In the blood, oxygen is transported in two forms: dissolved in plasma (which is measured by PO2) and bound to hemoglobin (measured by SpO2). The amount of oxygen bound to hemoglobin is much larger than the amount dissolved in plasma (Fig. 1.2).
Figure 1.2. The amount of oxygen bound to hemoglobin is much larger than the amount dissolved in plasma. (Drawing by Melanie Tong.)

The “matching” of ventilation and perfusion is important to proper lung function. It does no good to ventilate an alveolus that is not being perfused (alveolar dead space) or to perfuse an alveolus that is not being ventilated because of atelectasis (collapse of alveoli). In the normal lung, ventilation (V) and perfusion (Q) are not evenly matched (known as V/Q mismatch), and this worsens with lung disease and dorsal or lateral recumbencies. Both ventilation and perfusion increase toward the dependent regions of the lung, but since blood is heavier than lung parenchyma, perfusion increases at a faster rate than ventilation. Vasodilation or vasoconstriction, caused by disease or anesthetic drugs, enhances V/Q mismatching and hypoxemia. From an anesthetic point of view, alveolar ventilation is very important because it will control the amount of volatile or gaseous anesthetic agent that can diffuse into the bloodstream. Any increase in alveolar ventilation will increase anesthetic uptake into the pulmonary blood (Fraser 2003).
The movement of air into and out of the lungs is called ventilation. The contraction of the inspiratory muscles, mainly the diaphragm, causes the chest cavity to expand, creating negative pressure. This is inspiration. During maximal inspiration, the diaphragm forces the abdominal contents ventrally and caudally. The external intercostal muscles are also involved. These muscles contract and raise the ribs during inspiration, increasing the diameter and volume of the chest cavity. Ventilation is the process by which gas in closed spaces is renewed or exchanged. As it applies to the lungs, it is a process of exchanging the gas in the airways and alveoli with gas from the environment. Breathing provides for ventilation and oxygenation.
Normal expiration is a passive process resulting from the natural recoil or elasticity of the expanded lung and chest wall. However, when breathing is rapid, the internal intercostal muscles and the abdominal muscles contract to help force air out of the lungs more fully and quickly. At the end of inspiration, the elasticity of the lung causes it to return to its smaller, unexpanded size. The ability to do this is called elastic recoil. The volume of air remaining in the lung at the end of a normal breath (the end expiratory lung volume) is called the functional residual capacity (FRC). FRC is composed of the expiratory reserve volume and the residual volume. On expiration, there is still air left in the lungs; if there weren’t, all of the alveoli would collapse. FRC decreases slightly in supine and lateral recumbency compared to prone. Certain conditions such as pregnancy, obesity, and abdominal distention due to gas-filled organs or masses can exacerbate decreases in FRC. FRC is diminished with small airway diseases. At FRC the alveoli in the nondependent lung sections are larger than those in the dependent regions because of gravity and the weight of the lung. Alveoli in the dependent regions are squashed and compressed by the weight of the overlying lung tissue. In lateral or dorsal positions the dependent alveoli are also compressed by the weight of the mediastinal structures and by the weight of the abdominal contents pressing against the diaphragm. In patients with large abdominal masses or excessive bloating (such as gastric dilatation volvulus GDV or obstructive colic in horses), the problem becomes especially significant.
In spite of many protective mechanisms in place in the lungs, including FRC, small airway and alveolar collapse (atelectasis) still occurs in the normal animal. Atelectasis is especially prominent in the dependent lung regions when an animal is recumbent. Intermittent deep positive pressure breaths in the anesthetized patient can help minimize small airway and alveolar collapse. The use of positive end expiratory pressure (PEEP) is known to help as well. PEEP increases airway pressure and FRC to help keep small airways and alveoli open during expiration (Battaglia 2001). PEEP valves are commercially available (in 5, 10, and 15 cm H2O) and can be added to the anesthesia machine for this purpose. Unfortunately, once atelectasis has occurred, it is very difficult to open the closed alveoli. Applying excessive pressure in an attempt to open alveoli (as in the case of attempting to reinflate a packed-off lung during a thoracotomy) tends only to damage the already working tissue (barotrauma) before reinflation takes place. Reinflation is a slow, delicate process and will happen on its own in healthy tissue over time or once recumbency or insult changes.
The degree of stiffness or compliance of the lung tissue affects the amount of pressure needed to increase or decrease the volume of the lung. With increasing stiffness, the lung becomes less able to return to its normal size during expiration. Virtually all diseases cause the compliance of the lungs to decrease to some extent.
The amount of airflow resistance can also affect lung volumes. Resistance is the degree of ease in which air can pass through the airways. It is determined by the number, length, and diameter of the airways. An animal with a high degree of resistance may not be able to exhale fully, thus some air becomes trapped in the lungs.
Tidal volume is the volume of gas passing into and out of the lungs in one normal respiratory cycle. Normal tidal volume for mammals is 10–20 mL/kg. Minute volume is used to describe the amount of gas moved per minute and is approximately 150–250 mL/kg/minute. Minute volume = tidal volume × respiratory rate. It is alveolar ventilation that is important for gas exchange, however. Alveolar ventilation is the portion of ventilation that contributes to gas exchange (McDonnell and Kerr 2007). Tidal volume is used to ventilate not only the alveoli, but also the airways leading to the alveoli. Because there is little or no diffusion of oxygen and carbon dioxide through the membranes of the airways, they comprise what is known as dead space ventilation (Fig. 1.3).
The other part of dead space is made up of alveoli with diminished capillary perfusion. Ventilating these alveoli is ineffective and will do nothing to improve blood gases. The nonperfused alveoli and the airways are known as anatomic dead spaces. Therefore tidal volume has a dead space component and an alveolar component. Dead space ventilation is about 30–40% of tidal volume and minute volume in a normal patient breathing a normal tidal volume. Dead space ventilation has a purpose. It assists in humidifying and tempering inhaled air, and it cools the body, as in panting. Panting is predominantly dead space ventilation. During panting, the respiratory frequency increases and the tidal volume decreases so that alveolar ventilation remains approximately constant. This is the reason that when animals under anesthesia pant, they very often wake up. They are not effectively ventilating their alveoli and exchanging gas well. Often times these patients will be hypercarbic because they are not able to effectively reduce their carbon dioxide levels. Slower, deeper breaths are usually more efficient. Certain pieces of anesthesia equipment can add to the anatomical dead space of a patient by “extending” its airway. This is called mechanical dead space. Endotracheal tubes that are too long and extend far beyond the patient’s nose would be an example. Adding this dead space presents a further challenge to patients trying to effectively ventilate.
Figure 1.3. Anatomical dead space where no gas exchange takes place. (Drawing by Melanie Tong.)

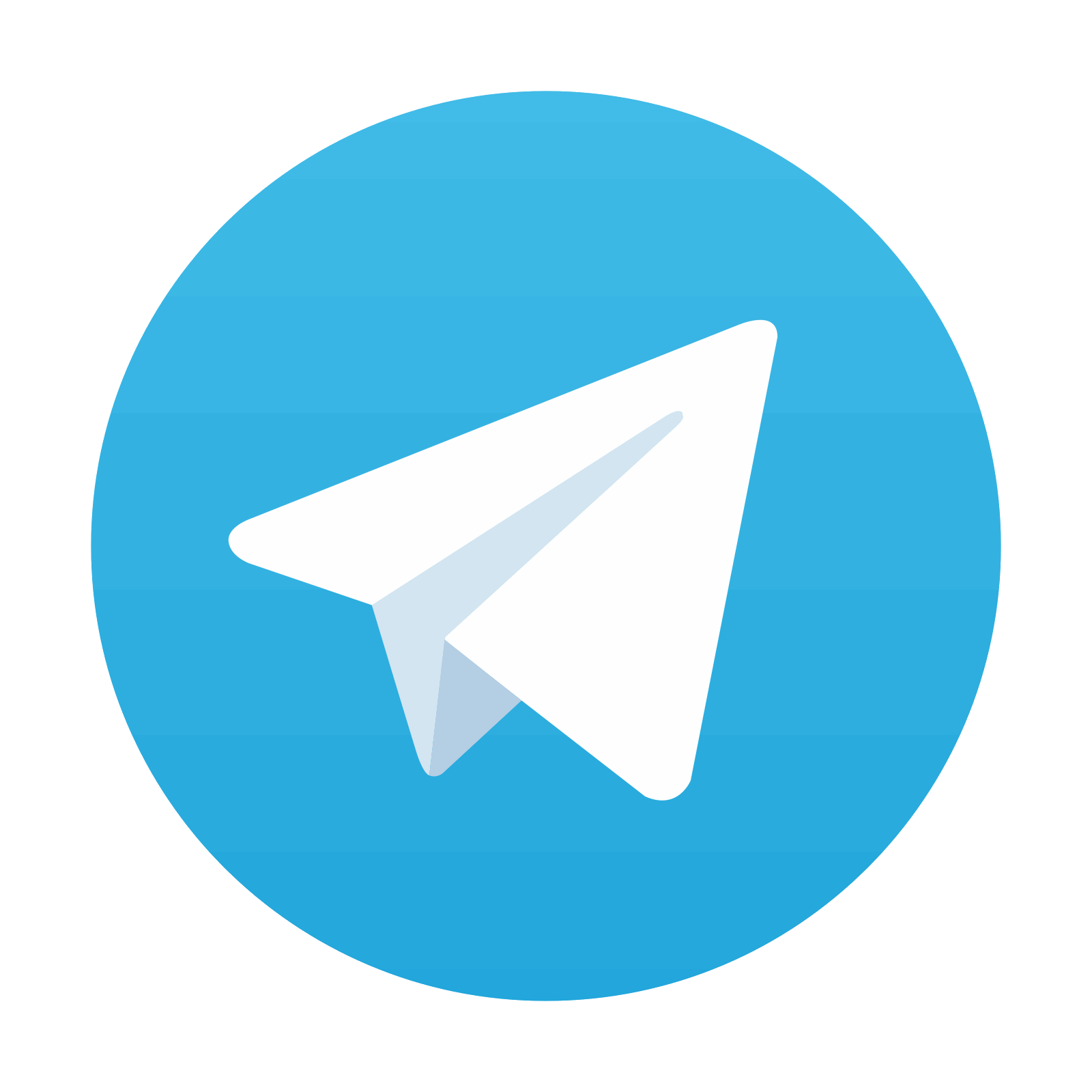
Stay updated, free articles. Join our Telegram channel

Full access? Get Clinical Tree
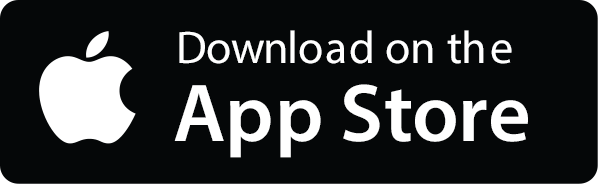
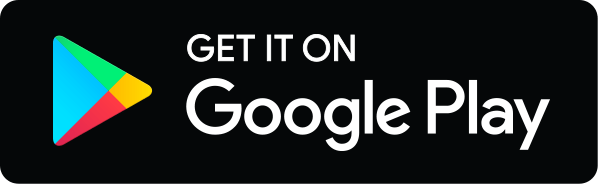