, Monika Hassel2 and Maura Grealy3
(1)
Centre of Organismal Studies, University of Heidelberg, Heidelberg, Germany
(2)
Spezielle Zoologie, Universität Marburg FB Biologie, Marburg, Germany
(3)
Pharmacology and Therapeutics, National University of Ireland Galway, Galway, Ireland
2.1 Stages of Development in Overview
2.1.1 Most Animals Pass Through an Embryonic Phase, a Larval Stage, Metamorphosis and an Adult Phase to Reach Eventually Sexual Maturity
In humans and in animals where offspring are generated through sexual reproduction the development of a new individual must be prepared in the gonads of both parents. The gonads must produce the gametes in processes subsumed under the term gametogenesis which occurs as oogenesis in females and spermatogenesis in males. In the female gonad, the ovary, oogenesis provides the egg cells, and in the male gonad, the testis, spermatogenesis supplies sperms. Details of oogenesis and spermatogenesis are described in Chap. 8.
The development of the new individual begins with fertilization. Fertilization is the fusion of two generative cells or gametes, the sperm and the egg cell (ovum), to form a zygote, the fertilized egg (Fig. 2.1). Sperm and egg cells are haploid; each contributes a single set of chromosomes, which is termed “paternal” in the sperm, and “maternal” in the egg cell. Gamete fusion provides the embryo with two complete genomes (the diploid set of chromosomes), a source of information sufficient to construct the new organism. In addition, fertilization induces the activation of the resting egg cell (Chap. 3). Only upon activation can the egg undergo embryogenesis.
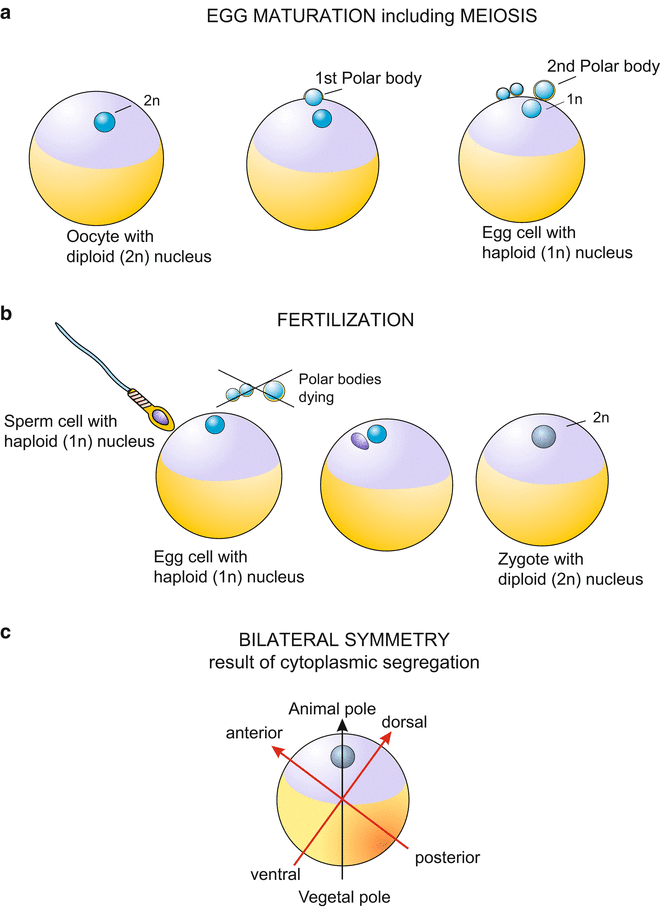
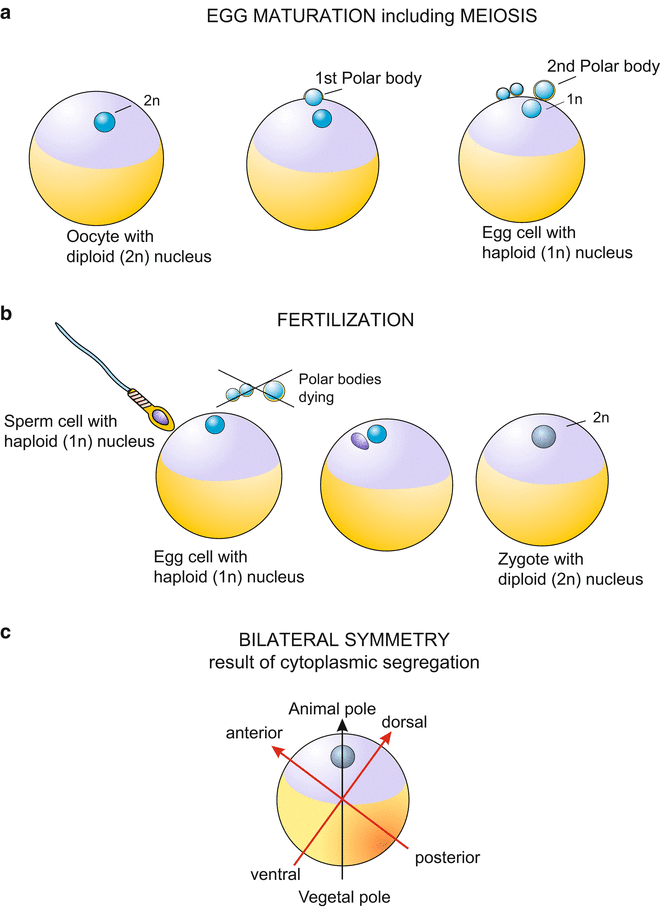
Fig. 2.1
General diagram showing egg maturation and meiosis, fertilization and establishment of the body axes, exemplified by the amphibian germ. Diagonally opposite to the point of the sperm entry into the egg the future back line will extend
Embryogenesis follows fertilization: within a protective envelope, and in viviparous organisms such as humans within the maternal body, numerous cells are produced from the zygote by continuous mitotic divisions. The resulting cells stay together, collectively modelling the basic architecture of the new organism, and provide it with all the essential organs necessary to begin an autonomous life when the young animal hatches out of the envelope or the mother gives birth. In the majority of animals, development occurs in the so called indirect fashion: embryogenesis results in a first phenotype, the larva. An example is the sea urchin, which can be taken as prototype of animal developments (Fig. 4.2). The larva itself undergoes development, which usually brings about only minor, unobtrusive changes, followed by a dramatic metamorphosis to a new phenotype, which is termed imago or adult, and which typically settles in an ecological niche different from that in which the larva lived. Larva and adult exploit different nutritive resources in their environments. Some groups of animals, among them the vertebrates, circumvent a larval state; in direct development the adult phenotype arises from the embryo step by step.
After a juvenile phase, life culminates when the adult reaches sexual maturity. Senescence finishes the phase of sexual maturity, and death ends the life of the somatic cells of the individual. Before death, its generative cells should have passed life on to a new generation.
2.1.2 The Egg Cell Contains, Besides the DNA of the Nucleus, Also Cytoplasmic Sources of Inherited Information; Structurally the Egg Becomes Polar, That is Asymmetrically Organized
Eggs contain yolk, a rich source of energy and molecular building materials. This is well known. Proposals to clone species which are about to become extinct, or even to clone prehistoric animals by removing nuclei from somatic cells of relics and implanting them into enucleated eggs of other, present day species show that knowledge about the various sources of inherited information is not very common. Although the DNA in the nucleus is the main source of information, it is not the only source, and not the only of significance. Ever since embryologists made surgical operations with eggs or early embryonic stages (e.g. T Boveri, EB Wilson, and EG Conklin; Chap. 1, Box 1.1), they became aware that eggs contain regulative determinants in their cytoplasm. In general terms these regulatory constituents are called cytoplasmic determinants. Pioneering work has been done with eggs of Drosophila (Sect. 4.6) and Xenopus (Sect. 5.1). Using refined techniques and the methodology of molecular biology these determinants are now being identified.
Essential constituents are mRNA’s that code for proteins with regulatory function, collectively referred to as transcription factors. Such factors control gene activities. Since these mRNA’s are produced by cells of the maternal organism and, therefore, are derived from genes of the mother they became known as maternal RNA’s. The producing cells are the oocytes themselves or nursing cells in their neighbourhood: Their products (mRNA or proteins coded from them) are deposited in the egg in the course of oogenesis. Insofar as these exert regulatory functions one speaks also of maternal information or maternal determinants.
Effects of such stored cytoplasmic determinants become apparent when the Mendelian rule of reciprocity is invalidated and the genetic constitution of the mother is of particular significance (e.g. hinny compared to mule, lefthand or righthand coiling in snails, Fig. 4.21).
One of the first identified cytoplasmic determinants is the mRNA of the gene bicoid in the egg of the fruitfly Drosophila (this maternal mRNA is indispensable but specific for Drosophila melanogaster and closely related flies, Sect. 4.6). Several of such maternal factors have also been found in the eggs of the African clawed frog Xenopus (Sect. 5.1). According to present knowledge each animal egg harbours a collection of such maternal constituents, arranged in a three-dimensional pattern. As a rule, cytoplasmic determinants are not uniformly distributed in the egg but concentrated at defined sites. Experimentally working embryologists have to take the internal structure of the egg, the location of its cytoplasmic constituents and their possible species-specificity into consideration. In this introductory section we take a first look at the morphology of the animal egg without having an eye to ultramicroscopic details and without referring to biochemical data.
Animal-Vegetal Polarity.
Even when the egg is spherical rather than elongated and elliptically shaped (the eggs of insects are elliptic, for example), in its internal structure an egg cell is always anisotropic or asymmetrical. In the terminology used in biology, an egg has a polar structure. At the very least, this polarity (anisotropy) is expressed in the location of the nucleus. Even in the immature oocyte (the diploid precursor cell) the nucleus is usually not centrally located but in the periphery near the surface of the cell. Because of its large size the nucleus of the oocyte is traditionally referred to as “germinal vesicle”. In the course of the meiotic divisions which give rise to the mature egg, the germinal vesicle apparently disappears as it undergoes the two meiotic divisions. As a consequence of these divisions the polar bodies are formed at this peripheral location (Fig. 2.1). Polar bodies are miniature sister cells of the egg cell.
The location where the polar bodies are pinched off is usually shown as the ‘North Pole’ of the egg and is called the animal pole. The opposite ‘South Pole’ is named the vegetative pole or vegetal pole. Material is generally deposited at the vegetative pole which later in development is used in the formation of the primordial gut (archenteron), or is incorporated into the lumen of the gut.
In this context, the adjective ‘animal’ refers to typical animal organs such as eyes or the central nervous system, which often are formed in the vicinity of the egg’s animal pole. The adjective ‘vegetal’ refers to the future ‘vegetative’ organs which derive from the primordial gut and serve ‘lower’ functions of life such as processing of food. The axis which extends from the ‘North Pole’ to the ‘South Pole’, and passes through the centre of the globe is termed the animal-vegetal egg axis.
However, when the site where the archenteron will be formed coincides with the location where the polar bodies are pinched off rather than being opposed to it, as for example in Cnidarians (Fig. 22.7), the traditional terminology often caused confusion and gave rise to erroneously oriented and labelled illustrations of eggs and embryos.
Bilateral Symmetry.
Following fertilization the interior of the egg starts moving. Many constituents are rearranged and in the course of this ooplasmatic segregation the vast majority of animal eggs becomes organized in a bilaterally symmetrical way (Fig. 2.1c). In the amphibian egg, the prototype of a vertebrate egg, two polarity axes can be made out at this stage and one can distinguish between anterior or cranial, and posterior or caudal, and between the site of the future back (dorsal) and future belly (ventral). The egg shows bilateral symmetry. In insects the egg is already bilaterally symmetrical before fertilization.
Covers and Distribution of Yolk.
Animal egg cells are surrounded by stabilizing and protective acellular envelopes. There are only a few exceptions; for instance the eggs of Cnidarians are covered only by an unobtrusive halo of glycoproteins. The innermost acellular sheet, which directly covers the surface of the egg cell, is made of glycoproteins and termed the vitelline membrane (this is not a phospholipid bilayer as is the cell membrane but a thin sheet composed of hydrophilic glycoproteins). In mammals the envelope of the egg is called the zona pellucida, in amphibians and fishes the jelly covering, and in insects the chorion. (The term chorion can refer to different, non-homologous structures. While in insects ‘chorion’ designates the acellular envelope of the egg; in reptiles, birds and mammals ‘chorion’ means a cellular ‘extraembryonic’ epithelial structure which is made by the embryo itself.)
Zoological textbooks like to list classifying terms, which inform readers familiar with Ancient Greek about the amount and distribution of yolk (end syllable –lecithal) in the egg. Prefixes are: oligo = few or little, poly = much or many, iso = uniform, centro = in the middle, telo = at the end, concentrated at one of the poles. Amount and distribution of the yolk affect the type and pattern of cleavage.
2.1.3 Cleavage Is a Series of Rapid Cell Divisions
Fertilization and activation of the egg are followed by cleavage (Figs. 2.2, 2.3, and 2.4). After fusion with the sperm, the fertilized egg, the diploid zygote, is still unicellular. Its task is to give rise to a multicellular organism that may comprise many millions of cells. There now follows a series of rapid cell divisions. At high speed the zygote is divided, without increase in volume and mass, into more and more cells which therefore are smaller and smaller. This stage of development is called cleavage. It is indicated by the appearance of furrows on the surface of the egg, for example on the surface of the yellow egg sphere of the chicken, indicative of proceeding cell divisions.
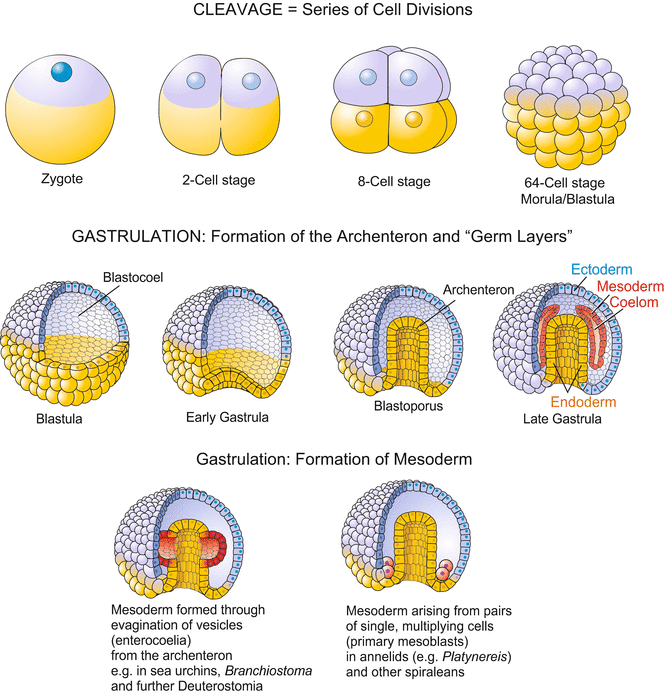
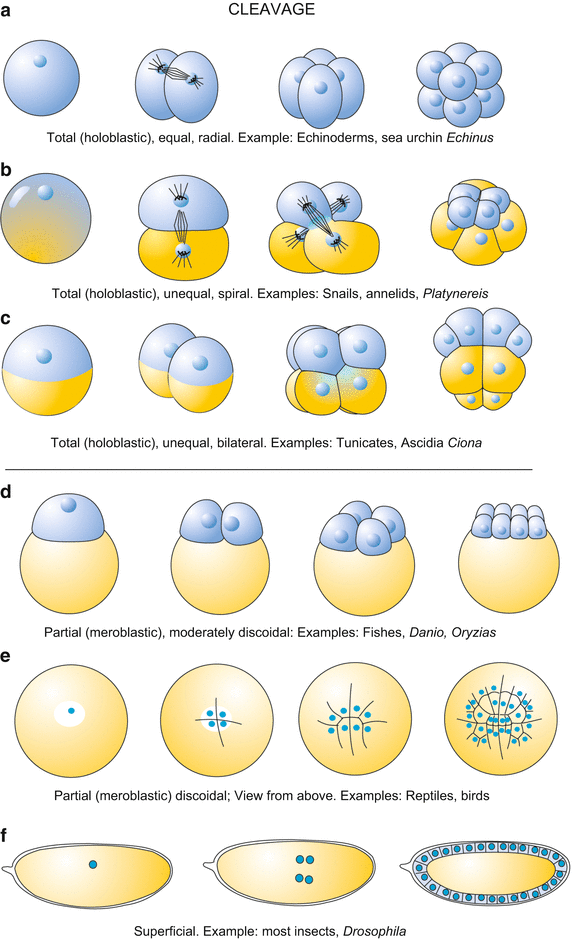
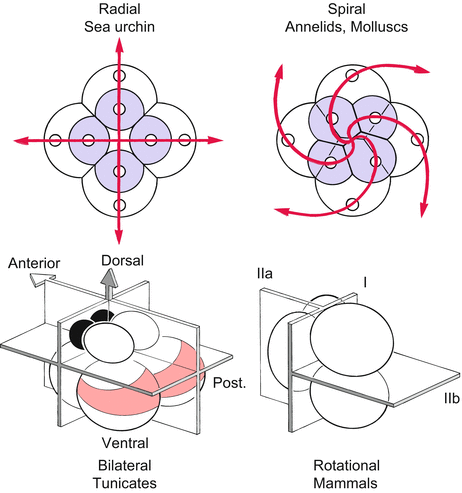
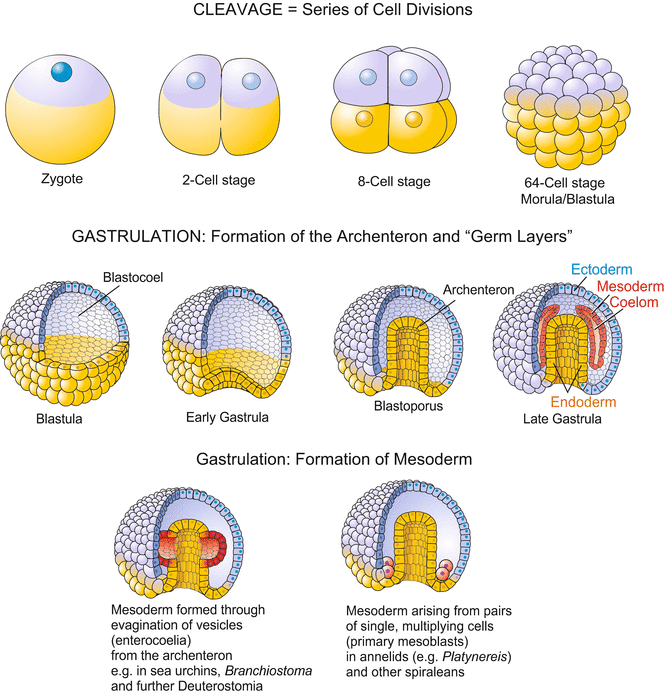
Fig. 2.2
Schematic overview of early embryonic stages. The illustration basically follows the development of the Deuterostomia (sea urchin, amphibians) showing the primary mouth (blastopore) at the vegetal pole. The formation of the mesoderm can occur in different ways. The illustration shows two basic types
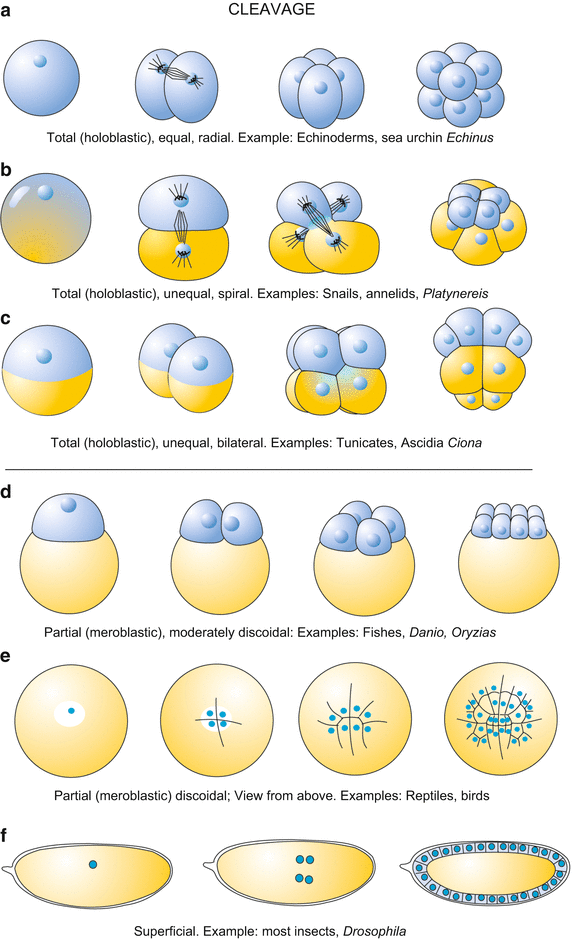
Fig. 2.3
Overview showing the most frequent modes of cleavage. (a) In radial cleavage the blastomeres come to lie in columns, one in top of the other. (b) In spiral cleavage (e.g. in annelids) in each division the daughter cells become displaced to the right or to the left at angles to the vertical axes caused by the oblique orientation of the mitotic spindle. (c) The bilateral cleavage (displayed for instance by the urochordates) produces a bilaterally symmetrical germ starting with the first division. (d, e) In eggs rich in dense yolk, as for example in those of birds, cleavage membranes initially separate the yolk mass incompletely. In the central area cells become entirely surrounded with a cell membrane first and thus complete cells occupy only the central area while in the periphery cells are still incomplete. The flat blastodisc lies on top of the yolk mass. (f) The superficial cleavage in Drosophila consists initially of division of nuclei only. Division of nuclei continues until 254 nuclei are present; these begin to migrate into the periphery to form the cellular blastoderm. Conventional cells are formed by wrapping cell membranes around the nucleus and a portion of cytoplasm
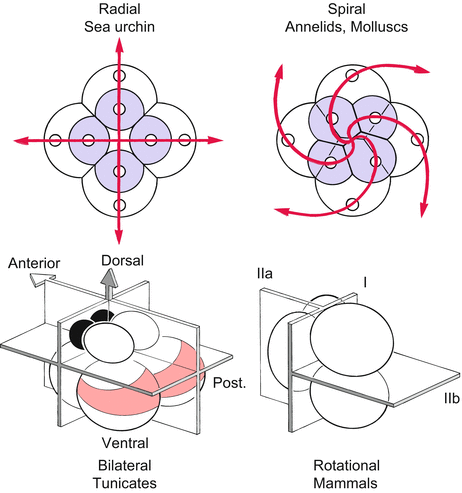
Fig. 2.4
Types of cleavage according to the spatial pattern in which the first blastomeres become arranged. Holoblastic eggs usually contain little yolk and are entirely cleaved during cell division (cytokinesis). Radial and spiral cleavage patterns are most conveniently recognized looking down upon the top (animal pole) of the developing embryo. The bilateral and rotational cleavages are shown from a side view
Cleavage can be
holoblastic (=total); (Greek: holos = whole, blastos = germ-bud, seedling, embryo): the egg is completely subdivided into individual cells (Figs. 2.2 and 2.3a–c); or it can be
meroblastic (=partial); (Greek: meros = part of), because of the huge amount of bulky yolk the egg is not divided in complete membrane-enclosed cells (Fig. 2.3d–f), at least not at the beginning of its development.
Whether or not holoblastic cleavage with regular cell divisions is possible depends on its spatial dimensions and its content of yolk. Where holoblastic cleavage occurs the first daughter cells are called blastomeres. Depending on whether the first daughter cells are equal or unequal in size, we refer to equal or unequal cleavage. Where unequal cleavage occurs a blastomere may give rise to large macromeres and small micromeres. Examples of meroblastic cleavage are the superficial cleavage in insects (Figs. 2.3f and 4.26), and the discoidal cleavage in fishes (Figs. 2.3d and 5.22), reptiles and birds (Figs. 2.3 and 5.22).
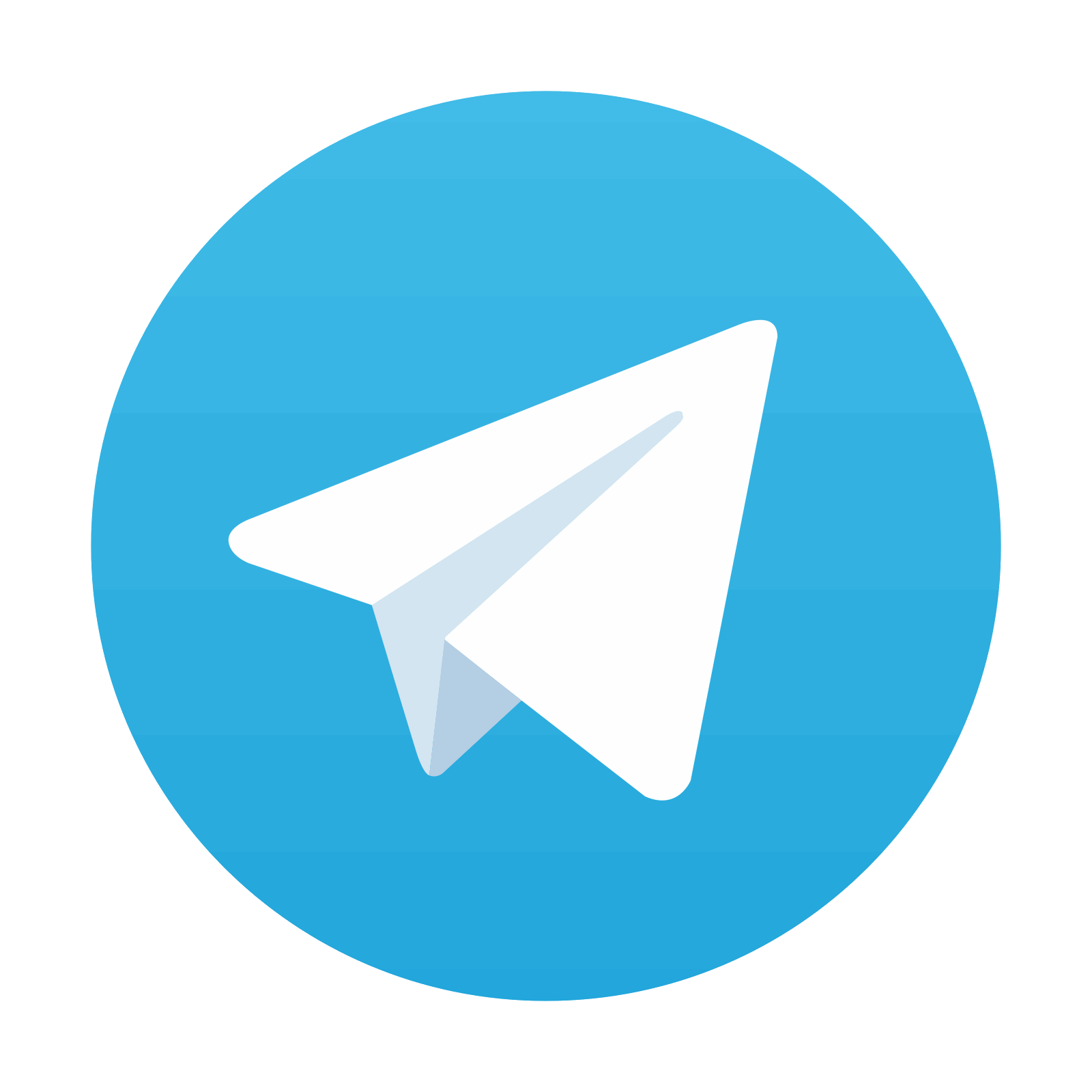
Stay updated, free articles. Join our Telegram channel

Full access? Get Clinical Tree
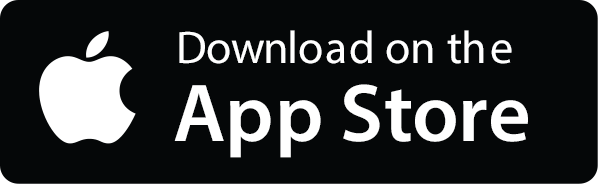
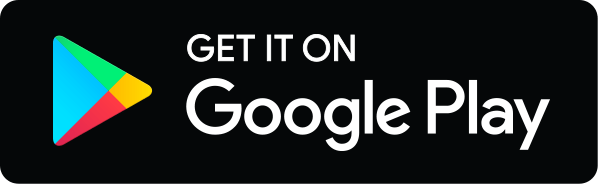