Chapter 74 Snake Bite: North American Pit Vipers
SOURCES
Pit vipers are the largest group of venomous snakes in the United States and are involved in an estimated 150,000 bites annually of dogs and cats.1 Approximately 99% of all venomous snake bites in the United States are inflicted by pit vipers. In North America, members of the family Crotalidae belong to three genera: the rattlesnakes (Crotalus and Sistrurus spp.) and the copperheads and cottonmouth water moccasins (Agkistrodon spp.).
Pit vipers can be identified by their characteristic retractable front fangs, bilateral heat-sensing “pits” between the nostrils and eyes, elliptical pupils, a single row of subcaudal scales distal to the anal plate, and triangular shaped heads (Figure 74-1). Those members of the Crotalus and Sistrurus genera (the rattlesnakes) have special keratin rattles on the ends of their tails, with the exception of one subspecies (C. catalinensis). Agkistrodon species, the copperheads and water moccasins, are found throughout the Eastern and Central United States. Copperheads are responsible for the majority of venomous snake bites to humans in North America because of their proclivity for living next to human habitation. Water moccasins can be pugnacious and have a greater tendency to deliver venom when they bite. Rattlesnakes (Crotalus spp, Sistrurus spp) are found throughout the continental United States and account for the majority of deaths in both human and animal victims. Clinicians should become familiar with their regional indigenous poisonous snake species.
Ninety percent of venomous snake bites occur during the months of April through October. Two thirds of the bites are inflicted by snakes less than 20 inches in length. The heat-sensing pit located between the eye and the nostril can differentiate a temperature gradient of 0.003° C at a distance of 14 inches. These snakes can strike approximately one half of their body length at a speed of 8 ft/sec. The number of puncture wounds from a single bite can be one to six because several ancillary fangs can rotate forward with the strike. Rattlesnakes do not always rattle before striking.
Based on clinical observations, it was once thought that pit viper venom was more toxic in the hotter months of the year. However, when individual snake venoms were analyzed, no seasonal variation in patterns of venom proteins was evident over any period of time. The venom is not considered more toxic in the summer months; however, snakes show increased aggression and venom yield with environmental warming and an increased photoperiod (as in the spring and summer).2 The maximum venom yields occur during the hottest months of summer.
TOXIC DOSE
The severity of any pit viper bite is related to the volume and toxicity of the venom injected and to the location of the bite, which may influence the rate of venom uptake. As a generalization, the toxicity of pit viper venoms ranges in descending order from the rattlesnakes to the water moccasins and then to the copperheads (Table 74-1). The toxicity of rattlesnake venom varies widely. Nine species and 12 subspecies of rattlesnakes have populations with venoms containing proteins that are immunologically similar to the potent neurotoxin, Mojave toxin. It is possible for pit viper venom to be strictly neurotoxic with virtually no local signs of envenomation. Examples of these venom types are certain subpopulations of rattlesnakes: Mojave rattlesnakes (C. scutulatus) canebrake rattlesnakes (C. horridus atricaudatus), and tiger rattlesnakes (C. tigris).3–5
Table 74-1 Venom Yields of North American Snakes
Snake Species | Dry Weight (mg venom) | LD50 IV (mice) |
---|---|---|
Eastern diamondback (Crotalus adamanteus) | 200–850 | 1.68 |
Western diamondback (Crotalus atrox) | 175–800 | 2.18 |
Mojave rattlesnake (Crotalus scutulatus) | 75–150 | 0.23 |
Eastern coral (Micrurus fulvius) | 2–20 | 0.28 |
Copperhead (Agkistrodon contortrix) | 40–75 | 10.92 |
Cottonmouth (Agkistrodon piscivorus) | 90–170 | 4.19 |
MECHANISM OF TOXICITY
Pit viper venoms are a complex combination of enzymatic and nonenzymatic proteins (Box 74-1). The primary purpose of the venom is not to kill, but rather to immobilize the prey and predigest its tissue. The venom is derived from modified salivary glands. The venom is 90% water and has a minimum of 10 enzymes and 3 to 12 nonenzymatic proteins and peptides in any individual snake. The nonenzymatic components, called the “killing fraction,” have a median lethal dose (LD50) more than 50 times smaller than that of the crude venom.
Box 74-1 Examples of Enzymes from Pit Viper Venoms
Arginine ester hydrolase | Phosphodiesterase |
Proteolytic enzymes | Acetylcholinesterase |
Thrombin-like enzyme | RNAse |
Collagenase | DNAse |
Hyaluronidase | 5’-Nucleotidase |
Phospholipase A2 | NAD-nucleotidase |
Phospholipase B | Lactate dehydrogenase |
Phosphomonoesterase | L-Amino acid oxidase |
More than 60 purified polypeptides have been identified in crotalid venoms. Approximately 50 enzymatic crotalid venom fractions have been characterized. Proteolytic trypsin-like enzymes, which are catalyzed by metals (e.g., calcium, magnesium, and zinc), are common constituents of pit viper venom and cause marked tissue destruction. Arginine ester hydrolase is a bradykinin-releasing agent that may adversely affect clotting activity. Thrombin-like enzymes also can mediate increased clotting activity. The eastern diamondback rattlesnake (C. adamanteus) venom enzyme, protease H, induces systemic hemorrhage.6 Five proteolytic toxins from western diamondback rattlesnake (C. atrox) venom induce hemorrhage by cleaving laminin and the basement membrane at band A.7,8 Crotavirin, found in prairie rattlesnake (C. viridis viridis) venom, is a potent platelet aggregation inhibitor and prevents platelet-collagen interaction by binding to collagen fibers. Interference with the platelet-collagen interaction has the net effect of blocking collagen-mediated platelet functions, such as adhesion, release reaction, thromboxane formation, and aggregation.9 The preponderant mechanism of afibrinogenemia seen in a patient after western diamondback rattlesnake (C. atrox) envenomation is a reflection of fibrinogenolysis and not a primary consumptive coagulopathy. The fibrinogenolysis results from indirect activation of plasminogen by vascular plasminogen activator.10
Differences in venom within a species induced by the age of the snake are highlighted by a study of northern Pacific rattlesnakes (Crotalus viridis organus) in which the adult venoms were shown to have approximately fivefold higher fibrinogenolytic protease activity. Two protease bands were identified in juvenile and subadult snakes, and four bands were identified in adult venom using gel filtration.11 Zinc metalloproteinase with fibrinolytic activity has been isolated from the venom of copperheads (Agkistrodon contortrix) and is called fibrolase. A specific fibrolase cleavage site is in the alpha chain of fibrin. The complexity of the issue of variation of venom components is highlighted by the differences found in fibrinolysis and complement inactivation of venoms from different Blacktail rattlesnakes (Crotalus molossus molossus). In a study of 72 individual Blacktail rattlesnake venoms, the following conclusion was made: there were no venom differences as a function of geographic distribution; however, individual venom variability was significant enough to be identified as an important clinical reality.12
The enzyme, phospholipase A, is distributed throughout pit viper venoms. This enzyme catalyzes the hydrolysis of fatty ester linkages in diacyl phosphatides, which form lysophosphatides and release unsaturated and saturated fatty acids. There are many antigenically different isoenzymes. Some controversy exists about the extent of any neurotoxic effects that these isoenzymes may possess. Many cellular substances may be released by this enzyme, including histamine, kinins, slow-reacting substance, serotonin, and acetylcholine. The extent of the release of these physiologically active compounds most likely depends on the ability of phospholipase A to degrade membranes. The enzyme, phospholipase B, may also be present and is responsible for hydrolyzing lysophosphatides. The phosphodiesterases, such as diester phosphohydrolase, break free the 5-mononucleotide, thereby attacking DNA and RNA and derivatives of arabinose. L-Amino acid oxidase catalyzes oxidation of L-alpha-amino acids and L-alpha-hydroxy acids. This is the most active of the known amino acid oxidases and has been found in all pit viper venoms studied; it is responsible for the yellow color of the venom. Nicotinamide adenine dinucleotide (NAD)-nucleotidase is found in Agkistrodon but not Crotalus venom. The enzyme catalyzes hydrolysis of nicotinamide N-ribosidic linkages of NAD, forming adenosine diphosphate riboside and nicotinamide. Other enzymes that are possibly present in viper venom include RNAse, DNAse, 5′-nucleotidase, and lactate dehydrogenase. Direct cardiotoxic effects of venom proteins have been exhibited in some pit viper venoms, particularly the diamondback rattlesnakes.
CLINICAL SIGNS
The onset of clinical signs after a snake bite may be delayed for several hours (Box 74-2). In humans it is estimated that 20% of all pit viper bites are nonenvenomating (i.e., dry), with an additional 25% classified as mild envenomations. It is for this reason that so many antidotal treatments are championed, and it also emphasizes the necessity to rely on scientific evaluation for the various treatment modalities proposed.
Box 74-2 Common Signs of Envenomation
Pain | Sloughing tissue |
Swelling | Shock |
Ecchymosis | Puncture(s) |
Weakness | Nausea |
Every pit viper envenomation is different for many reasons (Box 74-3). The severity of an envenomation is additionally altered by factors, such as species of victim, body mass, location of bite, postbite excitability, and use of premedications (e.g., nonsteroidal antiinflammatory drugs in older dogs that may make the dog more susceptible to clotting defects). The snake affects the severity of the envenomation by species and size of snake, age of snake, motivation of snake, and degree of venom regeneration since last use.
Box 74-3 Variables Affecting the Severity of Envenomation
Victim | Snake |
Body mass | Species |
Bite location | Size |
Time to medical facility | Age of snake |
Type of first aid applied | Motivation for bite |
Concurrent medications (Nonsteroidal | Time since last venom use |
antiinflammatory drugs, etc.) | Time of year |
It is possible that a life-threatening envenomation may occur with no local clinical signs other than the puncture wounds themselves. This seems particularly true of those snake species with primary neurotoxic venoms (Box 74-4). Local tissue reactions to pit viper envenomation include puncture wounds, one to six from a single bite, which may be bleeding. Occasionally these fang wounds appear as small lacerations. Rarely, local swelling can obscure puncture wounds from small snakes. Rapid onset of pain may ensue with development of progressive edema. Ecchymosis and petechiation may become manifest. Tissue necrosis may occur, particularly in envenomations to areas without a significant subcutaneous tissue mass. The presence of fang marks does not indicate that envenomation has occurred, only that a bite has taken place. It must be reiterated that the severity of local signs does not necessarily reflect the severity of the systemic envenomation.
Box 74-4 Species of Rattlesnakes That Have PopulationsContaining Neurotoxin
Crotalus durissus terrificus var. cumanensis
Crotalus durissus terrificus (Brazil)
Crotalus horridus atricaudatus
Crotalus mitchellii mitchellii
Crotalus scutulatus scutulatus (venom A)
Severe hypotension results from pooling of blood within the shock organ of the species bitten (i.e., the hepatosplanchnic [dogs] or pulmonary [cats] vascular bed) and fluid loss from the vascular compartment secondary to severe peripheral swelling. This swelling can be great. A 2-cm increase in the circumference of a human’s swelling thigh can incorporate one third of the patient’s circulating fluid volume.13 Often this swelling is not edema, but rather caused by extravascularized blood, resulting from damage to blood vessel walls as a result of swelling and rupturing of the endothelial cells of the microvasculature, leaving large gaps in the vessel walls.
The victim’s clotting anomalies largely depend on the species of snake involved (Figure 74-2). Coagulopathies range from direct blockage or inactivation of various factors in the patient’s clotting cascade to the possible destruction of megakaryocytes in the circulating blood and bone marrow (Box 74-5). A coagulopathy, with prolonged clotting times, develops in approximately 60% of envenomated patients; by far the most common is hypofibrinogenemia. Venom induced thrombocytopenia occurs in approximately 30% of envenomations with an untreated nadir usually occurring between 72 and 96 hours. Some venom fractions inhibit platelet adhesion. Other pit viper venoms do not affect clotting per se, but rather destroy clots once they are formed by initiating aggressive fibrinolysis. Syndromes resembling diffuse intravascular coagulation (DIC) are possible with pit viper envenomations.
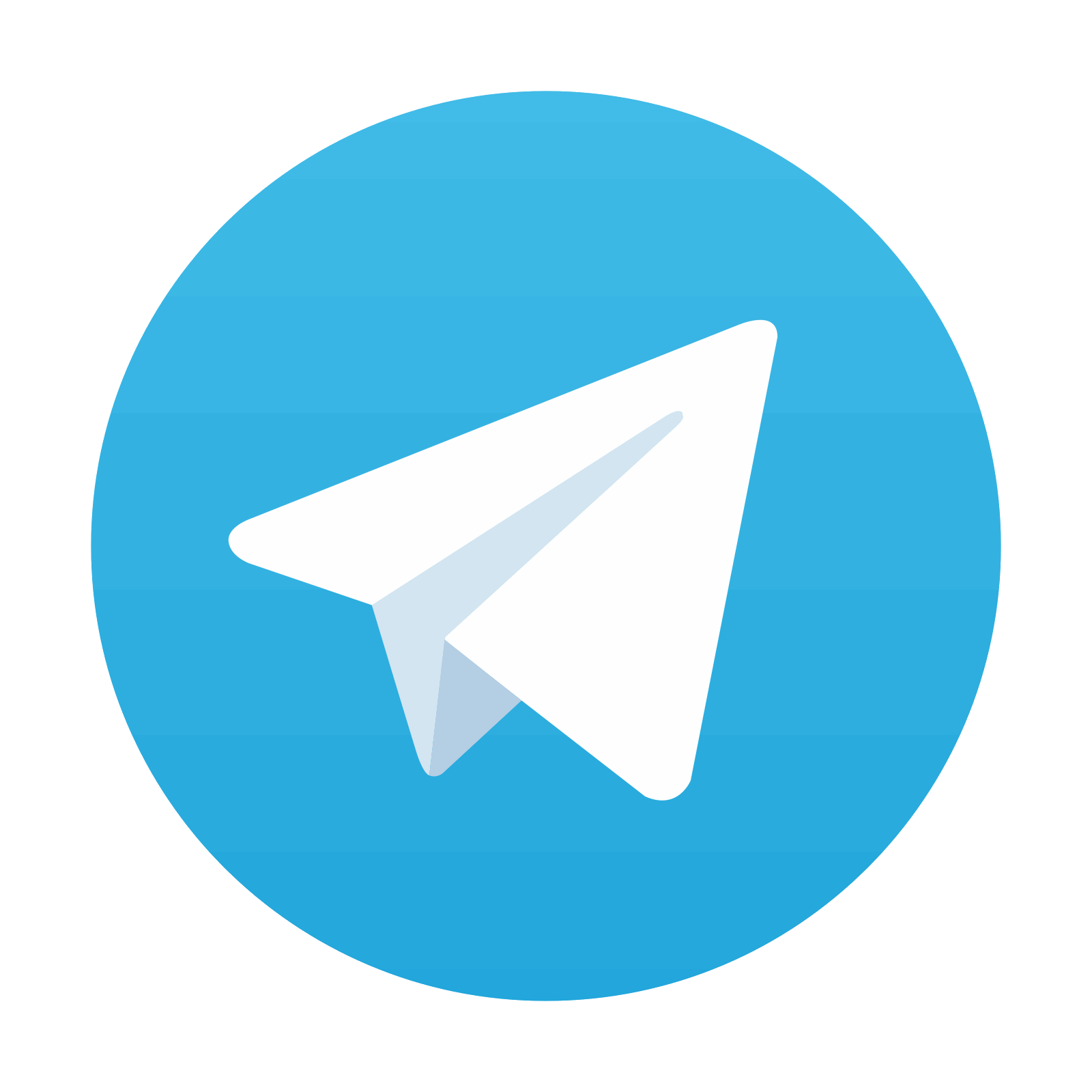
Stay updated, free articles. Join our Telegram channel

Full access? Get Clinical Tree
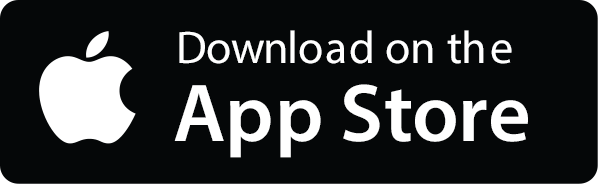
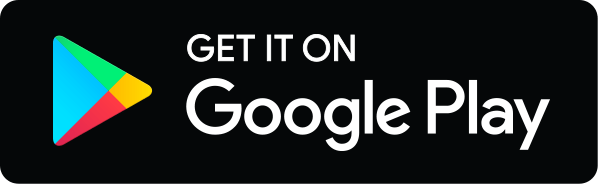