CHAPTER 1 Structure and Function of the Skin
General functions and properties of the skin
The general functions of animal skin are as follows:73,92
Ontogeny
To the authors’ knowledge, the ontogeny of equine skin has not been reported. The following discussion is an amalgamation of information from other domestic mammals and humans.34,36,89,91
Gross anatomy and physiology
In general, skin thickness decreases dorsally to ventrally on the trunk and proximally to distally on the limbs.* The skin is thickest on the forehead, dorsal neck, dorsal thorax, rump, and base of the tail. It is thinnest on the pinnae and on the axillary, inguinal, and perianal areas. The reported average thickness of the general body skin is 3.8 mm, with a range of 1.7-7.7 mm,93,99,107,114 and is thickest over the lumbosacral and gluteal areas. The average skin thickness in the areas of the mane and tail is 6.2 mm (range 3.5-10.7 mm) and 5.3 mm (range 2.5-6.4 mm), respectively.93,107 The hair coat is usually thickest over the dorsolateral aspects of the body and thinnest ventrally, on the lateral surface of the pinnae, and on the undersurface of the tail.
The skin surfaces of haired mammals are, in general, acidic. The pH of normal equine skin has been reported to range from about 4.8 to 6.8 and to increase to as much as 7.9 with sweating.25,48,51Normal equine skin is usually approximately neutral (pH of 7 to 7.4).48
Skin temperature of horses is measured with an electronic thermometer.117 The head and trunk are the warmest areas. Temperature decreases proximally to distally on the limbs. Temperature is lower over bony prominences.
Hair
Hair, which is characteristic of mammals, is important in thermal insulation; sensory perception; and as a barrier against chemical, physical, actinic, and microbial injury to the skin.36,64,91,92,101 The ability of a hair coat to regulate body temperature correlates closely with its length, thickness, and density per unit area and with the medullation of individual hair fibers. In general, hair coats composed of long, fine, poorly medullated fibers, with the coat depth increased by piloerection, are the most efficient for thermal insulation at low environmental temperatures. Coat color is also important in thermal regulation; light-colored coats are more efficient in hot, sunny weather. The glossiness of the coat is important in reflecting sunlight. Transglutaminase is a marker of early anagen hair follicles, and it is important in the protein cross-linking that contributes to the shape and remarkable strength of hair.36 The diameter of the hair shaft is largely determined by the volume of the hair matrix epithelium, and the final length of the hair shaft is determined by both the rate of hair growth and the duration of anagen.
Both primary (outercoat, guard) and secondary (undercoat) hairs are medullated. It has been reported that general body skin has from 2000 to 3000 primary hairs and from 3000 to 5000 secondary hairs, respectively, per cm2 of skin.99 Hair follicles are straight in horses with straight or curly hair coats.93
In general, no new hair follicles are formed after birth. The skin surface is smooth or contains grooves and ridges, and hairs emerge from the grooves.93,107 Hair follicles and thus emerging hairs are oriented both obliquely and perpendicularly to the skin surface.32,93,96,107 In general, the thinner the skin, the more acute the angle of the hair. The direction of the slope of the hairs, which varies from one region of the body to another, gives rise to the hair tracts.92 The study of hair tract patterns is called trichoglyphics. The true significance and origin of hair tracts are unknown. With the hair slope generally running caudally and ventrally, benefits include minimal impediment to forward motion and the ability of water to flow off the body to the ground without soaking the hair coat, which would reduce its thermal-insulating properties. No ready explanation is apparent for either the hair coat pattern or the dermal cleavage lines in horses.81
Hair Cycle
Analysis of the factors controlling or influencing hair growth is complicated by evolutionary history.36,90 The pelage changes as a mammal grows, and that of the adult often differs markedly from that of the juvenile, reflecting different requirements for heat regulation, camouflage, and sexual and social communication. Exmoor and other mountain and moorland ponies that live outdoors in the United Kingdom are considered to have five characteristic hair coats during their lifetime: birth, foal, yearling, adult summer, and adult winter.102 In addition, the cyclic activity of the hair follicles and the periodic molting of hairs have provided a mechanism by which the pelage can be adapted to seasonal changes in ambient temperature or environmental background. This mechanism is influenced by changes in the photoperiod, which acts through the hypothalamus, hypophysis, and pineal gland, altering levels of various hormones (including melatonin, prolactin, and those of gonadal, thyroidal, and adrenocortical origin) and modifying the inherent rhythms of the hair follicle anlagen and their concurrent downward growth and invasion through the dermis.92,104 Signals controlling hair follicle induction, development, regression, and reactivation have not been identified; however, multiple growth factors or their receptors (e.g., epidermal growth factor [EGF], transforming growth factors [TGF-B1, TFG-B2], neurotrophin-3) have been localized to hair follicles and the surrounding mesenchyme. These control cellular proliferation and collagenase release from cultured hair follicles. In addition, an interplay among class I major histocompatibility complex (MHC) expression, chondroitin proteoglycans, and activated macrophages is involved in the regulation of hair growth, especially during the catagen phase. Retinoic acid is important in hair shaft-follicular sheath interactions and processing.125
Hairs do not grow continuously but rather in cycles (Fig. 1-1). Each cycle consists of a growing period (anagen), during which the follicle actively produces hair, and a resting period (telogen), during which the hair is retained in the follicle as a dead (or club) hair that is subsequently lost. There is also a transitional period (catagen) between these two stages. The relative duration of the phases of the cycle varies with the age of the individual, the region of the body, the breed, and the sex, and it can be modified by a variety of physiologic or pathologic factors.
The hair cycle, and thus the hair coat, are controlled by photoperiod, ambient temperature, nutrition, hormones, general state of health, genetics, and poorly understood intrinsic factors.* Intrinsic factors include growth factors and cytokines produced by the follicle, the dermal papilla, and other cells (lymphocytes, macrophages, fibroblasts, mast cells) in the immediate environment. Hair replacement in horses is asynchronous (mosaic) in pattern because neighboring hair follicles are in different stages of the hair cycle at any one time. Replacement is unaffected by castration, and it responds predominantly to photoperiod and, to a lesser extent, to ambient temperature.6,28,29,50 An exception to this is the coarse permanent hairs of the mane, tail, and fetlock (“horsehairs”).52,100,107 Mane hairs grow a mean of 0.059 cm/day.123 Horses in temperate latitudes such as the northern United States, Canada, and the United Kingdom may shed noticeably in the spring.90,92
Exmoor and other mountain and moorland ponies living outdoors in the United Kingdom, and the Przewalski horse in Czechoslovakia, shed once a year between March and May.68,102 A cold, wet season may delay the shed. The spring shed takes as long as 7 weeks, but the date of beginning the shed varies from individual to individual, and in the same individual from year to year. In general, the shed progresses from the limbs and ventral neck and finishes on the back. The short, fine summer coat lasts from June to August. Hair growth increases in autumn, resulting in an increased length and thickness of the hair coat. The long, thick winter coat lasts from September to May. Horses kept blanketed or in heated barns fail to develop a dense winter coat. It has been suggested that domestication and breeding schedules may be causing a shift from periodic to continuous shedding.90
Hair grows until it attains its preordained length, which varies according to body region and is genetically determined; it then enters the resting phase, which may last for a considerable amount of time. Each region of the body has its own ultimate length of hair beyond which no further growth occurs. This phenomenon is responsible for the distinctive coat lengths of various breeds and is genetically determined. Equine mane hairs are similar to human scalp hairs in that they grow to a greater length than the rest of the body hairs and have a long anagen growth phase.92,100,123
Because hair is predominantly protein, nutrition has a profound effect on its quantity and quality (see Chapter 10). Poor nutrition may produce a dull, dry, brittle, or thin hair coat with or without pigmentary disturbances and may result in the retention of the winter hair coat.
Under conditions of ill health or generalized disease, anagen may be considerably shortened; accordingly, a large percentage of body hairs may be in telogen at one time. Because telogen hairs tend to be more easily lost, the animal may shed excessively. Disease states may also lead to faulty formation of hair cuticle, which results in a dull, lusterless coat. Severe illness or systemic stress may cause many hair follicles to enter synchronously and precipitously into telogen. Shedding of these hairs (telogen defluxion; see Chapter 10) thus occurs simultaneously, often resulting in visible thinning of the coat or actual alopecia.
The hair cycle and hair coat are also affected by hormonal changes.36,64 In general, anagen is initiated and advanced, and hair growth is accelerated, by thyroid hormones and growth hormones. Dermal papilla cells, which are a mesenchymal component of the hair bulb, are considered to play a fundamental role in the induction of epithelial differentiation. These cells are morphologically and functionally differentiated from dermal fibroblasts and are thought to be the primary target cells that respond to hormones and mediate growth-stimulating signals to the follicular epithelial cells.
Attention has been focused on the usefulness of hair analysis as a diagnostic tool.15 It is well recognized by most dermatologists and nutritionists in human medicine that mineral and trace element analysis of hair samples is not a clinically useful tool in the assessment of nutritional status.92 For instance, coat color, age of animal, diet, corticosteroid therapy, and even month of the year have been shown to affect the mineral concentration of equine hair.15 Until and unless adequate scientific documentation of the validity of such multielement analysis is performed, it is necessary for health professionals and the public to be aware of the limited value of hair analysis and of the potential to be confused and misled by it.43
Hair has been analyzed for drug abuse in humans for years, but hair analysis to detect drug administration has not been studied extensively in horses. A dose-time correlation for morphine concentration in equine mane hairs has been reported.123 Hence, hair analysis may be of use in forensic investigations in horses. Enrofloxacin and ciprofloxacin were detected in equine mane and tail hairs for up to 10 months post-treatment.26 In vitro enrofloxacin and ciprofloxacin were extensively bound to melanin, and in vivo their uptake was 40-fold greater in black than white hair.
Hair Coat Color
Coat color genetics in horses has been studied in some detail.92,112 Gene W causes an inability to form pigment in skin and hair. Horses that possess the dominant allele W are typically “white” from birth (skin pink, eyes blue or brown, hair white). Such horses are sometimes called “albino.” All nonwhite horses are ww.
White Camarque horses are black or dark gray at birth and lose their coloring during the course of their lives.2,35 This process begins at the age of 2- to 4-years-old, and the horses have a characteristic silver-gray coat by about the age of 10-years-old. In one study, the degree of coat pigmentation in white Camarque horses correlated directly with α-melanocyte-stimulating hormone (MSH) plasma levels.2
A number of cutaneous patterns or lines are evoked to explain certain distributions of skin lesions encountered clinically.92 Voight lines are the boundaries of the areas of distribution of the main cutaneous nerve stems. Langer lines reflect the course of blood vessels or lymphatics. Blaschko lines form the pattern assumed by many different nevoid and acquired skin diseases. Blaschko lines reflect a mosaic condition deriving either from a single mutated clone of cells originating from a postzygotic mutation or from an x-linked mutation made evident by lyonization. These lines follow a V-shape over the spine; an S-shape on the abdomen; an axial distribution on the limbs; and a wavy pattern down the forehead, over and below the eyes, over the upper lip, and behind the ear. Tension lines are determined by muscle action, connective tissue fiber orientation and traction, and gravity.
Microscopic anatomy and physiology
The microscopic anatomy and physiology of the skin of horses have been the subjects of several studies (Fig. 1-2).*
Epidermis
The outer layer of the skin, or epidermis, is composed of multiple layers of cells defined by position, shape, polarity, morphology, and state of differentiation of the keratinocytes (Figs. 1-2–1-5). These are of four distinct types: keratinocytes (about 85% of the epidermal cells), melanocytes (about 5%), Langerhans cells (3-8%), and Merkel cells (about 2%), which are associated with tylotrich pads. For purposes of identification, certain areas of the epidermis are classified as layers and are named, from inner to outer, as follows: basal layer (stratum basale), spinous layer (stratum spinosum), granular layer (stratum granulosum), clear layer (stratum lucidum), and horny layer (stratum corneum). In general, the epidermis of the horse is five to seven nucleated cell layers thick (not counting the horny layer) in haired general body skin (see Fig. 1-3), ranging from 30 to 100 μm in thickness (average 53 μm).93,96,107 The epidermis is thicker in mane and tail regions (40-200 μm, average 91 μm) and near mucocutaneous junctions (40-1000 μm, average 238 μm) (see Fig. 1-4).93,107 Rete ridges (projections of the epidermis into the underlying dermis) are not found in the normal hair-bearing skin of horses, except for the mane and tail base regions.93,107 Rete ridges, however, may be found in relatively glabrous areas (muzzle, mucocutaneous junctions) and the coronary band.
Basal layer
The stratum basale is a single row of columnar or cuboidal cells resting on the basement membrane zone that separates the epidermis from the dermis (see Fig. 1-5). Most of these cells are keratinocytes that are constantly reproducing and pushing upward to replenish the epidermal cells above. The daughter cells move into the outer layers of the epidermis and are ultimately shed as dead horny cells. Apoptotic keratinocytes (two apoptotic basal keratinocytes in 54 6-mm sections) and mitotic figures (three mitotic basal keratinocytes in 54 6-mm sections) are rarely seen.93 There is morphologic and functional heterogenicity in basal keratinocytes;27,36 some populations serve primarily to anchor the epidermis, and others serve a proliferative and reparative (stem cell) function. The tips of the deep epidermal rete ridges (in glabrous skin) and the bulb region of the hair follicle (site of attachment of the arrector pili muscle) are the presumed sites of the epidermal and hair follicle stem cells.34,92 The basal cell layer not only serves as the progenitor cell layer, but also produces the basement membrane, which functions as the site of attachment of the epidermis to the dermis.
Hemidesmosomes are junctional complexes distributed along the inner aspect of basal keratinocytes, whose major role is epidermal-dermal adhesion.34,36,79 The linkage of the keratin intermediate filament (cytokeratin) network to the hemidesmosome and basal keratinocyte plasma membrane involves several components, including the plaque proteins bullous pemphigoid antigen I (BPAG I or BP 230) and plectin, the transmembrane proteins α6β4 integrin and BPAG II (BPAG 180 or collagen XVII), and laminin 5.34,58,105 Various inherited or acquired defects in the hemidesmosome-anchoring filament components are known to produce various forms of epidermolysis bullosa and pemphigoid.34
Integrins are a large family of cell surface adhesive receptors.34,58,105 These cell surface glycoproteins are important in cell-cell and cell-matrix interactions and also act as signal transducers through which extracellular and intracellular components can influence and modify each other. Each integrin consists of a heterodimer of an α and a β subunit, which are noncovalently associated. In the epidermis, integrin expression is normally confined to the basal layer. The integrin subunits that are most abundant in the epidermis are α2, α3, β1, α6, and β4. Examples of keratinocyte integrin functions include α5β1, which mediates keratinocyte adhesion to fibronectin; α2β1, which mediates keratinocyte adhesion to collagens Type I and IV and laminin; α3β1, which is a receptor for epiligrin and is involved in adhesion to laminin; α1β5, which mediates keratinocyte adhesion to vitronectin; and α6β4, which mediates keratinocyte adhesion to laminin (Table 1-1).58
Melanocytes and melanogenesis
Melanocytes, the second type of cell found in the basal layer of the epidermis (see Figs. 1-3–1-6), are also found in the outer root sheath and hair matrix of hair follicles, in the ducts of sebaceous and sweat glands, and perivascularly in the superficial dermis.* Traditionally, melanocytes are divided structurally and functionally into two compartments: epidermal and follicular. Because melanocytes do not stain readily with hematoxylin and eosin (H&E) and because they undergo artifactual cytoplasmic shrinkage during tissue processing, they appear as clear cells or melanized dendritic cells in the stratum basale. In general, there is one melanocyte per 2 to 20 keratinocytes in the basal layer.93 They are derived from the neural crest and migrate into the epidermis, adnexal epithelia, and superficial dermis in early fetal life. Although melanocytes are of nondescript appearance, with special stains (DOPA reaction, Fontana ammoniacal silver nitrate) they can be seen to have long cytoplasmic extensions (dendrites) that weave among the keratinocytes. There is an intimate relationship between melanocytes and keratinocytes in which both cells interact and exist as epidermal symbionts—a functional and structural unit called the epidermal melanin unit. The epidermal melanin unit is dynamic and highly responsive to endogenous and exogenous stimuli. There is a complex communication among the melanocyte, its corresponding keratinocytes, and the surrounding epidermal environment that determines the constitutive level of melanocyte function. Ultrastructurally, melanocytes are characterized by typical intracytoplasmic melanosomes and premelanosomes and a cell membrane-associated basal lamina (Fig. 1-7). Most of the melanin pigment in the skin is located in the basal layer of the epidermis, but in dark-skinned animals, melanin may be found throughout the entire epidermis and within superficial dermal melanocytes. Melanin granules are often clustered as “caps” dorsal to keratinocyte nuclei, presumably a photoprotective localization.
Although the melanocyte accounts for only a small proportion of the epidermal cells, it serves a variety of important roles: (1) a cosmetic entity, participating in protective coloration and in sexual attraction; (2) a barrier against ionizing radiation, especially important in protection against ultraviolet light (UVL); (3) a scavenger of cytotoxic radicals and intermediates; and (4) a participant in developmental and inflammatory processes. Although melanin absorbs UVL over a broad spectrum, including UVA and UVB, it is not a particularly efficient absorber of UVL. It probably photoprotects in other ways, possibly as a quencher of free radicals generated in response to UVL. In dark-skinned horses, melanin granules are present throughout all layers of the epidermis, decreasing in quantity from the stratum basale to the stratum corneum.93
Melanins embrace a wide range of pigments, including the brown-black eumelanins, yellow or red-brown pheomelanins, and other pigments whose physicochemical natures are intermediate between the two. Pheomelanins differ from eumelanins by containing a high proportion of sulfur. Despite the different properties of the various melanins, they all arise from a common metabolic pathway in which dopaquinone is the key intermediate.34,36,59
Melanogenesis takes place exclusively within melanocytes and on the specialized organelle, the melanosome.34,36,59,79 Here, the specific enzyme tyrosinase catalyzes the conversion of tyrosine to dopa. Tyrosinase is the rate-limiting enzyme in the melanin pathway. It is a copper-containing enzyme, is found exclusively in melanocytes, and is thus a good specific marker for these cells. Tyrosinase is an unusual enzyme in that it has three distinct catalytic activities. The most critical is its tyrosinase hydroxylase activity, converting tyrosine to dopa. However, it is also able to use dopa or 5,6-dihydroxyindole (DHI) as substrates for oxidase activities. Mutations in the tyrosine structural gene are responsible for several types of albinism.34
Once dopa forms, it can spontaneously autooxidize to dopaquinone without tyrosinase (though at slower rates) and continue through the melanin pathway to dopachrome, 5,6-dihydroxyindole-2-carboxylic acid (DHICA), DHI, and indole-5,6-quinone.36,59,79 Another melanocyte-specific enzyme is dopachrome tautomerase, which converts dopachrome to DHICA. This conversion requires the presence of iron.
The determination to produce eumelanins or pheomelanins is under genetic control.36,59,79 If sulfhydryl groups are available, pheomelanins are produced. It has been proposed that the “switching” of melanin synthesis is mainly controlled by the levels of tyrosinase, with high levels producing eumelanins and low levels producing pheomelanins.
Mammalian pigmentation is regulated at many different developmental, cellular, and subcellular levels, and is influenced by many genes.36,59,79 Although melanocytes in the skin have characteristic basal levels of function that are particular to each individual, they are highly responsive cells that continually sample their environment and modulate their levels of proliferation and melanogenesis. Classically, melanin production was thought to be under the control of genetics and MSH from the pituitary gland.36,113 The main pigmenting hormones from the pituitary gland include α-MSH (α-melanocortin), adrenal cortical stimulating hormone (corticotropin), and β-lipotropic hormone (β-lipotropin).59,79 These hormones are derived from a larger precursor molecule, propiomelanocortin. However, the role of these hypophyseal origin hormones in physiologic and pathologic pigmentation in mammals is largely unknown. At present, interest focuses on the theory that melanogenesis and melanocyte proliferation and differentiation are most often regulated locally in paracrine and autocrine fashion.
Melanocytes express a number of cell surface receptors (e.g., intercellular adhesion molecule 1 [ICAM-1]) that allow interaction with other cells in their environment, including keratinocytes, Langerhans cells, fibroblasts, lymphocytes, and macrophages.34,59,79,124 They express receptors for and respond to (modifying their proliferation, differentiation, and melanogenesis) growth factor (e.g., β fibroblast growth factor [FGF]), hormones, interferons (IFNs), interleukins (ILs), eicosanoids, retinoic acid, vitamin D3, and a host of other cytokines. In fact, melanocytes are able to produce some of these themselves, thus acting in an autocrine manner. Melanocytes themselves secrete several cytokines (e.g., IL-8) and participate in inflammatory and immunologic reactions. Many of the precursors and intermediates of the melanin biosynthetic pathway are cytotoxic and could contribute to cellular injury and inflammation. It can be appreciated that a highly complex interaction exists among the cellular components of the epidermis, their respective immune cytokines, and the inflammatory mediators released in response to injury.
α-MSH is a neuroimmunomodulatory and antiinflammatory peptide that is synthesized and released by keratinocytes, Langerhans cells, fibroblasts, endothelial cells, and melanocytes themselves.59,79 α-MSH cell surface receptors can also be identified on these cells. α-MSH can, hence, modulate keratinocyte proliferation and differentiation, and endothelial cell and fibroblast cytokine and collagenase production. It also downregulates the production of proinflammatory cytokines and accessory molecules on antigen-presenting cells (monocytes and macrophages). α-MSH is an antagonist of IL-1, an important cytokine in the cutaneous immune response. Thus α-MSH is part of a mediator network that modulates cutaneous inflammation and hyperproliferative skin disorders. This may be far more important than any effect it has on skin pigmentation.
Melanogenesis takes place in membrane-bound organelles called melanosomes,34,36,59,79 designated Stages I through IV according to maturation (see Fig. 1-7). It is often stated that the ultrastructural hallmark of the melanocyte is the melanosome. However, it is more accurate to say that Stage I melanosomes are melanocyte-specific, because later stage melanosomes may be found in keratinocytes and other phagocytic cells.59 Melanosomes originate from the Golgi apparatus where the tyrosinase enzyme is formed. Stage I melanosomes contain no melanin and are electron-lucent. As melanin is progressively laid down on protein matrices, melanosomes become increasingly electron-dense. At the same time, they migrate to the periphery of the dendrites, where transfer of melanin to adjacent keratinocytes takes place. Transfer involves the endocytosis of the dendrite tips and the incorporated Stage IV melanosomes by the adjacent keratinocytes. Melanocytes eject melanosomes into keratinocytes by a unique biologic transfer process called cytocrinia.36 Dermal melanocytes are often referred to as continent melanocytes, because they do not transfer melanosomes as do the epidermal or secretory melanocytes. Skin color is determined mainly by the number, size, type, and distribution of melanosomes.
At present, there are no histochemical stains that can be performed on routinely processed skin biopsy specimens that exclusively stain melanin.30,59 Argentaffin stains rely on the ability of melanin to reduce silver from a silver solution (e.g., silver nitrate). Examples of argentaffin stains include Fontana-Masson and Gomori methenamine silver. These agents also stain neurosecretory granules and formalin pigment. Argyrophil stains are similar to argentaffin stains but use an external silver reducer to produce elemental silver. An example of an argyrophil stain is Grimelius stain. Argyrophil stains also stain nerves, reticulum, and elastic fibers.
Merkel cells
Merkel cells are dendritic epidermal clear cells confined to the basal cell layer, or just below, and occur in touch corpuscles (tylotrich pads) and the bulge region of the hair follicle (Fig. 1-8).27,36,59,92,124 These specialized cells (slow-adapting mechanoreceptors) contain a large cytoplasmic vacuole that displaces the cell nucleus dorsally, and their long axis is usually parallel to the skin surface. They possess desmosomes and characteristic dense-core cytoplasmic granules and paranuclear whorls on electron microscopic examination (Fig. 1-9). Merkel cells also contain cytokeratin, neurofilaments, and neuron-specific enolase, suggesting a dual epithelial and neural differentiation. Current evidence suggests that Merkel cells derive from a primitive epidermal stem cell.58 Merkel cells may have other functions, such as influencing cutaneous blood flow and sweat production (via the release of vasoactive intestinal peptide), coordinating keratinocyte proliferation, and maintaining and stimulating the stem cell population of the hair follicle (hence controlling the hair cycle).36,124
Spinous layer
The stratum spinosum (prickle cell layer) is composed of the daughter cells of the stratum basale (see Fig. 1-5).30,36,107 In general body haired skin, this layer is three to five cells thick.93 The spinous layer becomes much thicker at mucocutaneous junctions, on the muzzle, and at the coronary band. The cells are lightly basophilic to eosinophilic, nucleated, and polyhedral to flattened cuboidal in shape. The keratinocytes of the stratum spinosum appear to be connected by intercellular bridges (prickles), which are more prominent in nonhaired skin.
Keratinocyte adhesion is mediated by four major types of adhesive and communicative structures: desmosomes, hemidesmosomes, adherens junctions, and focal adhesions (see Table 1-1).30,36,58 Hemidesmosomes and focal adhesions are located on the basal surface of basal cells and mediate adhesion to the underlying extracellular matrix, whereas desmosomes and adherens junctions (containing the classic cadherins, E-cadherin, and P-cadherin) mediate adhesion between keratinocytes in all epidermal layers. Gap junctions are formed by the protruding ends of many identical protein complexes that lie in the plasma membranes of apposed cells. These protein complexes are called connexons, and each connexon is made of six connexins. Gap junctions serve primarily as intercellular routes of chemical communication.
Desmosomes are presently known to consist of keratin intermediate filaments and their attachment plaques, the keratinocyte plasma membrane, and the desmosomal core (desmoglea), which is interposed between two adjacent keratinocyte plasma membranes.30,36,58 Numerous desmosomal plaque proteins (desmoplakins I and II, plakoglobin, plakophilin, keratocalmin) and desmosomal core glycoproteins (which contain the desmosomal cadherins, desmogleins I, II, III and desmocollins I, II, III) have been characterized. Proteins of the plakoglobin (plakoglobin, β-catenin), vinculin (vinculin, α-catenin), and ezrin (talin, radixin) families are found at desmosomal and adherens junction attachments.
The keratinocyte cytoskeleton consists of three types of cytoplasmic filaments: cytokeratin, actin, and microtubules (tubulin).58 These filaments function in the orientation, polarization, organelle sorting, motility, shape change, signal transduction, and structural resilience of keratinocytes. Ultrastructurally, keratinocytes are characterized by tonofilaments and desmosomes (Fig. 1-10).30,36,58
Calcium and calmodulin are crucial for desmosome and hemidesmosome formation. At least three keratinocyte-derived calmodulin-binding proteins participate in a flip-flop regulation (calcium concentration-dependent) of calcium-calmodulin interactions: caldesmon, desmocalmin, and spectrin.34,36 Immunohistochemically, keratinocytes are characterized by the presence of cytokeratins.36,58 All epithelia express a keratin pair: one keratin chain from the acidic subfamily (Type I keratins, cytokeratins 9-20) and one chain from the neutral-basic subfamily (Type II keratins, cytokeratins 1-8).36,39,58,120 The keratin pairs change with different epithelia, and in the same epithelia at various stages of differentiation or proliferation. A number of workers have published electrophoretic patterns of proteins isolated from the keratins of a variety of animals and, on the basis of observed differences in banding patterns, have suggested that the technique might be useful as an aid to taxonomy, animal classification, and identification.36 The keratinocytes of the stratum spinosum synthesize lamellar granules (keratinosomes, membrane-coating granules, Odland bodies), which are important in the barrier function of the epidermis (Fig. 1-11).34,36 Keratinocytes are phagocytic (erythrocytes, melanin, melanosomes, cellular fragments, latex beads, inorganic substances) and play a role in the metabolism of potentially toxic compounds.27,34,92 Culture techniques for equine keratinocytes have been described.126
The epidermis and the hair follicle epithelium have the capability to process and metabolize molecules in a manner similar to the liver.27,34 The skin has a highly inducible cytochrome P-450-dependent microsomal mixed function oxidase system capable of metabolizing and conjugating a variety of compounds. The skin is believed to be a first line of metabolic defense against topical exposure to toxic compounds.
Langerhans cells
Langerhans cells are mononuclear, dendritic, antigen-presenting cells located in the suprabasal epidermis and the dermis.27,34,36,40,124 They are epidermal clear cells that, like Merkel cells, do not stain for melanin with DOPA. Langerhans cells have characteristic intracytoplasmic organelles (Birbeck or Langerhans granules), which are observed by means of electron microscopy (Fig. 1-12).27,36,40,54,124 Birbeck granules are variously described as having an appearance similar to a zipper, rod, flask, or tennis racket. They form by invagination of the plasma membrane and bound antigen, thus providing the morphologic description of the mechanism by which Langerhans cells internalize surface-bound antigen for processing and representation at the surface. Langerhans cells are aureophilic (i.e., they stain with gold chloride), and they contain membrane-associated adenosine triphosphatase, vimentin, CD45 (common leukocyte antigen), and S-100 protein (immunohistochemical markers). They are most specifically identified by monoclonal antibodies to CD1. Langerhans cells have Fc fragment (Fc)-immunoglobulin G (IgG) and complement 3 (C3) receptors, high-affinity receptors for IgE, and they synthesize and express antigens associated with the immune response gene. These cells are of bone marrow origin and of monocyte-macrophage lineage, and serve antigen-processing and alloantigen-stimulating functions. Following UVL exposure, epidermal Langerhans cells are decreased in density and altered morphologically, resulting in an immunosuppressive environment and antigen-specific tolerance.34,36 Topical or systemic glucocorticoids are known to depress Langerhans cell numbers and function.34,36 The number of Langerhans cells per unit of skin varies from one area of skin to another in the same individual, emphasizing the need to use adjacent normal skin as a control when counting Langerhans cells in skin lesions.36
The skin as an immunologic organ
The epidermis functions as the most peripheral outpost of the immune system. Langerhans cells, keratinocytes, epidermotropic T lymphocytes, and draining peripheral lymph nodes are thought to form collectively an integrated system of skin-associated lymphoid tissue or skin immune system that mediates cutaneous immunosurveillance.* Langerhans cells stimulate the proliferation of relevant helper T lymphocytes by the presentation of antigen; they also induce cytotoxic T lymphocytes directed to allogenic and modified self-determinants, produce IL-1 and other cytokines, contain numerous enzymes, and are phagocytic.*
Keratinocytes play an active role in epidermal immunity.7,34,36,79 They (1) produce ILs (IL-1, IL-2, IL-3, IL-8); (2) produce numerous other cytokines (e.g., prostaglandins, leukotrienes, IFN, colony-stimulating factors); (3) are phagocytic; and (4) can express antigens associated with the immune response gene in a variety of lymphocyte-mediated skin diseases (presumably as a result of IFN-γ secretion by activated lymphocytes).†
Lymphocytes were not found in the epidermis and adnexal epithelia of skin-biopsy specimens from normal equine skin.113a
Granular layer
The stratum granulosum ranges from one to two cells thick in general body haired skin and is thicker at mucocutaneous junctions and at the infundibulum of hair follicles.93,107 Cells in this layer are flattened and basophilic, and they contain shrunken nuclei and large, deeply basophilic keratohyalin granules in their cytoplasm (see Fig. 1-5). Keratohyalin granules are not true granules; they lack a membrane and are more accurately described as insoluble aggregates. Keratohyalin granules are the morphologic equivalents of the structural protein profilaggrin, which is the precursor of filaggrin and is synthesized in the stratum granulosum.34,36,55,56
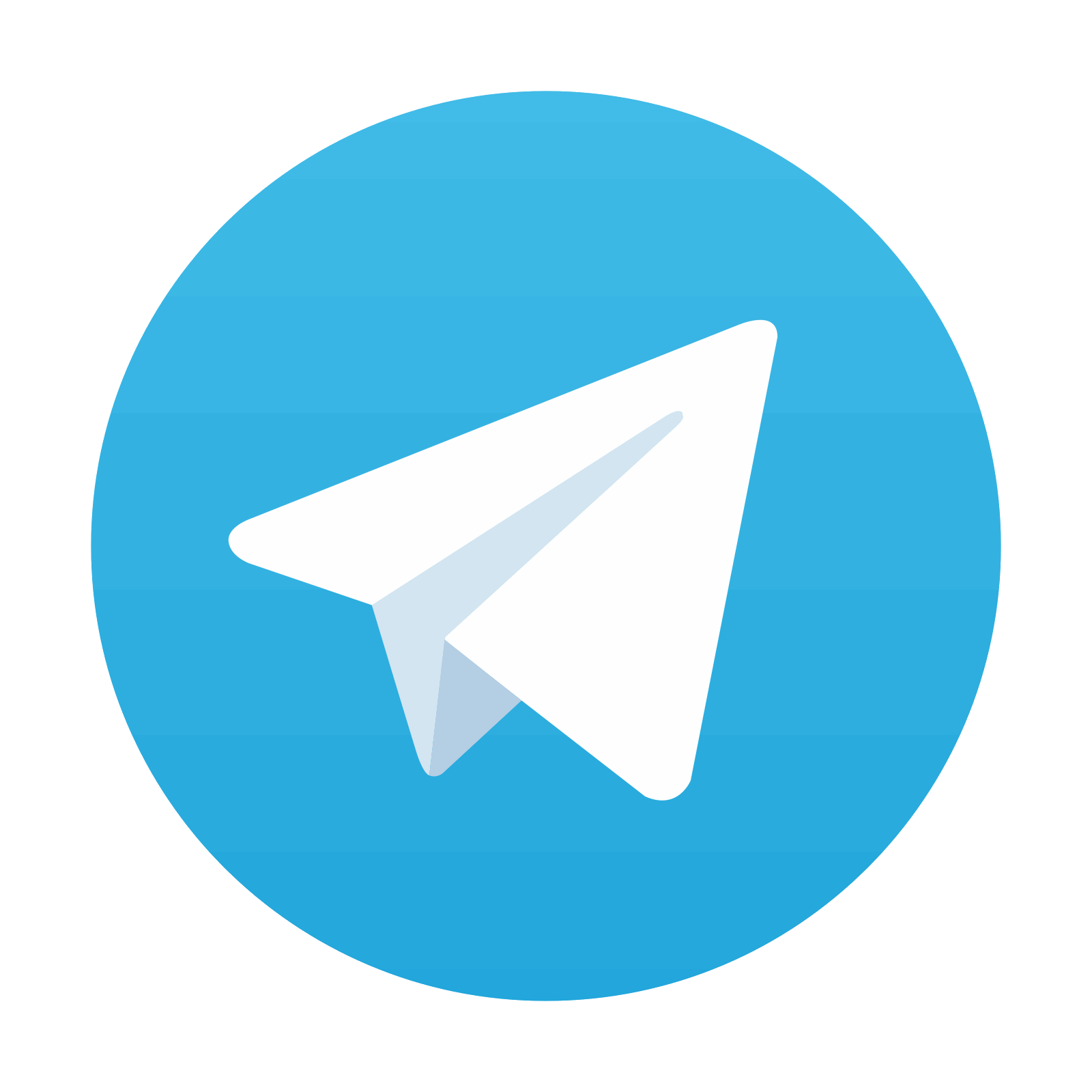
Stay updated, free articles. Join our Telegram channel

Full access? Get Clinical Tree
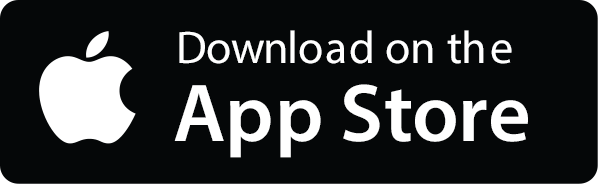
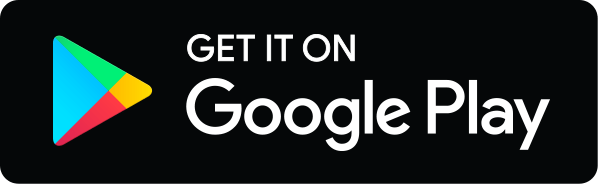