8.1 Introduction
The nervous system of the mouse is similar to that of other mammals in being divided anatomically and functionally into the central nervous system (CNS), consisting of the brain and spinal cord, and the peripheral nervous system (PNS), which connects the CNS to other organs and includes motor and sensory cranial and peripheral nerves and autonomic ganglia (controlling visceral functions).
With the widespread use of mice as biological models, an extensive literature has developed on the anatomy, physiology, development and pathology of its nervous system. Many resources are available online (see references to web sites at the end of this chapter).
8.2 Necropsy
The brain and spinal cord are lipid-rich, soft, fragile tissues, and therefore prone to microscopic and macroscopic artefacts. Prevention or limitation of artefacts during necropsy and subsequent tissue preparation is widely discussed in the literature because of the practical challenges in distinguishing between artefacts and pathological lesions. The principles used to reduce artefact are the same for all species and numerous general reviews of techniques are available that can be used in developing best practices for nervous tissue sampling in mice (Garman 2011; Spijker 2011; Bolon et al. 2013; Jordan et al. 2011).
Cervical dislocation can lead to extensive haemorrhage and traumatic damage especially to the hindbrain and cranial spinal cord and should, if possible, be avoided as a method of euthanasia when examination of the CNS is required. It is preferable to sever the head from the rest of the body after death with a sharp scalpel blade introduced at the level of the first cervical vertebra before dissecting the brain out of the skull (Figure 8.1). After this, although details in the necropsy techniques vary to some extent between laboratories, reflecting local experience and preference, examination of the CNS typically requires opening the bone in which the tissue is enclosed (Figure 8.1, Chapter 1, Figure 1.50). Once the head is severed from the body, the easiest way to remove the skull cap is to proceed cranially from the foramen magnum (the caudal opening in the skull from which the spinal cord protrudes). How far cranially the skull cap is removed will determine whether the olfactory bulbs or the nasal cavities are preserved for macroscopic and microscopic examination as it can be difficult to preserve both optimally. For this reason, it is worth considering ahead of time if the examination of the olfactory bulbs, which are an important region, rich in neural progenitors, will be required. Variable sampling of this region is also a source of inter-animal variation when brain weights are collected.
Figure 8.1 Necropsy. A dorsal longitudinal section (line in (a)) is carried with a scalpel blade from the nose down to the neck. Dorsal and lateral skin, subcutaneous tissues and muscles are removed to below the eyes and ears level (line in (b) and (c)). The skull is then severed from the rest of the body at the level of the first cervical vertebra with a sharp scalpel (to avoid crushing the enclosed cord). At this stage, the brain can be seen through the skull cap (i.e. the calvarium) (d) which can then be removed (e). For this, fine scissors are inserted into the foramen magnum and the skull cap is progressively lifted with fine forceps. The position of the pineal gland (which may remain attached on the skull cap) is indicated (asterisk in (e)). After removing the skull cap, and to avoid damaging the brain when lifting it out of the skull, care should be taken in preliminarily removing the cerebellar tentorium, transversally separating the cerebral cortex from the cerebellum and the cerebral falx, longitudinally separating both cerebral hemispheres. Once the skull cap and meninges are removed, the brain can be immersion fixed in situ or dissected further out of the skull. In this latter case, freeing the cranial end of the brain requires a sharp full thickness transversal cut through the olfactory bulbs (if their examination is not required) or further cranially (if the nasal cavities are not being preserved) removing sufficient bone on each sides to ensure that the brain (with or without remaining olfactory bulbs) can easily be lifted out of the skull without being ripped (dorsal view (f) and lateral view (g)). Finally, the ventral cranial nerves and pituitary are carefully sectioned to free the brain from the base of the skull (h). The pituitary (* in (i)) which is located in a small recess (the sella), can be separately fixed in situ with the remaining ventral skull (i). Likewise, the trigeminal ganglia (arrows), which sit on the ventral surface of the skull with its afferent nerves running cranially and efferent roots caudally, can be fixed or further isolated by freeing them from the thick covering dura.

Of the three layers of meninges separating the skull from the brain (or the vertebral body from the spinal cord) the dura mater is the most external and the toughest. Going inwards, are the richly vascularized pia arachnoid and the pia mater. Individual layers are not readily distinguishable at necropsy, but the meninges need to be removed before the brain can be extracted from the skull (Spijker 2011). This is best done by grasping them with a pair of forceps at the level of their two fibrous extensions, the cerebellar tentorium and the cerebral falx (Figure 8.1). Once removed, the naturally smooth surface of the brain is revealed (the mouse is a lissencephalic species lacking the gyri and sulci seen in the brain of gyrencephalic species) (Figure 8.1). The skull, containing the brain, can then be tilted upside down and the cranial nerves and pituitary cut ventrally while preventing pulling and stretching of the tissue as it is freed from the bone (Figure 8.1). Once the brain is removed, the floor of skull will still contain the pituitary in its sella, together with the trigeminal ganglia and nerves (Figure 8.1). The pineal gland that sits dorsally at the junction between the cerebral hemispheres and the cerebellum can be left attached to the skull cap.
The vertebral column, containing the spinal cord, is revealed by sectioning the skin along the back of the animal and removing the dorsal muscles on each side. The vertebral column can then be separated from the rest of the body by cutting along the proximal end of the ribs and the base of the sacrum. From there, the column can be either partially opened (laminectomy) or processed with the cord in situ (see section 8.3).
Routine examination of the PNS in mice is usually restricted to examination of a unilateral section of sciatic nerve (peripheral nerve). The sciatic nerves run along the lateral-caudal side of the femur and are easily accessed dorsally by sectioning the thigh muscles along the sacrum and spreading them laterally (Figure 8.2).
Figure 8.2 Sciatic nerve necropsy. A dorsal longitudinal dissection has been performed with a scalpel blade along the midline revealing the vertebral column. The dorsal muscular bundles (black asterisk) are severed from the column and pelvis with a scalpel to expose the white sciatic nerve (arrows) on each side.

At this stage, it is helpful to preserve the proximal / distal orientation of the spinal cord and nerves. This can be achieved by cutting the proximal and distal end of the cord / nerve at a different angle (straight cut at one end, oblique at the other), by tying a fine thread of material at one end of the sample (Krinke et al. 2000b) or by adhering the sample to a cardboard, the orientation being labelled with a pencil (ink can be washed out by solvents). Preserving the samples from stretch or crush can be achieved by manipulating them with care, holding them by their extremities, by using sharp blade or scissors for sectioning them and by laying them flat on a piece of cardboard before dropping them into the fixative. Techniques for preparing peripheral nerves for immersion fixation have been well described elsewhere (Jortner 2011).
Sampling of other nerves (cranial or peripheral) and ganglia is generally restricted to studies where they are of specific interest and are not described in detail here but protocols can be found in the literature (Shimeld et al. 2001; Bolon et al. 2006 and 2008).
Macroscopic observations visible at necropsy may be lost once the tissues have been further dissected or fixed and so it is important that these are described at necropsy (see Chapter 2, Table 2.3 for example descriptors). Here, an awareness of the visible anatomical landmarks helps to distinguish subtle changes (Figure 8.3). For instance, a proportional decrease in the size of the cerebellum, when compared with the cerebral cortex and medulla oblongata adjacent to it, can be used to diagnose cerebellar atrophy.
Figure 8.3 Macroscopic observation of the mouse brain. (a) On the dorsal surface of the brain (from rostral to caudal) one can recognize the position of the two olfactory bulbs (OB) and smooth cerebral hemispheres of the cerebral cortex which divide into distinct regions: the frontal (Fc), parietal (Pc), occipital (Oc), temporal (Te), cingulate (Cg), retrosplenial (Rs) and, on the ventral surface, the piriform (Pir) cortex. Also on the dorsal surface of the brain are the emplacement of the pineal gland (black asterisk) left attached on the skull cap, the superior and inferior colliculi -respectively referred to as anterior or rostral (Col r) and posterior or caudal (Col c) when respecting the spatial orientation in a quadruped mouse, the cerebellum (CB)-divided into a central vermis and two lateral hemispheres and extending at its widest part into the paraflocculi- and the medulla oblongata (MO) beneath it. (b) Rostro-caudal examination of the ventral surface of the mouse brain shows again the olfactory bulbs, the cortical hemispheres on which may sit the two optic nerves converging caudally into the optic chiasm (ox), the ventral surface of the hypothalamus which surrounds the infundibulum (white asterisk) where the pituitary attaches to the brain, the mamillary bodies (Mb), cerebral peduncles (cp) and the pons (PON) in continuity with the medulla oblongata along which run the pyramids (pyr). (c) These ventral macroscopic landmarks can be used to select the transversal sections prepared for histology (the black lines indicate approximate positions of the transversal sections presented on Figure 8.11).

Neuroanatomical terminology used in describing the CNS can be confusing, as some is based on the macroscopic landmarks, the developmental origin or on its functional units. Confusion and overlap may inevitably occur, which are also the reflection of our evolving knowledge of the CNS, of its functions and of the comparative similarity (or difference) between the mouse and other species. (For a comparative review of mouse and human brains the reader is referred to Hagan et al. (2011)). There is also potential for confusion over some commonly used terms. “Brainstem”, for instance, can be used to name the pons and medulla oblongata, but sometimes additionally includes parts of the midbrain and diencephalon. For descriptive purposes, lesions can be located using anatomical landmarks (Figure 8.3) complemented with qualifiers (for example, red focus on the cranioventral surface of the left olfactory bulb). Familiarity with these descriptive landmarks is therefore helpful to locate the changes observed (and their possible relationship to clinical signs). Being consistent and specific to be understood by others and allow data comparison across observers and/or studies is also valuable. It may not always be necessary to link the location of a lesion to an individual nucleus but using ‘brain’ as a lesion locator can be insufficient. In practice, a template of useful locators for recording macroscopic and microscopic lesions (either as a tabulated list of structures to examine or as a diagram) prior to starting the work can be time well spent (Figure 8.3 and Table 8.1).
Table 8.1 Example of template for recording macroscopic and microscopic lesions in the mouse brain. Adapted from Bolon et al. 2013. The structures listed here can be identified on figures 8.3, 8.8, 8.11 and/or 8.12.


8.3 Fixation/perfusion
The susceptibility of the nervous tissue to artefact also impact on its fixation. Historical habit and the prominence of specialist neuropathology techniques in the literature have led to the frequent recommendation to use perfusion fixation for CNS and/or PNS examination.
Perfusion fixation prior to tissue handling has advantages because, providing it is correctly done, it minimizes the time between death and tissue fixation. The fixative is directly infused through the vasculature antemortem under deep anaesthesia, minimizing autolysis and some of the perimortem artefacts induced by hypoxia, tissue handling or processing (mainly dark neurons and vacuolation). Perivascular retraction of the neuropil, which can be seen in immersion fixed tissues and may be worsened with hyperosmolar fixatives (Fix and Garman 2000), is another artefact not seen in well-perfused fixed tissues, unless too great a pressure is applied in the perfusion system. Perfusion fixation can also reveal subtle microscopic changes, which are not readily visible in immersion fixed tissues (Auer and Coulter 1994).
However, it has disadvantages in that it is time-consuming, potentially hazardous for the operator (inhalation of fixative fumes) and also limits the flexibility of using different fixatives for different tissues from the same animal at the time of necropsy. It also requires additional equipment, careful planning, proficiency of the operator in the technique and familiarity for the pathologist subsequently examining the tissue microscopically (as the tissue morphology will differ slightly from that of immersion fixed tissue: for example blood vessels will appear patent and empty) (Figure 8.4). Additionally, suboptimal perfusion may not deliver the expected advantages over immersion fixation and may increase interanimal variability in tissue morphology, which may in turn compromise the comparative microscopic examination. For these reasons and given that an experienced pathologist can, in the majority of cases, differentiate pathological lesions from those artefactual changes present in immersion fixed tissue, this simpler technique is considered acceptable when examination of the nervous tissue forms part of a routine screen of all organs rather than a specific study of the nervous system (Butt 2011).
Figure 8.4 Perfusion or immersion fixations and artefacts. (a) Blood vessels are patent and devoid of red blood cells in perfused nervous tissue (a section of cerebral cortex). (b) In contrast in immersion fixed tissue (in a comparable section of cerebral cortex), blood vessels are collapsed and/or contain red blood cells (arrows). (c) Artefactual vacuolation (blue arrows) can be seen in immersion fixed tissue, often located in the deep cerebellar nuclei/peduncles region. These vacuoles are variably sized, often empty with smooth rounded edges. The adjacent neurons appear morphologically normal and the cellularity of the area is unremarkable (indicating the absence of any glial reaction associated with the presence of the vacuoles). Perivascular retraction of the neuropil (an artefact of immersion fixation) can also be seen here (thick arrow). (d) Excessive pressure applied in a perfusion system can lead to severe artefactual vacuolation of the tissue giving it a ‘Swiss cheese’ appearance, not unlike that of severe freezing artefact.

Although 4% PFA is often chosen in the field of neurosciences for IHC and specialized neuropathological examination on the basis that alcohol contained in ready-made NBF solutions (and in other alcohol based fixatives) can cause artefactual vacuolation (Figure 8.4), 10% NBF may be suitable for many routine examinations. Another commonly used fixative in neurosciences is glutaraldehyde (either alone at 4% or in combination with PFA), which provides optimal fixation for EM. Specific examination of myelin sheaths by electron microscopy is a good example of when the combined use of glutaraldehyde and perfusion are desirable for optimal preservation of morphology (poorly fixed myelin sheath show artefactual separation –from the axon and / or between consecutive myelin layers—vacuolation and loss of cylindrical shape). Osmium fixation or postfixation is also used in some protocols. This provides increased contrast suitable for EM examination, but is not necessary for routine H&E pathological examination.
Freeze fixation, alone or in combination with preliminary perfusion, is best done by immersion of the tissue in cooled isopentane (sometimes preceded by immersion in a hypertonic sucrose solution) rather than by direct contact with dry ice or liquid nitrogen to protect cell membranes and tissue morphology. Such protocols, suitable for preserving genetic material (for in situ hybridization or PCR) and / or some proteins (for the IHC of some antigens), are not recommended for morphological examination of FFPE H&E sections, as immersion in hypertonic solutions and freezing induce tissue shrinkage and freeze artefacts.
The spinal cord can be fixed and processed in situ with the vertebrae (Figure 8.5). However, decalcification of the bone (to soften the tissue so that it can be cut on the microtome) prior to tissue processing is often done using formic acid. This has a negative impact on morphology (Knoblaugh et al. 2011) and results in shrunken cell nuclei with poor cytoplasmic and nuclear details (Figure 8.5). Partial dissection (laminectomy of a small segment of the column) or complete dissection of the spinal cord from the vertebral column prior to fixation can improve morphology but this has to be weighed against the time it takes , the delay in the time to fixation and the potential secondary artefacts (autolysis, crush, stretch etc.). Complete dissection of the cord from the vertebrae may also result in loss of the dorsal root ganglia (DRGs). For these reasons, perfusion fixation may be required when optimal morphological preservation of the cord, ganglia or nerves is needed for detailed and specific microscopic examination.
Figure 8.5 Spinal cord fixation, artefacts and histology. The spinal cord can be fixed and processed in situ (a, (c), (d) and (e)) with the vertebral column (after immersion in decalcification fixative) or fixed in NBF and isolated from the vertebral column prior to processing (b). The former may lead to artefactual changes. These include retraction of the cord in the vertebral canal (a), which can also be appreciated from the wrinkled surface of the cord and dark neurons (visible in (a), (c), (d), (e) and in insert in (C)). The illustrations in (c), (d) and (e) also provide a comparison of the relative size and shape of the central grey matter and dorsal horns in the cervical (c), thoracic (d) and lumbar (e) spinal cord. In cross section, the cervical cord is flatted dorsoventrally, the thoracic cord is more circular and the lumbar cord more trapezoid with proportionally thinner white matter and increasingly large surrounding nerve bundles as one progresses caudally. (Note: the scale varies in these different images)

8.4 Trimming
The value of careful examination of macroscopic landmarks on the surface of the brain and of their relative size and positions is mentioned in section 8.2, with alteration in size, colour, shape and relative positions potentially representing part of a phenotype (congenital/developmental or acquired). These landmarks can also be used to assist the sampling / slicing of the brain (Radovsky and Mahler 1999; Spijker 2011). Sectioning reveals new structures, which can also be examined for the presence of abnormalities, before the tissue is processed.
A brain atlas (hard copy, electronic or web-based versions, see references) can be useful to learn and identify anatomical structures (Paxinos and Franklin 2012). Familiarity with the neuroanatomy of the spinal cord, for which reviews and atlases are available, can also help integrating morphological lesions with clinical signs (Bolon 2000).
Most brain atlases provide stereotactic coordinates to reference the position of each brain (or spinal cord) structure on a 3D grid. These coordinates are used for targeted neurosurgery using a stereotactic frame fixed on the animal’s head (or back). These atlases use a reference point called bregma, identified by the intersection of the coronal and sagittal sutures on the skull at the junction between frontal and parietal bones. As an alternative to macroscopic landmarks, brain trimming can also be done using a matrix (also known as a brain slicer). These matrices (Figure 8.6) are available for a range of ages and sizes of mouse brain, to produce standard sections of a range of thicknesses and orientations. They potentially allow more consistent sectioning between animals and the production of sections matching that of an atlas (symmetrical along the median and horizontal axis, which makes the comparison of the microscopic section to that of the atlas easier). In practice, the advantages need to be balanced against the cost of these matrices and the degree of variability that still can be introduced depending on how perfectly the brain is positioned in the mould and the proficiency of the technician. In particular, how far caudally the medulla is cut and whether the olfactory bulbs are present will influence the position of the brain in the mould.
Trimming guidelines using macroscopic landmarks or stereotactic coordinates are available to assist developing a trimming scheme for the mouse brain. Most are derived from the field of toxicological pathology (Radovsky and Mahler 1999; Morawietz et al. 2004; Jordan et al. 2011; Bolon et al. 2013). Although often not specifically designed for mice (describing general procedures for rodents or specific to rats), the principles and types of sections they describe are relevant. What represents an adequate sample of nervous tissue (and of brain in particular) for routine microscopic evaluation is the subject of debate (Rao et al. 2011; Switzer et al. 2011; Bolon et al. 2013) centred on which anatomical and/or functional regions need to be examined given the relative complexity (anatomically and functionally) of the nervous system, the discrepancy that may occur between clinical observations and microscopic morphological lesions and the selective sensitivity of (sometimes small) regions. In practice a good scheme, whether designed for toxicology, phenotyping or fundamental research will cover a selection of anatomical/functional regions, be easily reproducible and consistent across the number of animals in the study and may also depend on the study focus.
Most trimming schemes used by pathologists consist of coronal sections which are easily compared with atlas plates (Morawietz et al. 2004; Jordan et al. 2011; Switzer et al. 2011; Bolon et al. 2013) (Figure 8.3 and Table 8.1). Sagittal/ parasagittal sections can be used to examine specific structures but can be difficult to obtain with consistent orientations because of their length.
Once the brain has been sliced, it is good practice to lay the different samples produced on the working surface prior to embedding the tissue in their cassettes and ensure that all are present and that the cranio-caudal orientation of the embedded surface is known. Microscopic sections are only few microns thick and as most trimming schemes will be limited to less than ten sections, flipping one of the trimmed fragment will result in examining the same section twice instead of two different sections a few millimetres apart. When trying to correlate clinical signs with any potential unilateral histological lesion, the preservation of the left/right orientation is also essential and would also be lost if the segments are inadvertently flipped at the time of embedding.
In the absence of visible macroscopic change or when carrying a routine sampling scheme for microscopic examination of the spinal cord, one to three segments of cord (from the cervical, thoracic and lumbar region) are considered sufficient. Transverse sections of cord provide a cross-section of nerves fascicules while longitudinal sections allow nerves to be followed and facilitate the identification of lesions. On a transverse section, the shape of the central grey matter makes it possible to recognize cervical, thoracic and lumbar areas (Figure 8.5) (Radovsky and Mahler 1999). A comprehensive scheme can include both transverse and longitudinal sections. The cervical intumescences, brachial plexus and nerves extending from the cord in the sacral region are not routinely examined. Labelling the tissue to preserve the craniocaudal and therefore left/right orientation is important to locate the changes (cervical, thoracic, lumbar, left or right, ventral or dorsal) and, if relevant, associate them with the relevant clinical signs. This can be done by having a small incision, a suture or an ink spot placed on one side, which would be recognizable on the histological section.
Dorsal root ganglia can be readily examined when the spinal cord has been fixed in situ in the vertebral canal (Figure 8.5). Serial parasagittal or horizontal sections can also be prepared to maximize the chance for DRGs to be included. Alternatively they can be sampled from the cord at necropsy and embedded in a cassette in groups of representative cervical, thoracic and lumbar ganglia (Bolon et al. 2013).
8.5 Special stains and techniques
The landmarks visible on the trimmed sections and additional ones can readily be distinguished on H&E-stained sections. Brain atlases often show Nissl’s stain (Cresyl violet) sections (instead of H&E sections), which distinguishes white and grey matter clearly (Figure 8.7). Nissl’s stain or toluidine blue stained sections can be used when more detailed structures needs to be distinguished, as discrete grey matter nuclei and white matter tracts can be more readily identified and, from their respective locations, precise position can be inferred with the help of an atlas. However the examination of H&E sections provides sufficient detail for identifying a number of structures and to locate the occurrence of potential pathological changes. Also it offers better cell morphology for the characterization of these lesions. Routine H&E sections should be 3–5 um thick as thicker sections sometimes used in research settings can give the tissue a hypercellular appearance, which can be confusing on histological examination (Figure 8.8).
Figure 8.7 Parasagittal section of Nissl’s stained mouse brain. Nissl’s stain (Cresyl violet) detects neurons by staining purple-blue the Nissl’s substance (RER) they contain in their cytoplasm. This therefore provides a good contrast between highly stained grey matter and paler, more poorly stained white matter. On this section the olfactory bulbs (OB), cerebral cortex (COR), striatum (ST), hippocampus (HIP), midbrain (MID), pons (PON), cerebellum (CB) and medulla oblongata (MO) regions can readily be identified.

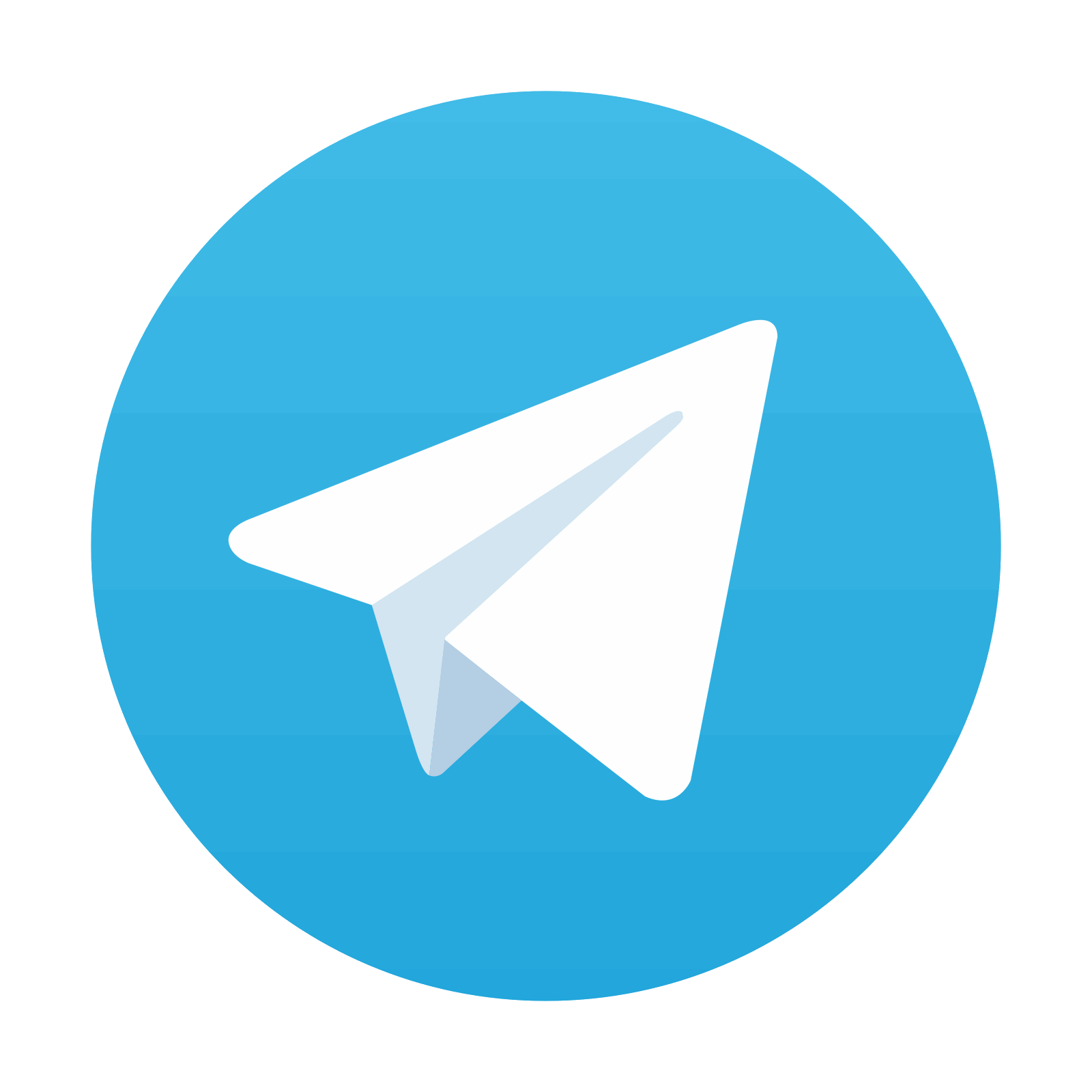
Stay updated, free articles. Join our Telegram channel

Full access? Get Clinical Tree
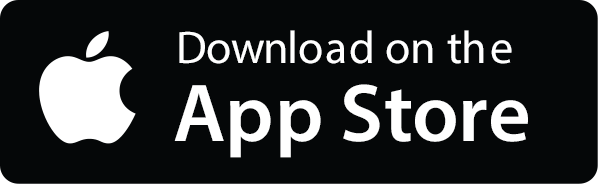
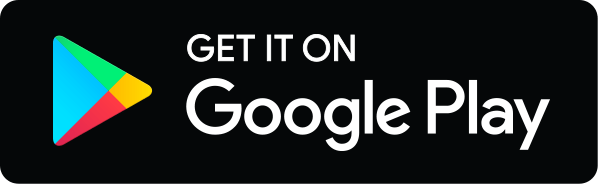