CHAPTER 21 Assisted reproduction technologies
Over thousands of years, mankind has slowly altered the traits of domestic animals to meet the needs of production. Over recent decades, more and more complicated manipulations of gametes and embryos have emerged as a means to that end. Collectively, these techniques are referred to as assisted reproduction technologies (ARTs).
ARTIFICIAL INSEMINATION
The procedural steps of AI will be described for cattle, with the peculiarities of pig, horse, small ruminant, and dog and cat AI discussed subsequently. Basic parameters of the semen of each of these species are presented in Table 21-1.
Artificial insemination in cattle
Semen evaluation
The concentration of spermatozoa in an ejaculate is a major quality characteristic and determines how many doses can be produced from a particular semen collection. The concentration is normally assessed by photometry, microscopy, or flow cytometry.
Semen dilution, packaging and freezing
After packaging, the semen doses must be stored properly until used for insemination. In cattle, this is usually by cryopreservation. The doses are prepared by dilution of the semen sample with a cryoprotective extender, usually so that each dose can be packed into a plastic straw containing 0.25 ml. The straws are then frozen, either by suspension in the vapour above liquid nitrogen for 10 min or by using a microprocessor-controlled freezer, and stored in liquid nitrogen until used. Thawing (which should immediately precede insemination) needs to be rapid; slow thawing leads to extensive crystallization of water and consequent damage to sperm membranes.
Artificial insemination in the pig
A phantom is used for support of the boar during ejaculation, and the semen collection is performed using the gloved-hand technique, which involves a steady firm pressure applied to the spiral-shaped portion of the penis. The ejaculate of the boar is fractionated, and so collection is normally done through a filter cloth to avoid mixing the more gelatinous portion with the sperm-rich fraction (Fig. 21-1).
Insemination of the sow or gilt is easy because the porcine cervix presents neither a portio vaginalis nor circular plicae. Instead, the vagina gradually leads into the cervix through a funnel and, in the cervical canal, the pulvini cervicales create a softer closure. This allows the insemination catheter to be positioned with its tip firmly interlocked with the pulvini cervicales, just as the spiral-shaped portion of the penis would be during copulation. Interestingly, it is now clear that stimulation of the sow or gilt by massage and riding on the back improves the results of insemination by helping the uterus transport semen to the oviducts. Often, a repeat insemination is performed if oestrus is sufficiently prolonged (see Chapter 2).
Artificial insemination in the dog and cat
Collection of semen from a tomcat requires extensive training and patience, and the presence of a receptive female. Semen can be collected in a tiny artificial vagina customized for rabbits. Alternatively, semen can be collected using electro-ejaculation after sedation. Insemination is intravaginal.
Sex sorting of spermatozoa
The ability to separate and sort functional spermatozoa according to whether they carry the X- or the Y-chromosome became a reality in the 1990s, based on their different DNA contents (Fig. 21-2). When stained with the DNA-binding dye Hoechst 33342, X-chromosome-bearing spermatozoa absorb more of the dye than do Y-chromosome-bearing. As a consequence, the X-chromosome-bearing spermatozoa will emit more light than will the Y-chromosome-bearing when excited by a laser in a flow cytometer. In the flow cytometer, this difference is measured extremely rapidly in individual stained spermatozoa, each in its separate micro-droplet formed by pumping the semen sample through a special nozzle at high pressure. An electromagnetic charge (positive, negative, or no charge) is then applied to each micro-droplet depending on the DNA content of the spermatozoon within the droplet, and these can then be directed to one side or the other depending on charge. Furthermore, selection of only viable, membrane-intact spermatozoa is made possible by staining with a food dye. Two defined populations of spermatozoa are therefore achieved after flow cytometric sorting using this method; one vial primarily containing viable X-chromosome-bearing spermatozoa, and another primarily containing viable Y-chromosome-bearing spermatozoa. The method has provided a means of determining the efficacy of any sperm sex-sorting enrichment approach, and is the basis for the sperm sexing system that is now used commercially to predetermine the sex of offspring in several species, primarily cattle. Flow cytometric sex-sorting of spermatozoa according to their DNA content is patented and is sub-licensed for mammals (non-human) to XY Inc., through Colorado State University. Species variation in factors such as DNA content and sperm head size make it necessary to optimize sorting conditions species by species to achieve acceptable results. In cattle, the sperm sexing efficiency is about 90% in a commercial setting.
MULTIPLE OVULATION AND EMBRYO TRANSFER
It has been the detailed knowledge of embryonic development and reproductive biology that has made it possible to use embryos for commercial purposes in breeding, production, and disease control. How embryo-based techniques have developed varies with species because of differences in the anatomy, physiology, and husbandry of domestic animals but the technologies have been most widely applied in cattle. Therefore, the following paragraphs will deal mostly with cattle, with briefer consideration of the other species subsequently.
Multiple ovulation and embryo transfer in cattle
Multiple ovulation (’superovulation’)
Selection of potential donor cows. Because the superovulatory treatment stresses the donor’s endocrine system, selecting a potential donor should include minimization of other stressful factors. A donor should show regular oestrous cyclicity, be in a good nutritional state, not have calved recently or be recovering from a disease, and have normal genital organs upon a thorough clinical examination.
Embryo recovery
Embryos are recovered 6–8 days after the onset of oestrus (Fig. 21-3). At this stage most embryos will have passed through the oviduct and entered the uterine horns, still surrounded by the zona pellucida. They are therefore accessible for collection through a non-surgical flushing of the uterine cavity. At this stage the embryos are fairly tolerant to handling, not only during their recovery but also in additional procedures such as cryopreservation (see later) or biopsy to isolate cells for sex determination (Fig. 21-4).
The procedure for embryo recovery. Embryos are collected by flushing, either both uterine horns at one time or each horn separately, using a special transcervical catheter with an inflatable cuff to occlude the lumen of the horn. The most commonly used flushing medium is modified phosphate-buffered saline (PBS) that contains glucose and pyruvate, substrates that are beneficial for post-compaction bovine embryonic development. The phosphate buffer helps keep the pH of the medium stable under practical conditions. Other additions are usually serum or bovine serum albumin (BSA) to provide nutrients and to prevent the embryos from sticking to plastic surfaces during the flushing and handling, and some form of antibiotic to minimize the risk of uterine infection. Epidural analgesia is routinely used during the non-surgical collection; it facilitates rectal manipulations of the genital tract and the collection catheter, and makes the animal stand more quietly both by reducing her discomfort and because she senses reduced control of her hind legs. Flushing fluid is introduced into the uterine cavity until, by rectal palpation, the uterus is judged to be sufficiently distended, at which point the fluid is drained into a collection vessel. The filling and emptying is repeated several times with additional fluid to ensure that the cavity is properly flushed. The combined flushes are filtered through a mesh that retains the embryos but allows debris and blood cells to pass. After this filtration, the reduced volume of flushing fluid above the mesh (and appropriate rinses) must be searched for embryos, using a stereomicroscope.
Embryo transfer
Synchrony between the embryo and the recipient is optimal when the embryo is transferred to a recipient at exactly the same post-ovulatory stage as the donor. However, for good-quality embryos, pregnancy rates are not affected by asynchronies of as much as 36 hours; embryos of poorer quality, on the other hand, seem less tolerant of asynchrony.
Multiple ovulation and embryo transfer in pigs
Hormonal stimulation to increase the number of ovulations is not often used because the inherently high ovulation rate in pigs makes it unnecessary. Hormonal interventions are, however, used for synchronization purposes in timing embryo collection. This is especially true when young pigs (gilts) are used; in sows, the natural synchronization following weaning is often adequate.
IN VITRO PRODUCTION OF EMBRYOS
In vitro production of embryos in the domestic species is normally performed in three steps: oocyte maturation, fertilization and, finally, embryo culture (Fig. 21-5). Each will be described briefly, using cattle as an example.
In vitro production of embryos in cattle
Oocyte collection and in vitro maturation
Oocytes used for in vitro embryo production may originate from ovaries collected at the slaughterhouse or may be retrieved by ultrasound-guided ovum pickup from genetically valuable animals. Most laboratories producing bovine embryos in vitro routinely collect ovaries at the slaughterhouse several times per week. At the laboratory, the contents of follicles larger than 2–3 mm are aspirated by means of a needle and vacuum pump and the oocytes are isolated from the follicular fluid by stereomicroscopy. This, of course, provides an extremely mixed population of oocytes for further culture: some oocytes come from healthy antral follicles, others from follicles that are more or less atretic. Therefore, oocytes without a cumulus investment (generally from atretic follicles) are discarded, having lost their developmental competence. In the case of ovaries from genetically valuable animals, oocytes may be more efficiently harvested by slicing the ovary with a set of several razor blades mounted in parallel about 1 mm apart; this chopping releases more oocytes from more follicles at various stages of development, including oocytes in their growth phase in secondary and small tertiary follicles. The oocyte’s growth phase ends, and its active transcription ceases, at a diameter (inside the zona pellucida) of around 110 µm, corresponding to the time when the oocyte becomes fully competent to mature to metaphase II and to sustain initial embryonic development.
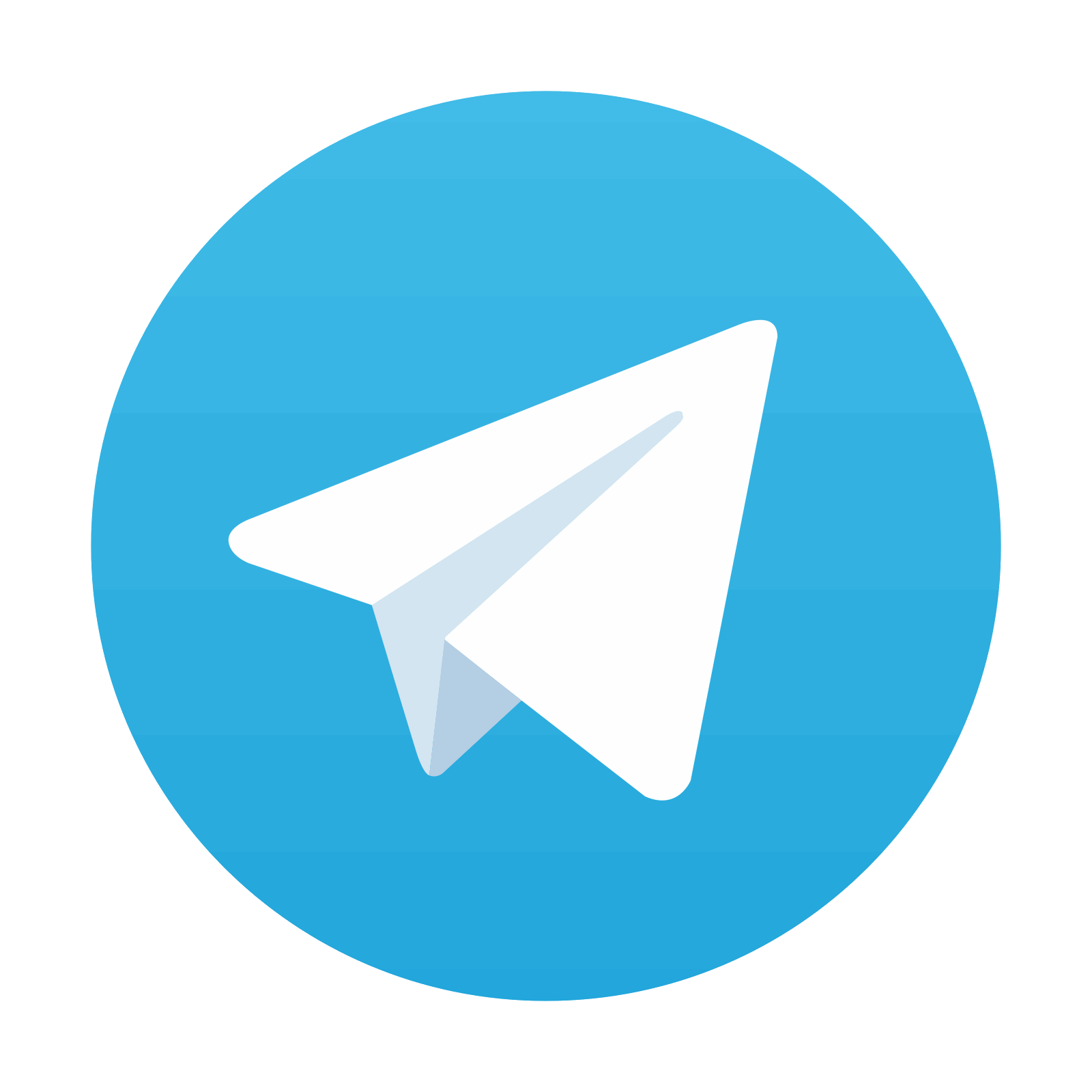
Stay updated, free articles. Join our Telegram channel

Full access? Get Clinical Tree
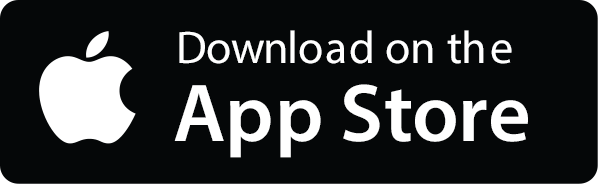
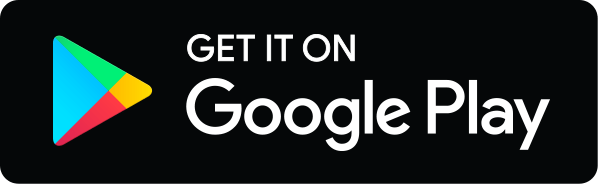