12.1 Background and development
The embryonic development of the eye is similar in all species. The retina and pigment layers in the eye derive from neurectoderm, the lens and cornea from surface ectoderm and the uvea and sclera from mesoderm. Initially the optic vesicle forms from bilateral outpouchings of forebrain neurectoderm. The optic vesicle expands retaining a connective stalk to the developing brain, which will become the optic nerve. The surface ectoderm overlying the optic vesicle thickens to form the lens placode, which subsequently invaginates into the optic vesicle. The surface ectoderm differentiates into two regions which will ultimately form the lens (lens vesicle) and the cornea and eyelids. The surrounding mesoderm condenses to form the outer uveal and sclera coats. Detailed descriptions and timing of these changes can be found in Kaufmann and Bard (1999).
The mouse eye is not fully developed at birth and a detailed description of the changes that occur is beyond the scope of this chapter but is reported by Smith et al. 2002a. Briefly, the mouse is born with closed eyelids, which open at day 12–14 postnatally. All structures in the eye show immature features including increased stromal density, presence of hyaloid vasculature, compressed appearance of retinal layers and rapid lens growth including mitotic activity) (Figure 12.1). As development of the eye is largely complete by 3 weeks of age, with the exception of retained remnants of the hyaloid blood vessels, retention of immature features after this time may be considered unusual.
12.2 Sampling technique for the eye
There are a number of approaches to sampling the eye and associated intraorbital glands (intraorbital lacrimal gland and Harderian gland). The head may be fixed whole and the eyes left in the head and sectioned with a whole head cross-section following decalcification (Figure 12.2a) or the eyes may be removed by enucleation (Smith et al. 2002a). Sectioning the eyes in the head has the virtue of being technically easier at necropsy, although it may not be possible to get a complete section through the globe and optic nerve and in addition the eye may not be well fixed if standard formalin fixative is used. An alternative approach is to bisect the head longitudinally after decalcification, embed on the cut surface so that longitudinal sections can be made through the eye (Ramos et al. 2011) (Figure 12.3). The eye may also be dissected out fresh at the time of necropsy by careful enucleation (Smith et al. 2002b; Escher and Schorderet 2011) or after fixation of the head. It is important that if the eye is dissected fresh at the time of necropsy that excessive traction is not placed on the optic nerve as this may cause extensive artefact. The eye can be fixed at this stage by immersion in an appropriate fixative or further dissection can be undertaken if specific tissues are required for additional analysis, for example RNA extraction (Escher and Schorderet 2011). While fixation in Davidson’s fixative (Latendresse et al. 2002) is advocated to optimize retinal morphology, in practice the small size of the mouse eye means that adequate fixation, at least for primary morphological phenotyping screens, may be achieved using routine neutral buffered formalin or paraformaldehyde (Dubielzig et al. 2010, Ch. 1). There is no need to inject formalin into the globe or open the eye to allow penetration of fixation.
Figure 12.2 (a) and (b) Cross section through head and eye. Microscope image of early postnatal mouse.

Figure 12.3 (a) and (b) Longitudinal section through head can be used to sample inner ear and eye. Sections without artefact may be hard to achieve in animals older than about 14 days post natal.

If dissected carefully, a length of optic nerve may be left attached to the globe and can be sectioned along with the rest of the eye if carefully embedded. Alternatively the optic nerve may be dissected at the time of necropsy and embedded separately (Smith et al. 2002b). Similarly the Harderian gland may be dissected separately or blocked in association with the globe.
Ideally the eye should be sectioned in a vertical longitudinal plane (Moraweitz et al. 2004) through the optic nerve. If precise orientation of the eye is required then it is advisable to mark the temporal, nasal, dorsal or ventral points of the globe at the time of dissection using a suitable indelible dye or tattoo ink (Figure 12.4). The distribution of cone photoreceptor cells varies between the dorsal and ventral aspects of the mouse retina (Szel et al. 1992) and so it may be necessary to orientate the eye when embedding to ensure that both aspects of the retina are examined (Figure 12.5). In general, haematoxylin and eosin stain is sufficient for examination of eye histology. However periodic acid –Schiff (PAS)/Alcian Blue may be useful to demonstrate basement membranes, trichrome stains to show connective tissue and Luxol fast blue/cresyl violet stain may be useful to demonstrate myelinated fibres in the optic nerve (Dubielzig et al. 2010, Ch. 1) (Figure 12.6). If necessary, techniques can be used to bleach out melanin in the retinal pigment epithelium (RPE) to allow cellular morphology to be examined in more detail.
Figure 12.4 Eye can be marked with indelible ink by gently protruding the eye by applying pressure to draw back the eyelids. The eye should be gently dried by dabbing with tissue before repeatedly applying indelible ink to produce a clear spot.

Figure 12.5 Diagram to show plane of section (dotted line) to ensure all quadrants of retina examined from the right eye marked as shown in Figure 12.4. To embed the right eye the tissue should be placed in the embedding mould with the optic nerve (open circle) to the left and the dot • facing directly away from the histologist and held with the optic nerve and dot parallel to the mould base. The eye should be rolled down so that the dot is at 45° to the horizontal and held in this position until the wax has cooled. The paraffin block obtained should be trimmed until the optic nerve is visible and sections taken from this level. The same principle can be applied to the left eye if required. D = dorsal, V = ventral, N = nasal, T = temporal.

Figure 12.6 Structures in the eye can be demonstrated using special stains, in this case PAS positive Descemet’s membrane (thin arrow) and lens capsule (thick arrow).

12.3 Artefacts
The eye is prone to artefacts, which can occur due to handling of the eye in life, during dissection or during fixation and processing.
Although less commonly used now, in vivo periorbital bleeding techniques can result in traumatic damage to the structures associated with the eye leading to inflammation of the optic nerve, surrounding muscles and Harderian gland (Van Herck et al. 1992; Williams 2002; Heimann et al. 2009).
The eye is easily damaged during dissection and artefacts related to handling are common and may include obvious traumatic lesions and others that may be more subtle, for example, detachment of the retina. An artefactually detached retina can be distinguished from a pathological condition due to the absence of proteinaceous fluid, attachment of receptor process fragments to the retinal pigment epithelium (RPE) and lack of RPE hypertrophy (Figure 12.7). Detached retina and poor retinal morphology are also common unwanted features of formalin fixation. Crushing of the optic nerve during dissection can lead to artefactual displacement of myelin between the choroid and retina in the region adjacent to the nerve.
The eye is prone to multiple artefacts following fixation and processing for histology. Separation of the collagen lamellae in the cornea is commonly seen (Figure 12.8) and can be particularly marked in eyes stored in modified Davidson’s fixative (Latendresse et al. 2002). The lens is a relatively hard tissue compared to the other structures of the eye, which makes it difficult to section. As a result of this and fixation, a range of artefacts including separation of the capsule, cracking and cavitation of the body of the lens and the appearance of granular material can occur, which all need to be distinguished from the cataract formation that is common in ageing mice (Figure 12.9). Retinal folds and rosettes are common background changes but may also be caused by rough handling during processing (Figure 12.10). In addition without careful orientation at embedding oblique sections can lead to apparent thickening or thinning of various structures (Smith et al. 2002b).
Figure 12.8 Separation of collagen lamellae in cornea of Davidson’s fixed eye. Also showing mitotic figure in basal layer of corneal epithelium.

Figure 12.9 Cataracts can be confirmed by features such as swollen lens fibres (thick arrows) and disorganized nuclei (thin arrows).

Figure 12.10 Rosettes and folds in the retina may be seen as incidental findings and can be due to inappropriate handling of tissue.

12.4 Anatomy and histology of the eye and associated glands
The eye is a complex structure, which is contained within a tough connective tissue capsule called the sclera. The integrity of the sclera is interrupted cranially by the transparent cornea and caudally by the optic nerve. The sclera is joined at the limbus by the palpebral conjunctiva of the eyelid (Figure 12.11).
The sclera is lined by the uvea, which consists of the vascular elements of the eye—the iris, ciliary body and choroid—which is in turn lined by the retina. The lens is suspended between zonules linked to the ciliary body.
The inner eye can also be divided anatomically into three major compartments. The most cranial (anterior) is the anterior compartment, which lies between the inner surface of the cornea and the cranial surface of the iris. The posterior compartment lies between the iris and the lens. The vitreous compartment which lies between the lens and retina (Figure 12.12).
It should be noted that unilateral micropthalmia is relatively common in C57Bl/6 stains, more frequently affecting females than males and the right eye more commonly than the left (Smith et al. 1994).
12.4.1 Eyelids
The eyelids of mice are not routinely examined as part of most sampling protocols. It is, however, important to be aware of their morphology in case fragments of tissue appear on a slide or in the event of a phenotype affecting them. On the outer surface the eyelids are covered by haired skin comprising keratinized stratified squamous epithelium composed of 7–12 layers of keratinocytes and hair follicles and associated structures similar to those found elsewhere. Just before the mucocutaneous junction there is a row of cilia or eyelashes, which, although apparently longer, are in other respects morphologically similar to pelage hairs (Tauchi et al. 2010). The branching stratified epithelial lined ducts of the Meibomian glands open on to the eyelid surface at the mucocutaneous junction (Figure 12.11 and 12.13). The Meibomian glands consist of lobules of sebaceous epithelium similar to the sebaceous glands of hair follicles. The eyelid stroma consists of a connective tissue containing a dense collagenous plate and striated muscle.
At the mucocutaneous junction, the epithelium abruptly changes to a thinner stratified but nonkeratinized epithelium, which makes up the palbebral conjunctiva. Goblet cells are also found with varying densities in this epithelium and can be visualized with Alcian blue/PAS stains. Around most of the globe the conjunctival epithelium folds back at the fornix to cover the sclera (bulbar conjunctiva). The palbebral conjuctival epithelium is thicker (4-7 cells) than the bulbar (2-4 cells). In the region of the inner canthus the conjunctiva also covers the nictatating membrane, a tissue fold which is given rigidity by a cartilage plate (Figure 12.13). Beneath the conjunctival epithelium is a connective tissue substantia propria containing blood vessels and in normal animals a resident population of mast cells, lymphocytes and plasma cells. Lymphoid aggregates may be found associated with the nictitating membrane in normal mice (Sakimoto 2002) and lymphoid follicles may occur elsewhere in the conjunctiva following antigen stimulation.
12.4.2 Sclera and cornea
Although the sclera in mice is structurally tough, it is sufficiently thin that the pigmented choroid may be seen through it giving the globe a dark appearance in pigmented strains. Microscopically, the sclera is composed of three connective tissue layers. The outer layer or episclera is composed of fibrovascular tissue and forms points of attachment for the ocular muscles. The episclera is lined by a dense collagenous stroma, which merges with a thin inner layer of collagen (lamina fusca) that loosely attaches the stroma to the underlying choroid. The stroma contains haphazardly arranged collagen fibres and occasional fibroblasts. Emissarial canals traverse the sclera and contain nerves and blood vessels.
The sclera joins the cornea abruptly at the limbus. The surface of the cornea is lined by a nonkeratinizing stratified squamous epithelium, which usually appears to be 4–7 cells thick with the light microscope (although up to 13 layers may be seen using electron microscopy Henriksson et al. 2009) (Figure 12.14) and is thicker centrally than at the periphery. The basal cells are cuboidal and, in normal animals, few, if any mitoses are present and these should be found in this layer only. Mitoses in higher layers suggest an increase in proliferation. The epithelium is attached to a basal lamina which can be stained with PAS (Figure 12.6). Subepithelial mineralization in the cornea is a frequent finding in ageing mice (Figure 12.15). Mineralization can be confirmed by using special stains such as Von Kossa’s.
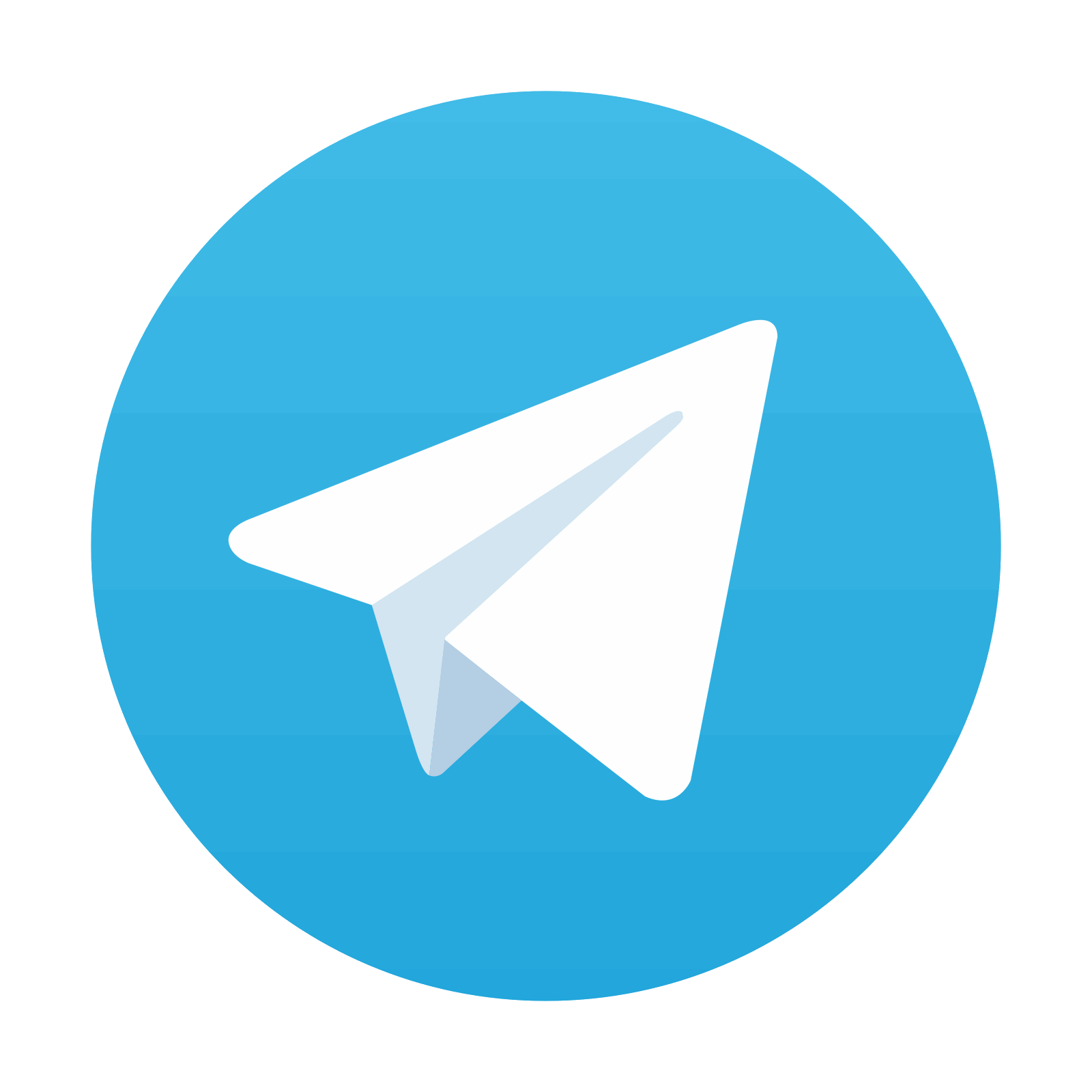
Stay updated, free articles. Join our Telegram channel

Full access? Get Clinical Tree
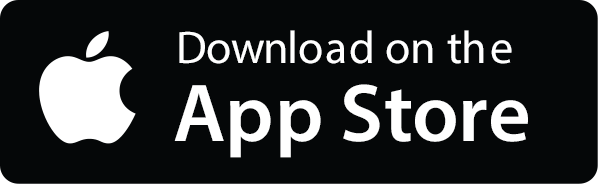
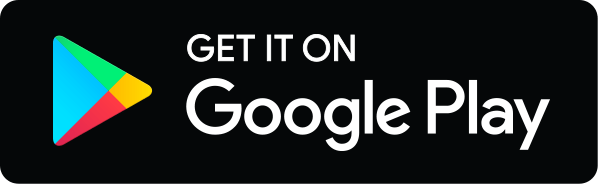