The spinal cord and the brain constitute the central nervous system. The spinal cord is enclosed within the vertebral canal, as are dorsal and ventral spinal roots that belong to the peripheral nervous system (Fig. 16-1). By means of spinal roots and spinal nerves, the spinal cord innervates the neck, trunk and tail, the limbs, and the caudal and dorsal surfaces of the head. Dorsal roots convey sensory (afferent) input to the spinal cord, whereas ventral roots carry motor (efferent) output from the spinal cord to muscles and glands. The spinal cord performs three general functions: 1. Via spinal nerve connections, it processes afferent information from muscles, tendons, joints, ligaments, blood vessels, skin, and viscera, and it discharges efferent commands that control muscles and regulate glands. 2. The spinal cord is a reflex center, producing subconscious responses of muscles and glands to particular stimuli. 3. The spinal cord conducts information to and from the brain through a system of axonal tracts, by which the brain receives status information about the neck, trunk and limbs while dispensing commands that control posture, movement, and the visceral aspects of behavior. Within the vertebral canal, the spinal cord and spinal roots are enveloped by three protective layers termed meninges (Fig. 16-2). The dura mater, the most superficial meningeal coat, is fibrous and thick. It forms a cylinder surrounding the spinal cord, and through lateral extensions it and the other meningeal layers ensheathe spinal roots. The thin arachnoid membrane lines the inner surface of the dura mater. A subarachnoid space containing cerebrospinal fluid is located deep to the arachnoid membrane. Arachnoid trabeculations traverse this space to attach to the pia mater. The pia mater, the deepest, most vascular meninx, is bound to glial cells concentrated at the spinal cord surface (glial limiting membrane) (Uehara & Ueshima, 1988). The pia mater is thickened bilaterally along the lateral margin of the spinal cord, forming denticulate ligaments. Each ligament has periodic lateral extensions that attach to dura mater, thereby suspending the spinal cord such that it is surrounded by cerebrospinal fluid (Fig. 16-3). In transverse sections, gray matter appears butterfly shaped, having bilateral wings connected across the midline by central intermediate substance (Fig. 16-3). The central intermediate substance (substantia intermedia centralis) surrounds the central canal and includes the gray commissure (commissura grisea), which traverses the midline dorsal and ventral to the central canal. The bilateral extension of intermediate substance into each gray matter “wing” is called lateral intermediate substance (substantia intermedia lateralis). The spinal cord features septae, sulci, and fissures, which are useful as landmarks (Fig. 16-3). A septum is a thin barrier formed principally by astrocytes in white matter. A sulcus is a shallow groove on the spinal cord surface. A fissure is a midline cleft typically lined by pia mater. The basis for dividing the spinal cord into segments is the attachments of dorsal (or ventral) roots. Each dorsal or ventral spinal root (radix dorsalis; radix ventralis) is composed of thousands of axons with varying amounts of Schwann cell myelin and enveloped by meninges. The axons of each root are bound together laterally where dorsal and ventral roots join to form the spinal nerve, but as roots approach the spinal cord, their axons regroup into separate bundles called rootlets (fila radicularia). The rootlets attach serially along the spinal cord (Fig. 16-4). Caudal, formerly coccygeal, roots may have only 1 or 2 rootlets, whereas segments innervating limbs may have 12 or more rootlets per dorsal or ventral root (Fletcher & Kitchell, 1966a). At the two locations, where nerves to the limbs arise, the relative diameter of the spinal cord is increased. The cervical enlargement (intumescentia cervicalis) gives rise to spinal nerves that form the brachial plexus that innervates the thoracic limb. The cervical enlargement involves part of segment C6, segments C7 and C8, and part of segment T1. The lumbar (lumbosacral) enlargement (intumescentia lumbalis), which innervates the pelvic cavity and pelvic limbs, involves part of segment L5, segments L6 and L7, and part of segment S1 (Fletcher & Kitchell, 1966a). Caudal to the lumbar enlargement, the spinal cord tapers into an elongate cone, designated conus medullaris. This region consists of segments S2, 3 and Ca l to 5. These segments appear successively smaller, and they are surrounded by caudally directed spinal roots (Fig. 16-5). Approximately 1 cm caudal to the last segment (spinal cord termination), the spinal cord is reduced to a uniform strand of glial and ependymal cells called the terminal filament (filum terminale) encased in a layer of pia mater. A caudal extension of dura mater that envelops the filum terminale is called the spinal dura mater filament (filum durae matris spinalis). This filament extends caudally in the vertebral canal where it attaches to a sacral or caudal vertebra. A dura-arachnoid sac, enclosing subarachnoid space and cerebrospinal fluid in a lumbar cistern, extends approximately 2 cm caudal to the end of the spinal cord neural parenchyma. Caudal to this the dura mater constricts around the filum terminale. At the level of the filum terminale, leakage may occur between the central canal and the narrowed subarachnoid space (Marin-Garcia et al., 1995). Within the vertebral canal, sacral and caudal spinal roots stream caudally, beyond the conus medullaris, to exit at their respective intervertebral foramina. Collectively, these roots are designated cauda equina. In the dog, most of the cauda equina lies caudal to the lumbar cistern; the roots are individually enveloped by meningeal sheaths (Fletcher & Kitchell, 1966a). At birth, the canine spinal cord extends into the sacrum. Following postnatal development, the spinal cord terminates in the caudal lumbar region. Overall, the vertebral column outgrows the spinal cord in length; however, the degree of elongate growth of spinal cord segments is regionally variable (Fletcher & Kitchell, 1966a). As a result, the positional relationship between spinal cord segments and vertebrae is regionally variable. In certain experimental and clinical situations, it is necessary to locate spinal cord segments with respect to more readily identifiable vertebrae. Spinal cord segments alternately lengthen, shorten, lengthen, and shorten again along the extent of the spinal cord (Fig. 16-4). The longest spinal cord segment is C3; caudal to it, segment length declines, reaching a minimum at the level of segment T2. Caudal to this, thoracic segments lengthen, particularly by increased distance between adjacent roots. Segments of the thoracolumbar junction are relatively long. Caudal to these, segments become progressively shorter. Thus spinal cord segments display considerable differential growth relative to more subtle changes in vertebral length. With respect to position within the vertebral column, four spinal cord regions can be distinguished (Fig. 16-4): (1) an initial cervical region, where at least the first cervical segment lies within its corresponding vertebra; (2) a caudal cervical through cranial thoracic region, where segments are positioned cranial to their respective vertebrae; (3) a thoracolumbar junction, where segments again lie within their corresponding vertebrae; and (4) a caudal lumbar, sacral, caudal region, where segments lie progressively cranial to their respective vertebrae (Fletcher & Kitchell, 1966a). Positions of spinal cord segments relative to vertebrae may vary by half a vertebral length cranial or caudal to the typical relationship for medium-sized and large dogs, depicted in Figure 16-4. In these dogs, the conus medullaris ends approximately at the level of the L6-7 intervertebral disc. Small dogs (weighing less than 7 kg) have relatively longer spinal cords, particularly evident in the lumbosacral and caudal regions, where segments may be one vertebra caudal to the relationship illustrated in Figure 16-5. Because spinal roots always travel to intervertebral foramina formed by corresponding vertebrae, spinal root length reflects the location of a spinal cord segment relative to its numerically corresponding vertebra. Spinal roots are short in initial cervical and thoracolumbar junction regions, where segments lie near to or their corresponding vertebrae. Roots are longer where segments are displaced cranial to corresponding vertebrae, as a result of segment shortening and differential growth between the spinal cord segments and the vertebrae (Fig. 16-6). The first spinal nerve exits the vertebral canal through a lateral vertebral foramen, located in the dorsal arch of the atlas. Spinal nerves C2 through C7 exit through intervertebral foramina formed by cranial margins of corresponding (C2-7) vertebrae. Because it lacks a corresponding vertebra, the C8 spinal nerve exits through the intervertebral foramen formed by the cranial margin of vertebra T1. The remaining spinal nerves, T1 to Ca5, exit through intervertebral foramina formed by caudal margins of their corresponding vertebrae (Fig. 16-4). The relationship between the spinal cord dorsoventral diameter to the dorsoventral diameter of the vertebral canal is important in assessing lesions that have the potential of compressing the spinal cord. Based on measurements made on cervical myelograms, small breeds have a spinal cord to vertebral canal higher ratio than large breeds (Fourie & Kirberger, 1999). Spinal cord interneurons are interposed between a particular input and the resulting output from the spinal cord. In the process of “wiring” connections between input and output, interneurons establish elementary patterns of motor neuron output that can be used in such activities as spinal reflexes, locomotion, and other voluntary movement. Interneurons may be activated by synaptic input arriving from primary afferent neurons, from caudally projecting pathways (coming from the brain), from other interneurons, and from axonal branches of efferent neurons. Different reflexes and caudally projecting paths may share the same interneurons (Hongo et al., 1989). Spinal cord projection neurons send axons into white matter to form, generally, cranial projecting pathways to the brain. The projection neurons are activated by primary afferent neurons that become excited in response to stimulation of viscera, muscles, joints, or skin. Primary afferent neurons influence the excitability of projection neurons directly or through interneurons or other projection neurons (Willis, 1985, 1986; Yaksh, 1986). The excitability of spinal cord projection neurons is also modified, directly or through interneurons, by caudally projecting axons from brain projection neurons (Noble & Riddell, 1989). Spinal cord projection neurons may be characterized by the nature of the peripheral stimulation that ultimately activates them. Some neurons respond specifically to noxious stimuli, or specifically to touch and pressure stimuli, or specifically to thermal stimuli. Neurons that respond to a specific stimulus function in modality identification and precise localization of the stimulus (Yaksh, 1986). Many projection neurons respond nonspecifically to both mild mechanical stimulation and mechanical or thermal noxious stimulation (Willis, 1986). Nonspecific neurons have a wide dynamic range of responses and appear specialized to code the intensity of stimulation. These multireceptive neurons have relatively large receptive fields. Commonly, the nociceptive sensitivity and receptive field size of multireceptive neurons are subject to modification by caudally projecting tracts. The majority of spinal cord projection neurons respond just to somatic stimulation (skin or muscles and joints), but others respond to both somatic and visceral stimulation (Cervero & Lumb, 1988). Somatic efferent neurons innervate skeletal muscles. Cell bodies of the neurons are located in the ventral horn, grouped into motor nuclei. Somatic neurons that innervate typical muscle fibers responsible for producing muscle tension are referred to as alpha motor neurons. In a clinical context, these neurons are referred to as lower motor neurons—the motor neuron with an axon that leaves the central nervous system to innervate the target organ. Their destruction results in flaccid paralysis of the muscles innervated (Brooks, 1986). A motor neuron and all of the muscle fibers it innervates constitute a motor unit. All motor units of a particular skeletal muscle have their neuronal cell bodies grouped together, forming a motor neuron pool within a motor nucleus within the ventral horn. Motor units vary in their properties. Small motor units (relatively small alpha motor neurons innervating relatively few muscle fibers) are typically activated earliest during muscle contraction; they produce small degrees of tension and contract relatively slowly, and their muscle fibers are highly resistant to fatigue. At the other extreme, the largest motor units are recruited only during maximal muscle contraction; their muscle fibers contract rapidly and are easily fatigued (Binder & Mendell, 1990; Henneman et al., 1965). In addition to the conventional organization of neuronal cell bodies in the core of the spinal cord, a very small population of neuronal cell bodies and their processes have been found in a subpial position primarily in the segments of the spinal cord enlargements. Here they predominate on the dorsolateral surface of the white matter where the processes form a subpial plexus (Fedorets, 2001). Two schemes are currently used for categorizing spinal cord neurons into functionally significant groups (Fig. 16-7). One approach involves identification of cell columns (nuclei). This scheme works for some functionally specific nuclei; however, it omits many neurons that are morphologically dispersed. The other approach involves dividing spinal cord gray matter into ten laminae (Rexed, 1952, 1954). The laminar classification scheme accounts for all spinal cord neurons; however, it is usually difficult to distinguish individual lamina in routine preparations. In general, both laminar and nuclear schemes are used, depending on which applies best to a specific situation. The lateral cervical nucleus (nucleus cervicalis lateralis) is found in the first two cervical segments of the spinal cord (Brodal & Rexed, 1953; Ha & Liu, 1966). Lateral to the dorsal horn, the profile of the nucleus forms a peninsula or island surrounded by white matter. The nucleus consists of projection neurons (third-order neurons of the spinocervicothalamic pathway). The nucleus relays cutaneous noxious stimuli and touch to conscious centers (Lu & Yang, 1989). In this text nociception is the response of an animal to a noxious stimulus, one that causes injury or has the potential to cause injury. This response indicates a patient’s discomfort or pain caused by the noxious stimulus. Pain is not a sensory modality. It is the conscious response to a noxious stimulus. Marginal nucleus (dorsomarginal nucleus) refers to flattened neurons located at the dorsal surface of the dorsal horn along the entire length of the spinal cord. Although the nucleus is not morphologically prominent, it is important as a site of nociceptive projection neurons (Craig et al., 1988; Yaksh, 1986). The substantia gelatinosa, a concentration of small neurons, forms a homogeneous crown at the apex of the dorsal horn, deep to the marginal nucleus. It extends the entire length of the spinal cord and blends cranially with the nucleus of the spinal tract of the trigeminal nerve. Most substantia gelatinosa cells are interneurons that project to the remainder of the dorsal horn, but a few larger cells are spinothalamic tract projection neurons. The substantia gelatinosa receives cutaneous input, from axons activated by noxious, tactile, or thermal stimulation (Light & Kavookjian, 1988; Rethelyi et al., 1989). The nucleus thoracicus (nucleus dorsalis; nucleus of the dorsal spinocerebellar tract; Clarke column) consists of sparse, large cell bodies located medially at the base of the dorsal horn of T1 through L4 spinal cord segments. The nucleus thoracicus receives input from muscles caudal to the thoracic limbs. The nucleus is composed of projection neurons that give rise to the ipsilateral dorsal spinocerebellar tract, which projects to the cerebellum and to nucleus Z of the medulla oblongata (McIntyre et al., 1989). The intermediolateral nucleus is located at the lateral margin of the lateral intermediate substance of thoracic and cranial lumbar levels of the spinal cord. The nucleus forms the lateral horn of segments T1 through L3. The nucleus contains preganglionic sympathetic neurons (Cummings, 1969). Embryologically, the neurons occupy a medial position, but subsequent migration positions the majority of cell bodies laterally. The sacral parasympathetic nucleus is found in the sacral spinal cord segments, chiefly S2 and S3 (Oliver et al., 1969; Purinton & Oliver, 1979). It consists of parasympathetic preganglionic neurons that form a mediolateral band in the lateral intermediate substance. The dorsal portion of the nucleus is concerned with bowel control, the lateral portion with urinary bladder contraction (Leedy et al., 1988). The cell bodies of alpha and gamma efferent neurons that innervate a particular skeletal muscle are grouped together, forming a motor neuron pool. Related skeletal muscles have their motor neuron pools grouped into longitudinal columns that extend over one or more spinal cord segments (Horcholle-Bossavit et al., 1988). These columns are designated motor nuclei when viewed in spinal cord sections. From one to seven motor nuclei are evident in the different segments along the length of the spinal cord (Romanes, 1951). Clusters of neuronal cell bodies located laterally in the ventral horn are designated lateral motor nuclei. They innervate limb musculature. In cervical segments, a lateral motor nucleus of the accessory nerve (nucleus motorius n. accessorii) gives rise to the spinal roots of the accessory nerve. Lateral motor nuclei are best developed in the enlargement segments, which innervate limbs. In these segments, the nuclei are somatotopically arranged, relative to the locations of the muscles they innervate. For example, proximal to distal muscles of the pelvic limb are innervated by ventral to dorsal nuclei, and the most lateral nuclei supply cranial limb muscles, whereas the more medial nuclei innervate caudal muscles of the limb (Fig. 16-8). Dorsal root afferent axons enter the spinal cord by penetrating the dorsolateral sulcus, which marks the separation between dorsal and lateral funiculi. Within the dorsal funiculus, afferent axons typically bifurcate into longer cranial and shorter caudal branches (Fig. 16-9). These branches, in turn, give off collateral branches that penetrate gray matter to synapse on projection neurons, interneurons, and, in some cases, motor neurons. The number of segments (generally two to eight) over which cranial and caudal branches extend and give off collaterals is proportional to the size of the receptive field of the primary afferent neuron.
Spinal Cord and Meninges
The Spinal Cord
Morphologic Features of the Spinal Cord
Spinal Cord Segments
Segmental Relationships to Vertebrae
Gray Matter of the Spinal Cord*
Gray Matter Organization
Gray Matter Nuclei
Dorsal Horn Nuclei
Intermediate Substance Nuclei
Ventral Horn Nuclei
White Matter of the Spinal Cord
< div class='tao-gold-member'>
Stay updated, free articles. Join our Telegram channel

Full access? Get Clinical Tree
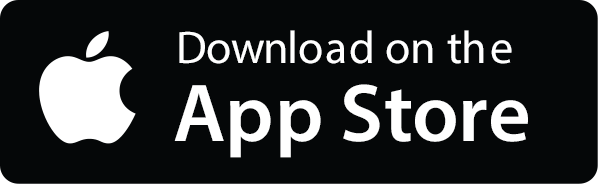
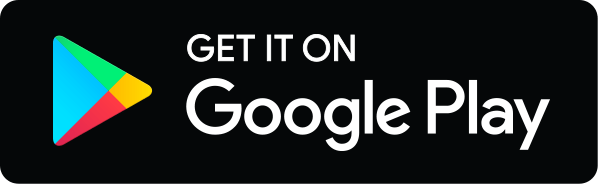