John F. Timoney Streptococcus is an important genus of human and animal primary and opportunist pathogens, and of non‐pathogenic commensals. Primary pathogens are associated with acute extracellular infections of different types, including septicemias and pneumonia, with mastitis, as well as infections of the throat and its local lymph nodes. Streptococci are Gram‐positive spherical bacteria less than 2 μm that typically grow by cell division in one plane, so that nascent cells form a linear array. Most are facultatively anaerobic and catalase negative with complex and variable nutritional requirements, which reflect adaptation as commensals or pathogens. Identification is based on a combination of colony characteristics, hemolytic properties, carbohydrate and protein antigen composition, fermentation and other biochemical reactions, DNA sequence of 16 and 23S rRNA genes, and, within species, multilocus sequence typing (MLST), including that of core genomes (cgMLST). The majority of pathogenic streptococci possess a serologically active carbohydrate antigenically different from one species or group of species to another. These cell‐wall antigens, designated A–H and K–V, are the basis of the Lancefield grouping system and are widely used by clinical laboratories for serogrouping. Groups B, C, D, E, G, L, U, and V contain the pyogenic streptococci responsible for suppurative (pus‐producing) infections in a variety of host species. Some pathogenic streptococci, notably Streptococcus uberis, Streptococcus parauberis, and Streptococcus pneumoniae, are not groupable in the Lancefield scheme. The common animal pathogenic species are summarized in Table 26.1. Streptococci pathogenic for domestic animals can to some extent be grouped by their adaptation to specific organs/body systems. Thus, Streptococcus agalactiae, Streptococcus dysgalactiae, and S. uberis cause disease in the udder; Streptococcus equi, Streptococcus canis (some feline strains), and Streptococcus porcinus are pathogens of the lymphatics of the head and neck; S. pneumoniae causes lower respiratory tract disease in horses; Streptococcus suis is adapted to survive on/in blood mononuclear cells that transport it to the central nervous system (CNS), lungs, and joints. Moreover, all these streptococci exhibit varying degrees of host specificity and so contrast with Streptococcus zooepidemicus, which, although the closely related ancestor of S. equi, is an opportunist pathogen of different body systems in a variety of hosts. Table 26.1 Pathogenic streptococci of animals arranged by Lancefield group and their important virulence and virulence‐associated factors. The known virulence factors of the streptococci most frequently involved in animal disease are shown in Table 26.1. With the exception of S. pneumoniae and S. suis, all are loosely categorized as pyogenic. Comparison of the available genome sequences of the pyogenic streptococci shows that about 66% of their genetic content is common to all; the remainder are variable and formed by genes associated with prophages, integrative conjugative and elements, insertion elements, and other genes acquired by horizontal transfer. In general, streptococcal virulence is based on surface and secreted proteins and on structures that directly or indirectly impede phagocytosis, are involved in adhesion and carbohydrate metabolism, induce release of proinflammatory cytokines or that degrade host proteins. The best understood streptococcal virulence factors are the hyaluronic acid capsule, the antiphagocytic M proteins, and the pyrogenic exotoxins. However, other molecules, including streptolysins, proteases, leukocidal toxins, plasminogen activators (streptokinase), and plasmin receptors found on the surface or secreted, also contribute to pathogenicity. In addition, most pathogenic streptococci have the ability to bind components of the host’s plasma, such as albumin, immunoglobulins (Ig), and fibrinogen, and to bind to fibronectin, laminin, and other components of the host cell. Organisms coated with one or more of these plasma components may be able to evade host defenses either by escaping detection or by blocking deposition of opsonic components of complement. S. agalactiae, the lone member of the Lancefield group B, is an important cause of chronic, subclinical contagious bovine mastitis. It is also a cause of mastitis and invasive disease in camels and an occasional cause of disease in dogs, cats, fish, and hamsters. A serious pathogen of human newborns, it causes neonatal septicemia and meningitis. Human and bovine populations of S. agalactiae are generally distinct and separate, with limited evidence of interspecies transmission (Sukhnanand et al. 2005). Genetic analysis of global isolates has demonstrated epidemic clones adapted to human or bovine hosts (Skov Sorensen et al. 2010). In recent years a hypervirulent clone, sequence type (ST) 283, has been isolated from diseased humans and Tilapia in Southeast Asia, apparently spread through food‐borne infection to humans from these fish, in which infection causes loss (Barkham et al. 2019). The originating source remains unknown. ST67 has been widespread among bovine isolates and ST103 has been identified widely in dairy herds in Scandinavia. These types as well as ST568 dominate among bovine isolates and therefore may have virulence traits specific for the udder (Pang et al. 2017). There are nine serotypes based on a polysaccharide capsule which varies based on the arrangement of four sugars into a unique repeated unit. Horizontal transfer of genes for capsule biosynthesis accounts for diversity in capsule serotype. About 25% of strains are untypable. Conjugative transfer of large segments of chromosomal DNA among strains of S. agalactiae (Brochet et al. 2008) and recombination account for both strain diversity and emergence of clonal complexes with combinations of virulence factors and capsular type suited to particular host niches. S. agalactiae is a parasite of the epithelium and tissues of ruminant mammary glands, and eradication of the organism from herds is therefore advanced by identification of animals with mammary infection followed by treatment or culling. Recent recognition of strains that survive in the environment may undermine this approach (Jorgessen et al. 2016). Bovine isolates show enhanced growth in milk compared to isolates from humans and fish. Virulence and virulence‐associated factors are summarized in Table 26.1. Capsular polysaccharide encoded by cps, including its type‐specific antigen, is antiphagocytic, and specific antibodies are mouse protective. Capsular genes may transfer horizontally and selection for different capsular types may involve fitness responding to the acquired immune response. The terminal sialic acid on type III capsular polysaccharide inhibits activation of the alternate complement pathway and blocks deposition of C3 on the bacterial surface. Capsule also promotes adherence to epithelium. A diverse and large number of proteins, representing at least 10 families and often encoded on pathogenicity islands, are coordinately expressed with the capsule on the surface of S. agalactiae. They function in adhesion, invasion, iron binding, metabolism, transport, and inhibition of phagocytosis (Lindahl et al. 2005). The 105‐kDa BPS protein (group B streptococcal protective surface antigen) is found predominantly with the R1 protein on type 1a isolates and is protectively immunogenic for mice (Erdogan et al. 2002). The 45‐kDa Sip protein is exposed on the polar surfaces of all serovars of S. agalactiae. The conserved Sip protein lacks an anchor sequence motif, and so its attachment to the bacterial surface may depend on an interaction with another bacterial protein. The cyclic adenosine monophosphate (cAMP) factor is a 23.5‐kDa ceramide binding protein that potentiates the action of staphylococcal sphingomyelinase (beta toxin); it is sometimes used in the identification of S. agalactiae. The lethal property of the CAMP factor for cell cultures suggests a cytotoxic action for mammary tissue. It binds to the Fc region of IgM and IgG. Insertional inactivation of efb, the gene that encodes this protein, increases mouse LD50 50 times, demonstrating its importance in virulence. The surface‐expressed fibrinogen‐binding FbsA protein contributes to adhesion, aggregates platelets, and may hinder opsonization by phagocytes. The HylB protein (hyaluronate lyase) may contribute to tissue spread by degradation of N‐acetylglucosamine. Surface pili encoded by dick, Pilbara and PilC may also contribute to adhesion in bovine mastitis. The conserved P1‐2b pilus protein appears to be expressed only by bovine isolates and so is a potential vaccine candidate in the cow. With the exception of FbsA, the presence of some or all of these genes varies greatly among bovine isolates (Arpini et al. 2016). Genetic analysis of S. agalactiae from global sources has identified epidemic strains adapted to either human or bovine hosts. These clones seem to have emerged from a genetically heterogenous core population by recombination involving major parts of the genome (Skov Sorensen et al. 2010). Comparison of arrays of genes for virulence factors and lactose operon has identified four genotypes, human, bovine, fish and environmental (Pang et al. 2017). All the bovine isolates carried the pilus island 2b, the lactose operon, an enhanced ability to grow in milk and form biofilms, and a greater ability to adhere to bovine epithelial cells than the other strains (Pang et al. 2017). S. agalactiae enters through the teat meatus, and colonization of the gland is favored by adhesion to epithelium of gland sinuses. Backjetting of contaminated milk against the teat ends is an important factor in the introduction of infection past the teat sphincter. Keratin and associated bacteriostatic long‐chain fatty acids of the teat canal are the first barriers to physical penetration of the epithelial lining. Bacterial multiplication is controlled by the lactoperoxidase–thiocyanante–H2O2 system, by lysozyme, and by the flushing action of milk during milking. Multiplication on the epithelium of the teat and duct sinuses results in a slowly progressing inflammation and fibrosis. Although S. agalactiae rarely penetrates the epithelium, some cows experience a transient invasion of the lymphatics and supramammary lymph nodes. Release of chemoattractants from damaged host cells and S. agalactiae attracts polymorphonuclear leukocytes (PMNs), which then ingest and kill many of the invading streptococci. Since normal milk has very low complement content, opsonization is probably derived from C3 in the inflammatory exudate, which becomes fixed on the bacterial surface. Initial invasion is more likely to result in colonization in older cows and in mammary glands, where there is delay in the arrival of PMNs at the invasion site. Death of PMNs and release of lysosomal enzymes cause further tissue damage. Fibrin plug formation in the smaller milk ducts may lead to involution of secretory tissue and loss of milk‐producing capacity (“agalactia”). Without treatment, the organism persists in the face of the host’s immune response, and the infection and mastitis become chronic. The antiphagocytic effect of capsular sialylated polysaccharide may be the important bacterial virulence factor in persistence. Much of the protective activity of colostrum against S. agalactiae has been shown to be associated with IgA and IgM. Serum antibodies appear to have little or no protective effect. Agglutinins in milk of infected cows, together with failure of bacterial clearance mechanisms in the udder, suggest that acquired immune responses are insufficient in clearance. The antigenicity of group B polysaccharide is greatly increased by conjugation to proteins, such as ovalbumin. Approaches to immunization of cattle include the use of surface glyceraldehyde‐3‐phosphate dehydrogenase protein plasmin receptor, and a chimeric cAMP antigen composed of epitopes of the S. agalactiae and S. uberis cAMP factors (Fontaine et al. 2002). This combination has shown some promise as a subunit vaccine against S. agalactiae mastitis. However, it is hoped that future research on local mammary immune responses will be helpful in design of more effective vaccines. Streptococci with the species designation dysgalactiae belong to Lancefield groups A, C, G, and L and are found as epithelial and mucosal commensals of most mammals and birds, and as opportunist pathogens of domestic animals and farmed fish. A reclassification, based on genotypic and phenotypic characterization, established the subspecies dysgalactiae and equisimilis, although phylogenetic relationships may be more complex (Jensen and Kilian 2012; Porcellato et al. 2021). Strains in subsp. dysgalactiae are Lancefield group C only, alpha‐hemolytic, and found mainly as a cause of acute and subclinical mastitis in cattle. Strains in subsp. equisimilis are beta‐hemolytic, may have either the A, C, G, or L group antigens, and cause disease in a variety of animal species including humans. Both S. dysgalactiae subspecies express numerous surface‐exposed and secreted proteins that bind to plasma or host tissue components. These streptococcal proteins include protein G, FnbA, FnbB, IgG‐, IgA‐, and α2‐macroglobulin receptor Mig, plasminogen receptor (GapC), and M‐like proteins, which bind IgG, fibronectin, α2‐macroglobulin, plasminogen, and fibrinogen, respectively (Vasi et al. 2000). Secreted virulence‐associated proteins include streptokinase, pyrogenic exotoxin G (SpeG), streptolysin O or S, streptodornase (DNase), and hyaluronidase. Immunization of cows with Mig and GapC reduces cell counts in milk following challenge (Bolton et al. 2004). GAPC‐immunized cows also showed reductions in the number of challenge bacteria in their milk. Release of hyaluronidase and fibrinolysin may be of value in tissue penetration and dissemination. Bovine sera contain an antibody specific for SpeG, and the recombinant protein stimulates proliferation of bovine peripheral blood mononuclear cells. However, its significance in the pathogenesis of mastitis is unknown. Infections of the mammary gland are usually associated with insect bites or other injury to the teat or udder epithelium, which facilitates direct access of surface‐exposed or secreted virulence bacterial proteins to their targets in the host. Since infections are opportunistic, cases occur sporadically with an acute clinical course. Trueperella pyogenes is frequently present as a synergist. S. dysgalactiae subsp. equisimilis includes group G and L strains from humans, and C and L strains from animals. Isolations of subsp. equisimilis are made only infrequently from horses, cattle, dogs, and cats. It is most frequently isolated from the joints of piglets that have acquired infection from sows that are tonsil carriers. Piglets are invaded via wounds including the umbilicus, or from the tonsil and develop suppurative arthritis following bacteremic spread. Subsp. equisimilis is occasionally isolated from abscessed lymph nodes of horses and from aborted placentas. The streptokinases of subsp. equisimilis show strict species‐specific plasminogen activation (i.e. porcine isolates activate porcine but not equine plasminogen; McCoy et al. 1991). Whole‐genome sequencing has shown that subsp. dysgalactiae is a distinct entity, although bovine and ovine isolates cluster separately (Porcellato et al. 2021). No single gene or genetic region differentiates these two clusters. By contrast, subsp. equisimilis was more heterogenous and could be generally differentiated according to host. Phylogenetic analysis of isolates showed that S. dysgalactiae were delineated according to source, suggesting the adaptive evolution of this species to several hosts (Porcellato et al. 2021). Interestingly, the majority of the genetic content specific for human‐associated subsp. equisimilis had high homology to genes harbored by the major streptococcal human pathogen, Streptococcus pyogenes. Not surprisingly, lineage specific virulence factors detected tended to cluster near to hotspots for integration of mobile genetic elements (Porcellato et al. 2021). S. uberis is a tonsillar, intestinal, mucosal, and epithelial commensal of cattle, responsible for about 20–30% of cases of clinical mastitis in dairy herds in North America, Europe, and Australia. It is distinguished from the phenotypically similar but rarely isolated S. parauberis by differences in its 16S and 23S rRNA genes and by the absence of genes for pauA and the oligopeptide permease OppF. Isolates of S. uberis vary in MLST and in protein phenotype between and within infected herds, although persisting infections in individual cows are usually caused by a single genotype. Many infections are opportunistic invasions of the mammary gland of older cows under conditions of heavy environmental soiling with feces. Analysis of the genomic sequence of S. uberis reveals a great variety of metabolic capabilities and nutritional flexibility but relatively few classical streptococcal virulence factors (Ward et al. 2009). Thus, it is well equipped to cope with a variety of environments, such as those encountered in the bovine intestine, the environment, or the mammary gland. Following entry through the teat canal, the organism attaches, proliferates, and induces an influx of neutrophils into the secretory acini that is evident in 24 hours. Activation of the local macrophage inflammasome by the serine protease Sub1154 is required for colonization and inflammatory response by macrophages (Archer et al. 2020). Colonization is nevertheless unaffected by the ensuing neutrophil invasion. This is followed by septal edema, vacuolation of secretory cells, necrosis of alveoli, and infiltration of septa by lymphocytes. As the disease progresses, there is hypertrophy of ductular epithelium, involution of glandular tissue, and early‐stage fibrosis. Streptococci are both free and within alveolar epithelial cells and macrophages in the alveolar lumina but are infrequent in neutrophils (Thomas et al. 1994). The organism is also present in lymphatic vessels and lymph nodes and attaches to ductular epithelium. The severity of the mastitis varies greatly and is determined by strain virulence, number of infecting organisms, season, immune status of the cow, parity, and stage of lactation. Infections in some herds are more common following drying‐off and more prevalent in cows with open teat canals. Cows with chronic infections often show a very slight inflammatory response and a normal humoral immune response. Resistance to phagocytosis and to the bactericidal activity of neutrophils is a hallmark of S. uberis infection. Although a hyaluronic acid capsule is expressed on a small percentage of isolates, the majority of isolates of S. uberis do not produce mucoid colonies, and so the capsule is not an essential virulence factor. Furthermore, hasA or C gene deletion mutants, although less resistant to phagocytosis by bovine neutrophils (Ward et al. 2009), remain pathogenic. Resistance to the bactericidal activity of neutrophils may be due to factors released by S. uberis and to binding of casein to the bacterial surface (Leigh and Field 1994). Three sortase‐anchored surface‐expressed proteins have been shown to play a role in virulence; these have homology with C5a peptidase, a lactoferrin‐binding protein and collagen‐like surface anchored protein (Leigh et al. 2010). Other potential virulence factors include hyaluronidase, a 28‐kDa uberis factor similar to the cAMP factor of S. agalactiae, an adhesin specific for cubic mammary gland cells (Lammers et al. 2001), the plasminogen activator PauA (Rosey et al. 1999), and the manganese scavenger lipoprotein MtuA. PauA, lactoferrin‐binding Lbp, SclB, and HasA are regulated by vru, deletion of which causes loss of virulence (Egan et al. 2012). Activation of plasmin may be important in the generation of essential amino acids from casein and in uncovering target sites for adhesins. S. uberis enters and survives within mammary epithelial cells for extended periods (Oliver et al. 1998). A novel adhesion fibrillar molecule, S. uberis adhesion molecule (112 kDa), with lactoferrin‐binding abilities, contributes to attachment and invasion of cells by serving as a bridge between epithelial receptors and the bacterium. Antibodies to this protein inhibit these activities. Comparative whole‐genome analysis of clinical and non‐clinical strains, including known virulent and avirulent isolates, did not identify simple virulence gene gain or loss that could explain these differences (Hossain et al. 2015). Specific immune responses have been shown to be involved in clearance of S. uberis from experimentally infected udders that had recovered from a previous infection (Hill 1988). Sera from infected cows react strongly with S. uberis surface proteins. Studies also indicate that milk becomes opsonic and growth inhibitory for S. uberis after mammary infection (Fang et al. 1998). Nevertheless, protection against infection is not dependent solely on opsonic activity and neutrophils. Vaccination with culture filtrates rich in PauA, or streptokinase from S. uberis, induced protection in about 50% of challenged quarters of immunized cows and was correlated with levels of PauA‐specific antibodies (Leigh et al. 1999). Interestingly, pauA is undergoing positive selection pressure that is possibly immunological (Zadoks et al. 2005). The Gap C protein, uberis cAMP factor, and SUAM have also shown some protective efficacy in vaccination challenge trials in dairy cows (Almeida et al. 2015). S. equi causes strangles, a highly contagious infection of the nasopharynx and associated lymph nodes of solipeds. Isolates constitute a clone or biovar of the closely related S. zooepidemicus (Holden et al. 2009). Although widely used in the literature since 1986, the designation S. equi subspecies equi is therefore incorrect. Core genome multilocus sequence typing (cgMLST) has shown that distinct populations of S. equi exist in the United States and Europe, but that international transmission occurs (Mitchell et al. 2021). The only known source of S. equi is a nasal discharge or pus from an abscess, or feed, water, or fomite directly contaminated by a shedding equid. Clinically inapparent carriers, although infrequent, are associated with persisting infection in resident horse populations or serve as vehicles of transfer to previously uninfected premises. Prolonged carriage is commonly associated with empyema and chondroids of the guttural pouch or cranial sinuses. Persisting infection of tonsillar tissue appears to be rare. Whole‐genome sequencing of 670 isolates from mostly European and North American sources and use of cgMLST identified the national and international transmission of this organism in sport horses (Mitchell et al. 2021). This fascinating study identified six major clusters (STs) containing 103 SeM alleles (discussed below) of genetically related strains. The incubation period varies from 3 to 14 days after exposure. Disease onset is marked by fever, lassitude, nasal discharge, slight cough, difficulty in swallowing, and swelling of the mandibular lymph nodes. Pressure of enlarging retropharyngeal nodes with abscessation and associated lymph stasis obstruct the airway, hence the common name of the disease. Metastasis may result in abscess formation in other locations, such as the lungs, abdomen, or brain. In older animals with residual immunity, strangles may present as the clinically atypical or catarrhal form. Strains of S. equi expressing bacteriophage‐encoded hyaluronidase have also been associated with a clinically mild form of strangles. The basic pathologic process in strangles is the outpouring of neutrophils in infected lymph nodes and tonsils and onto the upper respiratory mucosa, changes associated with purulent exudation and abscess formation. Most cases recover quickly and uneventfully. Sequelae include myocarditis, anemia, purpura hemorrhagica, and glomerulonephritis. These latter two sequelae involve the formation of circulating immune complexes. The known or putative virulence factors of S. equi include a nonantigenic hyaluronic acid capsule, hyaluronidase, streptolysin S, streptokinase, streptodornase, IgG Fc‐receptor proteins, ADP‐ribotransferase, pyrogenic exotoxins SePE‐I, H, L, and M, peptidoglycan, antiphagocytic SeM, Se18.9 and IdeE, equibactin, and fibronectin‐binding FNE E and B. A leukocidal toxin may also be produced. Isolates of S. equi are almost always highly encapsulated and produce very mucoid colonies. The constitutive expression of capsule on S. equi appears to be controlled by the Fas BCAX regulon (Velineni and Timoney 2018). In other streptococci, capsule synthesis is controlled by CovRS. Non‐encapsulated mutants are much less virulent for mice and horses. Capsule greatly reduces the number of streptococci that become associated with the surface of neutrophils and are subsequently ingested and killed. The negative charge and hydrophilicity of the bacterial surface is increased producing a localized reducing environment that protects oxygen‐labile proteases. Capsule is also required for the functionality of SeM and possibly other surface‐exposed proteins (Timoney et al. 2014). Streptokinase (Skc, plasminogen activator) released in vivo by S. equi interacts with the C‐terminal serine protease domain of equine plasminogen to form active plasmin, a serine protease that hydrolyzes fibrin (McCoy et al. 1991). A plasmin receptor is also present on S. equi. The lytic action of plasmin on fibrin and host matrix protein may aid in the spread and dispersion of the organism in tissue. Convalescent sera contain significant amounts of Skc‐specific antibody.
26
Streptococcus
Introduction
Characteristics of the Organism
Pathogenic Species
Species
Lancefield group
Diseases
Important virulence and virulence‐associated factors
Streptococcus agalactiae
B
Bovine mastitis; camel mastitis, invasive disease; occasional pathogen other species, including fish; human neonatal sepsis
Capsular polysaccharide; C, R, and X proteins; cAMP factor; hyaluronidase; lipoteichoic acid; proteases; CspA; collagenase; D‐alanylated lipoteichoic acid; neuraminidase; PilA, B and C; other surface proteins; C5a peptidase
Streptococcus dysgalactiae subsp. dysgalactiae
C
Bovine mastitis; septic arthritis in young lambs
Hyaluronidase; streptokinase; fibronectin binding proteins FnbA and B; protein G; plasminogen receptor; streptodornase; M‐like proteins; α‐2‐macroglobulin receptor
S. dysgalactiae subsp. equisimilis
A, C, G, L
Miscellaneous infections: arthritis piglets; pneumonia in kittens and puppies; cellulitis farmed fish; lymphadenitis; metritis, placentitis in Equidae
As for subsp. dysgalactiae but also including streptolysin S and O
Streptococcus equi
C
Strangles in Equidae
Capsular hyaluronic acid; antiphagocytic SeM, Se18.9 and IdeE; streptolysin S; pyrogenic exotoxins: streptokinase; peptidoglycan; fibronectin binding protein; proteases; tonsil binding SzPSe; streptokinase; equibactin
S. zooepidemicus
C
Opportunist pathogen in many species; includes septicemia, pneumonia, metritis
Capsular hyaluronic acid; streptokinase; proteases; streptolysin S; peptidoglycan; tonsil binding SzP protein; fibronectin binding proteins; IgG binding protein
Streptococcus suis
Meningoencephalitis; septicemia and arthritis in pigs
Capsule; MRP and EF proteins; suilysin; OFS, enolase, SAO, multiple adhesins, FHB, IgA proteases. Virulence complex and multifactorial
Streptococcus porcinus
E, P, U, V
Porcine cervical lymphadenitis
M protein; streptokinase; poorly characterized (not discussed further)
Streptococcus canis
G
Canine and feline opportunist pathogen; neonatal bacteremia; lymphadenitis of juvenile cats, guinea pigs. Opportunist pathogen in many species, including mink, aquatic mammals, humans
ScM protein; streptolysin O; poorly characterized. Bacteriophage encoded pokeweed‐mitogen linked to streptococcal toxic shock syndrome, necrotizing fasciitis dogs (STSS/NF) in dogs
Streptococcus iniae
Important pathogen of warm water farmed fin fish globally; zoonotic opportunist pathogen. Causes septicemia and meninoencephalitis
Capsule; phosphoglucomutase; Fc‐binding protein blocks immunoglobulin action; M‐like protein; C5a peptidase
Streptococcus uberis
Mastitis
Casein receptor; has A; cAMP‐like uberis factor; plasminogen activator PauA; serine protease (SUB1154), SUAM (adhesin), (bp lactoferrin binding), SclB (collagen‐like adhesins)
Streptococcus pneumoniae
Broncheolitis and pneumonia in horses in training. Unique capsular type 3 strain adapted to horses.
Capsular polysaccharide; neuraminidases; pneumolysin; autolysin; IgA protease; fibronectin binding proteins; peptide permeases; ZmpB metalloproteinase; choline binding proteins PsPA, LytA and CppA. Unique horse adapted strain has deletion in lytA and ply genes (not discussed further)
Streptococcus agalactiae
Virulence Factors and Pathogenomics
Pathogenesis
Immunity
Streptococcus dysgalactiae
Virulence Factors and Pathogenomics
Streptococcus uberis
Virulence Factors and Pathogenomics
Immunity
Streptococcus equi
Source of Infection: Ecology and Epidemiology
Types of Disease and Pathologic Changes
Virulence Factors and Pathogenomics
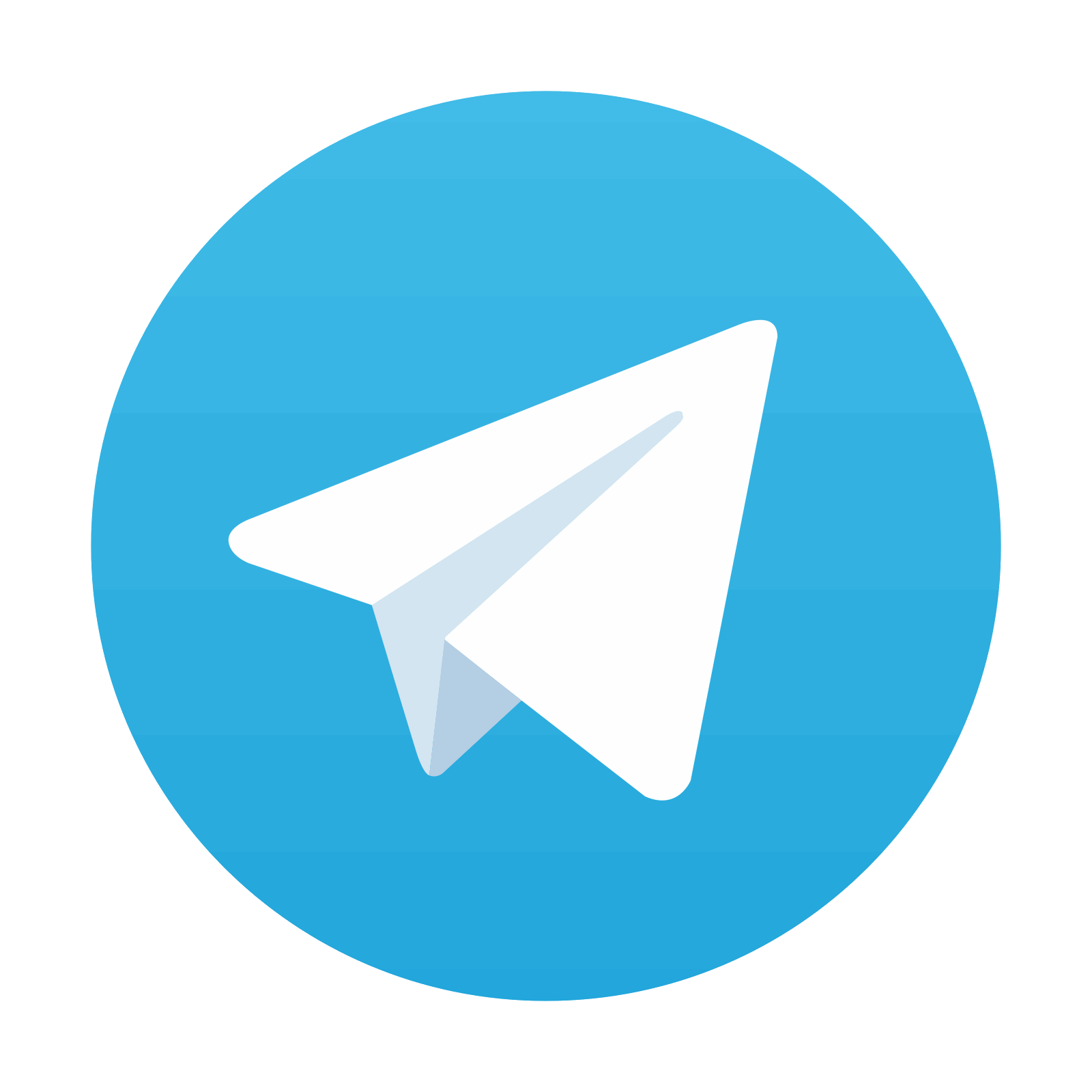
Stay updated, free articles. Join our Telegram channel

Full access? Get Clinical Tree
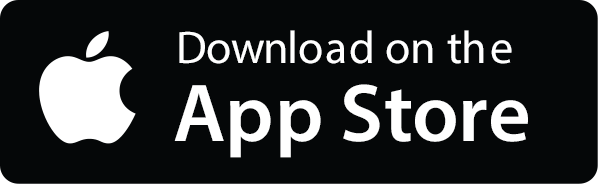
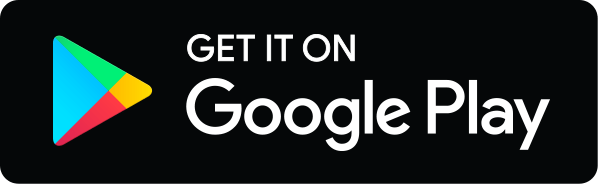