Chapter 7 Supportive Care of the Poisoned Patient
The poisoned patient will almost always require broad-based monitoring and supportive care to guard against direct and collateral organ damage induced by poison or intoxication. Such patients may have respiratory, cardiovascular, neurological, gastrointestinal, renal, hepatic, or hematologic abnormalities and these organ systems are the focus of the monitoring and support endeavors. There are, in addition, important nursing care issues relative to the management of recumbent and comatose animals.
RESPIRATORY
Breathing rate, rhythm, nature, and effort
Breathing rate can vary widely in normal animals and, except for extreme variations, is of limited value as a respiratory monitor. A change in breathing rate, however, is a sensitive indicator of a change in the underlying status of the patient. Bradypnea may be a sign of respiratory center depression secondary to intracranial or extracranial disease. The underlying cause should be identified and treated if possible. Arrhythmic breathing patterns are usually indicative of a problem within the central pattern generator in the brainstem, and such a finding suggests a poor prognosis. A weak breathing effort may be caused by central or peripheral neurological impairment or neuromuscular dysfunction, depending upon the specific poison or toxin. Bradypneic, arrhythmic, and weak breathing patterns may be associated with hypoventilation and such patients should be intubated and ventilated. Tachypnea is usually a sign of an underlying problem, such as hypoxemia, hypercapnia, hyperthermia, hypotension, sepsis, pain, or opioid administration, and seldom needs to be treated specifically.
Ventilometry
Ventilation volume can be estimated by visual observation of the chest wall and can be measured by ventilometry. Normal tidal volume ranges between about 8 and 20 mL/kg. Normal total minute ventilation ranges between about 150 and 250 mL/kg/min for dogs. PaCO2 defines alveolar minute ventilation and the measured minute ventilation should be appropriate. A large minute ventilation in combination with a normal (or high PaCO2) is indicative of dead space ventilation, as might occur in hypovolemia and pulmonary thromboembolism.
Hypoventilation
Hypoventilation is treated by endotracheal intubation and positive pressure ventilation.
Diseased lungs are stiffer (less compliant) than normal lungs, and are therefore much more difficult to ventilate. It is a common finding that the above guidelines are insufficient to adequately ventilate a patient with diffuse pulmonary parenchymal disease. To improve ventilation, the proximal airway pressure could be increased in a step fashion up to 60 cm H2O (or to the limit of the ventilator) and the ventilatory cycle rate could be increased in a step fashion up to 60 breaths per minute. The tidal volume should be decreased in an animal with diffuse lung disease because such lungs have a reduced vital capacity; a normal tidal volume could overdistend the reduced number of remaining lung units. Protective lung strategies currently aim for very small tidal volumes (i.e., 4 to 6 mL/kg). If oxygenation must be improved: (1) the inspired oxygen could be increased up to 100% for short periods of time or up to 60% for prolonged periods of time; (2) positive end-expiratory pressure (PEEP) can be added or increased (up to 20 cm H2O [PEEP increases transpulmonary pressure and functional residual capacity, and keeps small airways and alveoli open during the expiratory phase and improves ventilation and oxygenation. PEEP also minimizes the repetitive collapse and reopening of small airways, a process that contributes to ventilator-induced injury]); or (3) the inspiratory time or the inspiratory plateau could be increased. The inspiratory/expiratory [I/E] ratio must allow sufficient time for exhalation of all of the last breath, otherwise air trapping will occur.
Partial pressure of oxygen (PO2)
Venous PO2 reflects tissue PO2 and bears no correlation to PaO2. Mixed or central venous PO2 ranges between 40 and 50 mm Hg. Values below 30 mm Hg may be caused by anything that decreases the delivery of oxygen to the tissues (e.g., hypoxemia, anemia, low cardiac output, vasoconstriction); values above 60 mm Hg suggest reduced tissue uptake of oxygen (e.g., septic shock, metabolic poisons, shunting). Venous blood for such evaluations must be taken from a central vein, such as the jugular, anterior vena cava, or pulmonary artery; peripheral venous PO2 values are highly variable and difficult to interpret.
Hemoglobin saturation with oxygen
Hemoglobin saturation (SO2) measures the percent saturation of the hemoglobin and is related to PO2 by a sigmoid curve. In general a PO2 of 100 mm Hg is equivalent to an SO2 of about 98%; a PO2 of 80 mm Hg is equivalent to an SO2 of about 95%; a PO2 of 60 mm Hg is equivalent to an SO2 of about 90%; and a PO2 of 40 mm Hg is equivalent to an SO2 of about 75% (Tables 7-1 and 7-2, and Figure 7-1). The clinical information derived from the measurement of arterial SO2 (SaO2) is similar to that obtained from a PaO2 measurement in that they both are a measure of the ability of the lung to deliver oxygen to the blood.
Pulse oximeters attach to a patient externally (tongue, lips, tail, toenail). For most clinical purposes, most pulse oximeters are sufficiently accurate approximations of oxyhemoglobin saturation; accuracy should be verified by an in vitro standard if possible. The accuracy of a pulse oximeter is greatest within the range of 80% and 95%, and is determined by the accuracy of the empirical formulas that are programmed into the instrument. Tissue, venous and capillary blood, nonpulsatile arterial blood, and skin pigment also absorb light. A pulse oximeter must differentiate this background absorption from that of pulsatile arterial blood. It does this by measuring light absorption during a pulse and subtracting from that the light absorption occurring between the pulses. If the pulse oximeter cannot detect a pulse, it will not make a measurement. One of the common reasons for poor instrument performance is peripheral vasoconstriction.
Oxygen therapy
Oxygen therapy may be beneficial when the predominant cause of the hypoxemia is ventilation-perfusion mismatching or diffusion impairment. Oxygen therapy may not be substantially beneficial if the predominant cause of the hypoxemia is small airway and alveolar collapse. High inspired oxygen concentrations can be attained with a facemask. If the animal does not tolerate the facemask, the oxygen outlet should be held as close to the animal’s nose as possible. Oxygen cages and hoods are commercially available or can be homemade; oxygen tents and infant incubators are available from used medical equipment suppliers. Enclosed oxygen environments should not be used during the initial stabilization stages, but are useful afterward when the patient needs to be maintained on oxygen. In any enclosed environment, it is important to measure and control the oxygen concentration, to eliminate the carbon dioxide produced by the animal, and to control the temperature. High humidity is acceptable as long as the temperature is controlled at a comfortable level.
CARDIOVASCULAR
Heart rate and rhythm
Sinus tachycardia is primarily a sign of an underlying problem (e.g., hyperthermia, hypoxemia, pain, parasympatholytics such as atropine, sympathomimetics, supraventricular or ventricular ectopic pacemaker activity). In people, because of coronary artery narrowing, sinus tachycardia can increase myocardial oxygen consumption beyond oxygen delivery capabilities. In animals, where coronary artery disease is rare, tachycardia only becomes a problem for the patient when there is not enough time for diastolic filling, which results in a decrease in cardiac output. Specific treatment of sinus tachycardia may be indicated when the heart rate exceeds the low 200s for dogs or the high 200s for cats.
Ventricular arrhythmias may be caused by sympathomimetics, hypoxia, hypercapnia, myocarditis, electrolyte disturbances (potassium and magnesium), and arrhythmogenic factors released from various debilitated abdominal organs, intracranial disease, or digitalis intoxication. Ventricular arrhythmias become a problem for the patient when they interfere with cardiac output, arterial blood pressure, and tissue perfusion, or when they threaten to convert to ventricular fibrillation when (1) the minute-rate equivalent exceeds the high 100s for dogs and the low 200s for cats; (2) the complexes are multiform; or (3) the ectopic beat overrides the T wave of the preceding depolarization. Total elimination of ventricular arrhythmia is not necessarily the goal of therapy since large dosages of antiarrhythmic drugs (Table 7-3) have deleterious cardiovascular and neurological effects. A simple decrease in the rate or severity of the arrhythmia may be a suitable endpoint to the titration of the antiarrhythmic drugs.
Vasomotor tone and tissue perfusion
Peripheral and visceral perfusion is primarily regulated by vasomotor tone. Vasodilation improves peripheral perfusion while vasoconstriction impairs it. Vasodilation is a potent cause of hypotension while vasoconstriction increases blood pressure. Vasomotor tone is assessed by mucous membrane color (pale = vasoconstriction; red = vasodilation), capillary refill time (<1 second = vasoconstriction; >2 seconds = vasodilation), toe-web/core temperature gradient (>4°C = vasoconstriction; <2°C = vasodilation). Vasoconstriction may be caused by hypovolemia, heart failure, hypothermia, or vasoconstrictor sympathomimetics. Vasodilation may be caused by the systemic inflammatory response, hyperthermia, or the administration of vasodilator drugs. Vaso-corrective therapy may be necessary if treatment directed at the underlying cause has failed (Table 7-4).
Pulse quality
Assessment of pulse quality by digital palpation involves an evaluation of both the height and width of the pulse pressure waveform compared with normal. Tall, wide pulse pressure waveforms are seen in sepsis; tall, narrow waveforms occur with a patent ductus and during CPR. Small, narrow pulse pressure waveforms are seen with small stroke volumes and vasoconstriction. Small stroke volumes occur with hypovolemia, poor heart function, tachycardia, and ventricular arrhythmias. The pulse pressure waveform is largely a reflection of stroke volume and vessel size. It is not a measure of arterial blood pressure per se, although in a general way vessels with low pressure are easier to collapse and vice versa. The weak, thready pulse that occurs with hypovolemia is caused by small stroke volumes and vessel constriction; such patients may be normotensive. Peripheral pulse quality (such as the dorsal metatarsal in the dog) decreases and disappears earlier than do larger, more central arteries (such as the femoral) with progressive hypovolemia. The relative pulse quality of more peripheral versus more central arteries may provide a rough index to the magnitude of the hypovolemia.
Central venous pressure
Central venous pressure (CVP) is the luminal pressure of the intrathoracic vena cava. Peripheral venous pressure (PVP) is slightly higher than CVP and may provide some useful information but is subject to unpredictable extraneous influences. CVP (or PVP) is the relationship between venous blood volume and venous blood volume capacity. Venous blood volume is determined by venous return and cardiac output. For CVP, verification of a well-placed, unobstructed catheter can be ascertained by observing small fluctuations in the fluid meniscus within the manometer, synchronous with the heartbeat, and larger excursions synchronous with ventilation. Large fluctuations synchronous with each heartbeat may indicate that the end of the catheter is positioned within the right ventricle.
Arterial blood pressure
Arterial blood pressure can be measured indirectly by sphygmomanometry or directly via an arterial catheter attached to a transducer system. Sphygmomanometry involves the application of an occlusion cuff over an artery in a cylindrical appendage. The width of the occlusion cuff should be about 40% of the circumference of the leg to which it is applied. The occlusion cuff should be placed snugly around the leg. If it is applied too tightly, the pressure measurements will be erroneously low since the cuff itself, acting as a tourniquet, will partially occlude the underlying artery. If the cuff is too loose, the pressure measurements will be erroneously high since excessive cuff pressure will be required to occlude the underlying artery. Inflation of the cuff applies pressure to the underlying tissue and will totally occlude blood flow when the cuff pressure exceeds systolic blood pressure. As the cuff pressure is gradually decreased, blood will begin to flow intermittently. When the cuff pressure falls below systolic pressure: (1) systolic blood pressure can be estimated as the manometer pressure at which needle oscillations begin to occur during cuff deflation (caused by the pulse wave hitting the cuff); (2) systolic blood pressure can be estimated also as the manometer pressure at which one can digitally palpate a pulse distal to the cuff; (3) systolic blood pressure can be estimated as the manometer pressure at which the first blood flow sounds are heard via a Doppler ultrasound crystal placed over an artery distal to the occluding cuff; and (4) oscillometry analyzes the fluctuation of pressure in the cuff as it is slowly deflated and provides a digital display of systolic, diastolic, mean blood pressure, and heart rate. Most of these instruments can be set to recycle at discrete time intervals. Small vessel size and motion can interfere with measurements.
All external techniques are least accurate when vessels are small, when the blood pressure is low, and when the vessels are constricted. Direct measurements of arterial blood pressure are more accurate and continuous compared with indirect methods, but require the introduction of a catheter into an artery by percutaneous or cut-down procedure. The dorsal metatarsal, femoral, and ear arteries are commonly used in dogs and cats. The subcutaneous tissues around dorsal metatarsal and ear arteries are tight and hematoma formation at the time of catheter removal is rarely a problem. Once the catheter is placed, it is connected to a monitoring device. The catheter must be flushed with heparinized saline at frequent intervals (hourly) or continuously to prevent blood clot occlusion. The measuring device could be a long fluid administration set suspended from the ceiling. Fluid is instilled into the tubing via a three-way stopcock to a very high level and then allowed to gravitate into the artery until the hydrostatic pressure of the column of water is equalized with the mean arterial blood pressure of the patient. Since blood pressure oscillates, the system should be closed between measurements to prevent blood from entering into, and clotting, the end of the catheter. The measuring device could also be an aneroid manometer (Figure 7-2). Water or blood must not be allowed to enter the manometer. Sterile saline is injected into the tubing toward the manometer via a three-way stopcock until the compressed air increases the registered pressure to a level above that of mean blood pressure. The pressurized manometer system is then allowed to equilibrate with the mean blood pressure of the patient. The arterial catheter can also be attached to a commercial transducer and recording system. The extension tubing between the catheter and the transducer should not be excessively long and should be constructed of nonexpansible plastic to prevent damped signals. The transducer should be “zeroed” periodically and calibrated with a mercury manometer to verify accurate blood pressure measurements. The stopcock that is opened to room air for the zeroing process must be at the level of the heart. With modern patient monitors, the transducer can be placed anywhere with reference to the patient (the monitor will compensate internally with an “off-set pressure” for any vertical differences between the patient and the transducer). If the relative vertical position between the patient and the transducer changes, the transducer must be rezeroed.
Normal systolic, diastolic, and mean blood pressure are approximately 100 to 140, 60 to 100, and 80 to 120 mm Hg, respectively. In general, one should be concerned when the systolic blood pressure (ABPs) falls below 100 mm Hg or when the mean blood pressure (ABPm) falls below 80 mm Hg. In general, one should be very concerned when the ABPs falls below 80 mm Hg or the ABPm falls below 60 mm Hg. Hypotension may be caused by hypovolemia, poor cardiac output, or vasodilation (Box 7-1). Hypertension (high ABPm), when it occurs, is generally attributed to vasoconstriction. High ABPs, not associated with a high ABPm, is generally attributed to an inappropriate frequency response of the measuring system (for that patient and that time). Hypertension can cause increased hemorrhaging, retinal detachment, increased intracranial pressure, and high afterload to the heart and should be treated when ABPm exceeds 140 mm Hg (see Table 7-4). High ABPm may be due to a light level of anesthesia, hyperthermia, sympathomimetic drugs, hyperthyroidism (thyroxine-catecholamine synergy), renal failure (renin-angiotensin), pheochromocytoma (epinephrine), and an increased intracranial pressure. In the last case, the hypertension is most likely due to Cushing’s response to maintain an adequate cerebral perfusion pressure and should not be treated.
Cardiac output
Cardiac output can be measured by a variety of techniques in clinical patients, but in veterinary medicine it is usually not measured. The concept of cardiac output must be on the forefront of one’s monitoring and therapeutic considerations; it is after all the whole point of adequate cardiovascular function. Poor cardiac output is implied when preload parameters (CVP or PVP, pulmonary artery occlusion pressure, jugular vein distention, postcava distention on chest radiograph, and large end-diastolic diameter on cardiac ultrasound image) are high and the forward flow parameters (pulse quality, arterial blood pressure, signs of vasoconstriction, urine output, and physical and laboratory measures of tissue perfusion) are abnormal. Cardiac output is a flow parameter and can be low even when arterial blood pressure is normal.
Cardiac output may be reduced by poor venous return and end-diastolic ventricular filling (e.g., hypovolemia, positive pressure ventilation, or inflow occlusion); by ventricular restrictive disease (e.g., hypertrophic or restrictive cardiomyopathy, pericardial tamponade, or pericardial fibrosis); by decreased contractility; by excessive bradycardia, tachycardia, or arrhythmias; by regurgitant atrioventricular valves; or by outflow tract obstruction. Poor cardiac output should be improved by correcting the underlying problem when possible. Preload should be optimized. When poor contractility is thought to be the problem, sympathomimetic therapy (see Table 7-3) may be indicated.
Oxygen delivery
Oxygen delivery (DO2) is the product of cardiac output and blood oxygen content (Figure 7-3). Oxygen content is determined by hemoglobin concentration (most important) and PO2. Oxygen delivery must be adequate to meet metabolic requirements (oxygen consumption). Excessive anemia, hypoxemia, bradycardia, arrhythmias, reduced stroke volume, poor heart contractility, valvular lesions, tamponade, and hypovolemia may cause inadequate oxygen delivery. Combinations of these abnormalities can compound the oxygen delivery deficit. Excessive oxygen consumption can occur with hyperthermia and intense muscular activity, such as seizures. A disparity between oxygen delivery and oxygen requirement results in an increased oxygen extraction, low venous oxygen, metabolic (lactic) acidosis, and an increased arterial-mixed venous PCO2 gradient. Treatment of an oxygen delivery deficit should be directed at the underlying cause(s). If hypovolemia is thought to be the primary problem, fluids should be administered to reestablish an effective circulating volume. If contractility is thought to be the primary problem, dobutamine should be administered (dopamine if the patient is also hypotensive).
Fluid therapy
The clinical signs of hypovolemia and extracellular dehydration are different. Hypovolemia is identified by low preload (e.g., low CVP, PVP, or pulmonary artery occlusion pressure; collapsed jugular veins; radiographic appearance of a small postcava; and cardiac ultrasound appearance of a small end-diastolic diameter) and low forward flow (e.g., poor pulse quality, hypotension, vasoconstriction, oliguria in a patient that does not have renal disease, increased oxygen extraction, low venous oxygen, metabolic [lactic] acidosis, and an increased arterial-mixed venous PCO2 gradient). Dehydration is identified by an acute loss of body weight, a decrease in skin elasticity, dry mucous membranes in a patient that is not open-mouth breathing and has not received an anticholinergic, and high urine specific gravity and low sodium concentration in a patient that does not have renal disease and has not recently received a bolus of colloids or a diuretic. Hemoconcentration may occur, depending on the nature of the fluid loss. By definition, dehydrated patients have lost extracellular fluid and therefore all dehydrated patients are to some extent hypovolemic. However, the magnitude of the dehydration and that of the hypovolemia do not necessarily correlate and so each should be evaluated independently. Animals with evidence of subcutaneous edema may be associated with hypervolemia (e.g., heart failure, renal failure, and iatrogenic fluid overload) or hypovolemia (e.g., hypoproteinemia/hypocolloidemia and increased vascular permeability). In general, hypovolemia requires an initial, rapid (over a period of 10 to 60 minutes) fluid therapy plan, whereas dehydration requires a slow (over a period of 4 to 24 hours) fluid plan.
Usually an iso-osmolar, polyionic extracellular fluid (ECF) replacement crystalloid, such as lactated Ringer’s or an equivalent solution with approximately normal extracellular concentrations of sodium, potassium, chloride, and a bicarbonate-like anion (e.g., bicarbonate, lactate, gluconate, or acetate) should be used to restore a deficit. These replacement fluids should be administered without alteration if they are to be administered rapidly or if it is known or suspected that there are no major electrolyte abnormalities.
The volume of fluids required to replace the normal ongoing losses can be determined from predictive charts for the dog (Table 7-5) and cat (Table 7-6). If a chart is not available, the maintenance volume can be assumed to be between 50 mL/kg per day for large dogs, 75 mL/kg per day for small dogs and cats, and 100 mL/kg for very small or young animals.
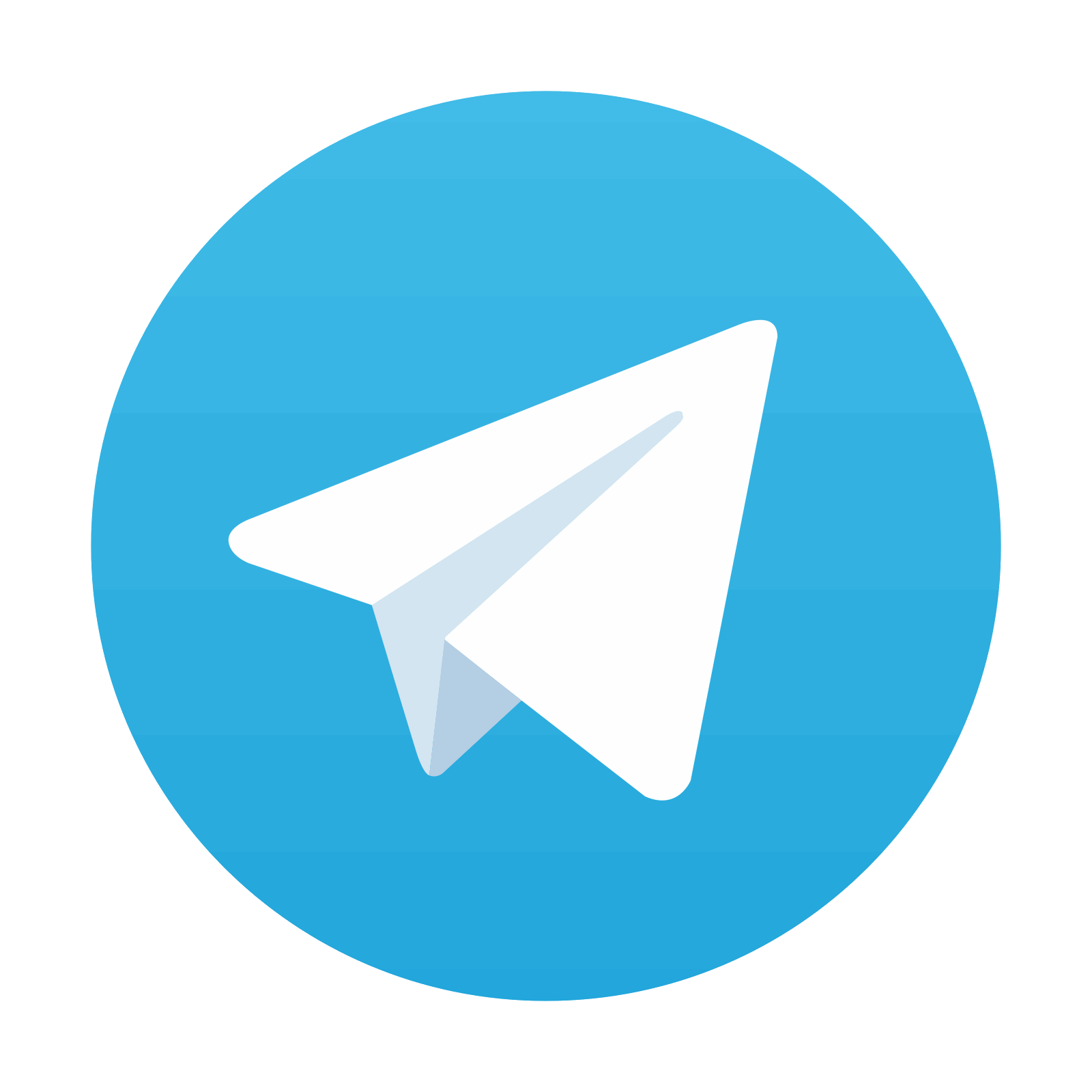
Stay updated, free articles. Join our Telegram channel

Full access? Get Clinical Tree
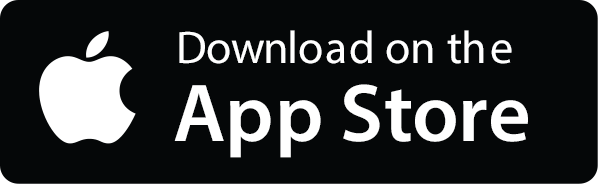
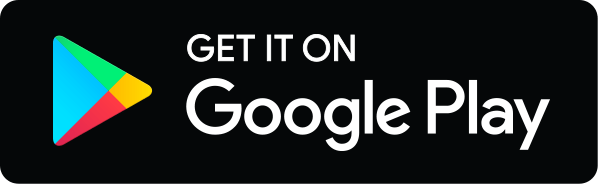