwin, 1991), with the bilby (Agar and Godwin, 1991) and koala (Baker et al., 1995) also having relatively high rates of production. Although most species had lower rates of lactate production when incubated with glucose than with autologous plasma, the bridled nailtail wallaby had a significantly higher rate when incubated with glucose alone than when incubated with plasma, suggesting the presence of an inhibiting factor in plasma. Many species are also able to efficiently use mannose and fructose as substrates for glycolysis, but only the bilby and rufous hare-wallaby were able to produce more than 2 µmol/mL RBC per hour when incubated with galactose (Agar and Godwin, 1991; Agar et al., 2000). When incubated with either dihydroxyacetone or glyceraldehyde, the erythrocytes of the brown antechinus and bilby both produce lactate at high rates (Agar and Godwin, 1991) in comparison with other species tested (Agar et al
INTRODUCTION
MEASUREMENT OF SUBSTANCES WITHIN ERYTHROCYTES
ERYTHROCYTE CARBOHYDRATE METABOLISM
Stay updated, free articles. Join our Telegram channel

Full access? Get Clinical Tree
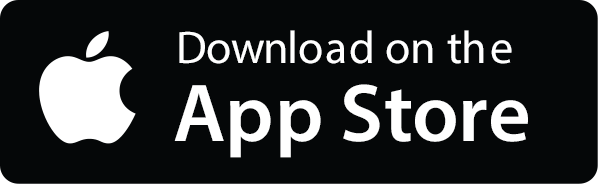
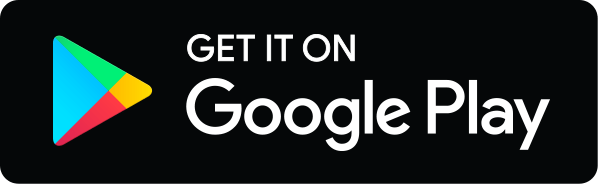