Carrie A. Schroeder Department of Surgical Sciences, School of Veterinary Medicine, University of Wisconsin–Madison, Madison, WI, 53706, USA General anesthesia has profound effects on the body, greatly affecting cardiac output, blood pressure, and perfusion of vital organs. The anesthetist’s task is to minimize these detrimental effects, with the goal of maximizing the resemblance to patient homeostasis. This is no simple task in the healthy patient and can be a daunting task in the face of disease. Renal disease is a common pathology faced when providing anesthesia to today’s pet population. The estimated prevalence of chronic kidney disease (CKD) is 0.5–1.0% in dogs and 1.0–3.0% in cats, increasing to 80% among older cats [1]. Before anesthetizing a patient with either acute kidney injury (AKI) or CKD, it is paramount to understand the effects of renal dysfunction on the patient, and the anesthetic and surgical effects on the kidneys; only then can an anesthetic plan be tailored to the patient and the disease process. Although anesthetic procedures and drug choices may have profound renal effects, the maintenance of normotension, euvolemia, and adequate cardiac output sufficient to maintain renal perfusion has the greatest effect on the prevention of initial or ongoing renal insult. The kidneys serve several functions, including metabolic waste products and toxin excretion, blood volume, extracellular fluid volume, osmolality regulation, and electrolyte balance. The kidneys also serve important roles in acid–base regulation, erythrocyte production, and hormone secretion. Each kidney is divided into cortical and medullary portions and is made up of thousands of nephrons, the functional kidney units [2]. The nephron comprises the glomerulus and the renal tubule (Figure 6.1a). The glomerulus is a unique ball of capillaries interposed between two sets of arterioles with a high hydrostatic pressure, favoring fluid filtration. Fluid filtered from the glomerulus enters the renal tubule, where, depending on several factors, reabsorption and secretion occur, resulting in the end product of urine (Figure 6.1b). Despite their relatively small size, the kidneys receive an impressively high proportion of cardiac output, roughly 20–25% [2, 3]. The vast majority (90–95%) of blood flow is directed to the renal cortex, with the remainder perfusing the renal medulla [3]. High blood flow serves to match the extremely high oxygen consumption of the kidneys. On a per‐gram basis, the kidneys have roughly twice the oxygen consumption of the brain, but nearly seven times the blood flow [4]. As oxygen delivery to the kidneys exceeds the consumption under normal circumstances, the arteriovenous difference in the kidneys is relatively low. Figure 6.1 (a) Schematic representation of the nephron and glomerulus. (b) Schematic representation of urine formation. [1] Filtration of blood at the level of the glomerulus. [2] Reabsorption of substances from renal tubules by peritubular capillaries. [3] Secretion of substances from peritubular capillaries into renal tubules. [4] Excretion of the urine. Urinary excretion is the net result of filtration minus reabsorption plus secretion (excretion = filtration – reabsorption + secretion). Source: Adapted from Guyton and Hall Textbook of Medical Physiology, 2021, with permission. In general, renal blood flow (RBF) is directly related to glomerular filtration rate (GFR). However, renal autoregulation serves to stabilize GFR, despite large swings in blood pressure (Figure 6.2). This occurs via several extrinsic and intrinsic [4] mechanisms at mean arterial blood pressures from approximately 70–180 mmHg [4]. Although GFR is preserved within this autoregulatory range, several factors can alter this process and lead to renal ischemia, despite apparent normotension. For example, sympathetic activation, as occurs in the presence of pain or surgical stimulation, can lead to renal vasoconstriction and hypoperfusion of the kidney [3]. Although autoregulation is preserved with most anesthetics, anesthetic drugs may cause a redistribution of blood flow away from the renal cortex [3]. Autoregulation may also be altered in the presence of chronic hypertension, acute renal failure, and sepsis [3]. In the face of these disease processes, mean arterial pressure must be maintained at a higher range to preserve autoregulation of RBF. Renal disease is broadly classified as AKI or CKD. AKI can occur over a period of hours to days and is due to several processes, including toxicities, infection, and inflammatory conditions. Broadly, perioperative injury can be due to prerenal AKI (e.g., hypovolemia or severe dehydration), direct renal injury, or postrenal AKI (e.g., urinary obstruction) [5]. Perioperative acute tubular necrosis may be due to ischemia–reperfusion injury or nephrotoxicity (sources may include antibiotic therapy or myoglobin) [5]. Some of the more common perioperative causes of AKI include urethral obstruction, sepsis, and infectious disease. Indeed, AKI has been reported to occur in 12% of dogs with abdominal sepsis [6]. In bitches presenting with pyometra, 20 out of 20 dogs presented with some degree of renal tissue injury including tubular degeneration or necrosis and/or glomerulonephritis, meeting the criteria for AKI [7]. Despite this presence of damage, only 40% of those patients met the criteria for sepsis via the sequential organ failure assessment score. It is important to be aware that kidney injury may be present or impending in any animal presenting with an acute abdomen‐type condition that may lead to sepsis or renal damage. Figure 6.2 Autoregulation of renal blood flow (RBF) and glomerular filtration rate (GFR). Renal autoregulation stabilized GFR within a range of mean arterial pressures of approximately 70–180 mmHg, while urine output is directly related to changes in blood pressure. Source: Guyton and Hall Textbook of Medical Physiology, 2021, with permission. Animals with AKI may present for any number of procedures, from brief urethral catheter placement to major exploratory laparotomy. AKI can also result from anesthesia and surgery. In humans, this is often due to a combination of prerenal azotemia and acute tubular necrosis [8]. While idiosyncratic cases can occur with no apparent intraoperative insults, tubular necrosis can occur perioperatively resulting from a prolonged decrease in renal perfusion due to decreased cardiac output, severe hypotension, or inflammatory processes [8–10]. Fortunately, the healthy kidney is relatively tolerant of perioperative insults, and multiple and/or severe insults are generally required to produce injury enough to cause a significant decrease in renal function [8]. Judicious fluid use and blood pressure maintenance, in addition to avoiding nephrotoxic agents and ischemia, are often sufficient to prevent perioperative AKI. Patients with AKI can present with coexisting abnormalities in acid–base status, electrolytes, and the biochemical profile that may affect patient anesthetic management (Table 6.1). Thus, before attempting general anesthesia, it is important to perform a thorough patient evaluation, including assessment of hydration, hemodynamics, electrolyte and acid–base status, blood pressure, and biochemical profile. Table 6.1 Physiological abnormalities commonly associated with acute kidney injury (AKI). ↑ and ↓ indicate an increase and decrease, respectfully, in a parameter, – indicates no change. AKI describes a wide range of severity and prognoses. Many classification schemes have been described in humans, including the RIFLE (risk, injury, failure, loss of kidney function, and end‐stage kidney disease) and AKIN (acute kidney injury network) systems [11]. In veterinary medicine, the International Renal Interest Society (IRIS) has developed recommendations for AKI grading in dogs and cats on a scale of I to V (Table 6.2) [12]. Prognosis is based on grading; animals with IRIS AKI Grades I and II that are appropriately managed may regain renal function within 2–5 days, while animals that fall in Grades IV to V may die within 5–10 days [12]. Overall, patient prognosis with AKI can be quite poor, with an overall mortality rate of 45–53% in small animals [13]. The risks versus benefits of performing general anesthesia on patients with AKI need to be assessed, and, if necessary, anesthesia should be delayed until appropriate patient stabilization has been achieved. However, the reality is that many patients with AKI, such as patients presenting with urethral obstruction (see Chapter 7), may require general anesthesia to attain appropriate stabilization, especially if sedation or regional anesthesia is not an option. CKD is the structural or functional disease of one or both kidneys that has been present for a longer duration, generally 3 months and longer [14]. CKD is due to a myriad of underlying problems and, unlike AKI, is considered progressive and irreversible. The diagnosis can be made based on several hallmark abnormalities, and a single test is generally insufficient to diagnose CKD. Like AKI, The IRIS has developed guidelines to stage renal disease on a scale of 1–4 based on renal function tests (Table 6.3). Patients are then substaged based on the presence or absence of proteinuria and hypertension. Complete guidelines for renal disease staging, as well as current algorithms for staging in small animals, can be found on the IRIS website (http://www.iris‐kidney.com). Based on the animal’s IRIS stage, appropriate prognosis and treatment can be pursued by the managing clinician. Animals presenting for general anesthesia with a history of CKD, especially those that have had a diagnostic workup and have been assigned an IRIS stage, generally are accompanied by a history of diagnostic tests and have been chronically managed medically. Newly diagnosed patients should be staged and stabilized with medical treatment before undergoing general anesthesia. Unfortunately, this is not always the case. All patients presenting with CKD should have baseline diagnostic tests performed before formulating an anesthetic plan, including a thorough evaluation of hydration, cardiovascular status, blood pressure, complete blood count, biochemical profile, and evaluation of electrolytes and acid–base status. In addition to standard biochemical screening that includes blood urea nitrogen (BUN) and creatinine, a fasting symmetric dimethylarginine (SDMA) immunoassay should be performed. SDMA is a biomarker that reflects GFR and is a more sensitive indicator of renal function [15]. Generally, SDMA levels below 14 μg dl−1 are considered acceptable and are within the reference interval. It is important to realize that these patients can present with several abnormalities (Table 6.4). Age‐related comorbidities such as diabetes mellitus and cardiovascular disease are also frequently encountered [7]. Stabilization and management of sequelae of the primary disease, as well as any comorbidities, are keys to successful management of CKD. Table 6.2 International Renal Interest Society (IRIS) staging of acute kidney injury (AKI) [12]. Stress and pain associated with surgery can have significant effects on the kidney. Catecholamine, renin, arginine vasopressin (AVP), and aldosterone release associated with surgical stimulation often lead to an increase in renal vascular resistance and decrease in RBF and GFR [5, 16]. Systemic analgesic drugs or regional anesthetic techniques may attenuate this response, but even patients without preexisting renal disease can experience perioperative kidney dysfunction because of anesthesia and/or surgery. The perioperative period is a time of extreme physiologic stress and it is important to anticipate possible negative consequences of anesthesia and surgical procedures. Patients that present with preexisting AKI or CKD should be optimized prior to surgery to minimize the detrimental effects of the perioperative period (see the section titled “Pre‐anesthetic Stabilization”). Table 6.3 International Renal Interest Society (IRIS) staging of chronic kidney disease (CKD). Source: Complete details and substaging may be found at www.iris‐kidney.com. Surgical manipulation may compromise RBF either through direct compression of vasculature associated with the kidneys or through increases in intra‐abdominal pressure. Renal hypoperfusion and venous congestion can occur through abdominal organ manipulation and elevations of central venous pressure [16]. Pneumoperitoneum associated with the insufflation in laparoscopic surgery can increase intra‐abdominal pressures significantly; abdominal compartment syndrome, defined as sustained increases in intra‐abdominal pressure >20 mmHg, may lead to organ dysfunction [5]. However, more modest increases in intra‐abdominal pressure (>12 mmHg) have been associated with a higher risk of renal damage [17]. AKI and oliguria may be consequences of intraoperative and postoperative intra‐abdominal hypertension and abdominal compartment syndrome [17, 18]. Table 6.4 Physiological abnormalities commonly associated with chronic renal disease. ↑ and ↓ indicate an increase and a decrease, respectfully, in a parameter. In addition, any intervention that decreases cardiac output can similarly affect RBF and GFR. This includes the cardiac depression that may be associated with general anesthesia, positive pressure ventilation (PPV), and positive end‐expiratory pressure (PEEP). It is important to closely monitor blood pressure and cardiorespiratory parameters as surrogate measures of cardiac output and address concerns accordingly to maintain adequate RBF. Optimizing hydration status and achieving euvolemia should attenuate the hemodynamic responses associated with positive pressure ventilation such as decreased venous return and pulse pressure variation, but increased airway and pleural pressures associated with PPV and PEEP can lead to renal vasoconstriction, antidiuresis, and anti‐natriuresis [15]. Increasing levels of PEEP can lead to increases in renin and aldosterone and depression of cardiac output, RBF, GFR, and urine volume [15]. When utilizing PPV and/or PEEP, more conservative ventilator settings are recommended. Acepromazine is a relatively common sedative utilized in modern veterinary practice. It belongs to the phenothiazine class of neuroleptics and produces mild to moderate sedation lasting anywhere from 1–6 h, depending on dosage, and no direct‐acting drug antagonist is available [19, 20]. Acepromazine causes blockade of dopaminergic and alpha‐1‐adrenergic receptors, inducing vasodilation that may lead to hypotension below the range of renal autoregulation [21, 22]. The concurrent use of volatile anesthetics and their accompanying vasodilation and hypotension may worsen phenothiazine‐induced hypotension. However, incremental dosages of acepromazine administered intravenously under isoflurane anesthesia increase cardiac index and oxygen delivery and preserve systemic blood pressures [23]. Renal function as measured by GFR, RBF, and blood analytes was preserved in dogs administered acepromazine prior to anesthetic induction, despite systemic hypotension [21]. Since no drug antagonist is available, one must use caution in choosing this agent in patients that may not tolerate prolonged sedation or the possible accompanying systemic hypotension associated with its use. Careful blood pressure monitoring is advised following administration of acepromazine, especially to patients with preexisting renal disease. This drug class includes agents such as xylazine, detomidine, romifidine, medetomidine, and dexmedetomidine. In small‐animal patients, the most relevant alpha‐2‐adrenergic agent is dexmedetomidine. Each of these agents significantly decreases heart rate and cardiac output while increasing systemic vascular resistance [24–27]. Medetomidine administration, the racemic mixture of levomedetomidine and dexmedetomidine, along with propofol induction decreased cardiac output by approximately 60% and decreased RBF by approximately 50% in dogs [28]. However, GFR was increased despite these changes. Another study evaluating the renal effects of medetomidine in dogs revealed variable effects dependent on the method of drug administration. For example, intramuscular administration of medetomidine induced decreases in RBF and GFR, whereas intravascular administration induced opposite effects, increasing RBF and GFR [29]. These contradictory effects may be attributed to the immediate systemic hypertension following intravenous administration, an effect less drastically observed with intramuscular administration [29]. Slow administration may attenuate the hemodynamic responses associated with rapid or bolus administration. Constant rate infusion (CRI) of dexmedetomidine in dogs anesthetized with sevoflurane decreased cardiac index but had no effect on GFR or RBF [27]. A diuretic effect is often observed with these agents owing to several possible mechanisms, including inhibition of AVP, natriuresis, and osmotic diuresis due to insulin inhibition and resulting hyperglycemia [27, 29]. Therefore, the use of alpha‐2‐adrenergic agonists in patients with unresolved urinary obstruction should be avoided. Owing to the hemodynamic instability and possible associated decreases in RBF as well as wide blood pressure variations, alpha‐2‐adrenergic agonists should be used judiciously. When administered intravenously, agents should be administered slowly and titrated to effect. Doses administered intramuscularly should be carefully calculated and kept to a minimum. This can be achieved by the coadministration with other agents with minimal cardiac effects, such as opioids or benzodiazepines. Blood pressure and heart rate should be monitored, at minimum, when these agents are administered. The benzodiazepines, most commonly midazolam and diazepam, exert a sedative effect via enhancement of the endogenous inhibitory neurotransmitter, gamma‐aminobutyric acid (GABA) [30]. Their cardiovascular effects are mild, and they are well‐tolerated in patients with many disease states, including renal disease [31]. Although benzodiazepine use is generally recommended and considered safe, caution must be exercised in using these agents in animals that may demonstrate paradoxical excitement on administration, most notoriously juvenile patients, and young cats [32]. Sole administration of benzodiazepines to most veterinary patients is generally not suggested for this reason, and, to improve sedation, coadministration with an opioid or other sedative is advised. When used appropriately, benzodiazepines are an excellent choice for patients either with preexisting renal disease or in disease states that may predispose them to renal damage (e.g., sepsis, hypovolemic shock). The opioids can be broadly classified into agonists, agonist–antagonists, and partial agonists based on their effects on opioid receptors [33]. Opioid agonists include, among others, morphine, hydromorphone, fentanyl, and methadone; these exert a pharmacologic effect via interaction with the mu‐opioid receptor. Butorphanol is a commonly used agent in veterinary medicine and is classified as an opioid agonist–antagonist, acting as an antagonist at the mu‐opioid receptor and an agonist at the kappa‐opioid receptor. Buprenorphine, a partial agonist, acts as an agonist at the mu‐opioid receptor but exhibits a submaximal response as compared to opioid agonists [34]. This submaximal response does not necessarily reflect analgesia, as buprenorphine has proven moderate, although variable, analgesic efficacy in several species, especially as part of a multimodal analgesic plan [35, 36]. Direct adverse renal effects of the opioids are generally mild and of little consequence for patients with renal disease [37, 38]. However, bradycardia associated with high opioid dosages may lead to decreases in the cardiac index, potentially impacting RBF [39]. Significant bradycardia should be treated as necessary to restore hemodynamic stability. The profound analgesic effects of this pharmacologic class may attenuate the sympathetic response associated with surgical pain, thus minimizing renal vasoconstriction. Furthermore, minimal alveolar concentration (MAC) of volatile anesthetics is reduced with concurrent opioid use. For example, oxymorphone and hydromorphone administration decreased the MAC of isoflurane in dogs by approximately 45%, while fentanyl decreases isoflurane MAC in a similar, dose‐dependent manner [40, 41]. Decreases in MAC associated with opioid use, which have minimal direct effects on blood pressure, may attenuate the systemic hypotension associated with volatile anesthetics. Multiple opioids are metabolized by extrarenal pathways, and duration and effect are largely unaffected by renal failure [42, 43]. However, active morphine and meperidine metabolites rely on renal excretion; significant renal disease may result in untoward effects such as prolonged sedation, respiratory depression, or neuroexcitation [42,44–48]. For example, morphine is metabolized in most species to morphine‐3‐glucuronide and morphine‐6‐glucuronide, both eliminated by glomerular filtration. Morphine‐6‐glucuronide has similar activity to the parent drug, and significant renal impairment resulting in compromised excretion of this metabolite has been shown to lead to prolonged clinical effects in human patients [44–46]. Similarly, the metabolite of meperidine, normeperidine, has been reported to cause seizures in humans with renal impairment [47, 48]. Nonsteroidal anti‐inflammatory drugs exert their effects via inhibition of the cyclooxygenase (COX) and lipoxygenase (LOX) enzymes that oxidize arachidonic acid to various prostaglandins and leukotrienes. The result is inhibition of important mediators of pain and inflammation, such as prostaglandin E2 and prostacyclin [49–51]. COX enzyme isoforms, including COX‐1 and COX‐2, are constitutively expressed in multiple species, including dogs and cats [52]. Protective prostaglandins are derived largely from the COX‐1 isoform; such prostaglandins are thought to induce renal protection under conditions of renal ischemia. Under stressful conditions such as that induced by general anesthesia and surgery, RBF can become prostaglandin‐dependent. Furthermore, animals with CKD may have altered COX expression [52]. COX‐1‐sparing NSAIDs such as meloxicam and carprofen and selective COX‐2 inhibitors such as robenacoxib are considered more kidney‐sparing than nonpreferential NSAIDs such as aspirin [53, 54]. Multiple studies evaluating these agents in healthy canine and feline patients have failed to reveal detrimental renal effects, even under conditions of moderate hypotension [28,54–62]. However, adverse effects have been reported, including renal insufficiency in cats following use of meloxicam, and the safety of these drugs in small animals with preexisting renal disease in the perioperative period is still questionable [63]. Evidence of renal dysfunction following periods of experimentally induced dehydration has been documented [64]. Subclinical renal damage in patients receiving perioperative NSAIDs that experience periods of hypotension or hypoperfusion cannot be fully ruled out, and the perioperative safety of these agents under conditions of true clinical hypovolemia, hypotension, and hypoperfusion has not been definitively proven. In the acute perioperative period, judicious use of these agents is recommended. Administration should only occur in patients that have not experienced hypotensive episodes, and extreme caution should be exercised in patients with preexisting renal dysfunction or patients at risk for AKI (e.g., sepsis). For long‐term analgesia in animals with chronic pain and concurrent CKD, the documented benefit may far outweigh any possible risk, and safety of these agents for long‐term administration has been documented [65, 66]. Relatively new NSAIDs that inhibit the prostaglandin E2 EP4 receptor (EP4 prostaglandin receptor antagonist, or PRA), such as grapiprant, are effective analgesics used primarily for osteoarthritis [67]. At this time, no significant adverse effects have been reported in long‐term, oral use [68]. However, efficacy for acute, perioperative pain has not been evaluated. Until clinical studies demonstrate the utility of these EP4 antagonists for acute pain, agents of this class cannot be recommended for surgical analgesia. Alfaxalone is a synthetic neurosteroid that, like many other anesthetic agents, potentiates the inhibitory action of GABA with the GABAA receptor [69]. This agent is unique among anesthetic induction agents in that it can produce this effect and induce varying levels of sedation and anesthetic induction through administration by either intramuscular or intravenous routes. Following administration of alfaxalone, cardiovascular function is reasonably well‐maintained and significant decreases in cardiac output are not to be expected [70–73
6
Renal Disease
Introduction
Anatomy and Physiology
Pathophysiology
Abnormalities commonly associated with acute renal injury
↑ BUN
↑ Creatinine
↑, ↓, or – Na+
↑ K+
↑ P
↑, ↓, or – urine output
Metabolic acidosis
↑ Respiratory rate
Hypertension or hypotension
Cardiac dysrhythmias
Nausea and vomiting
AKI grade
Creatinine (mg dl−1)
Clinical description
I
<1.6
Nonazotemic AKI
Documented AKI (historical or clinical)
Progressive increases in creatinine (≥0.3 mg dl−1 within 48 h)
Oliguria or anuria over 6 h
II
1.7–2.5
Mild AKI
Documented AKI
Static or progressive increases in creatinine (≥0.3 mg dl−1 within 48 h)
Oliguria or anuria over 6 h
III
2.6–5.0
Moderate to severe AKI
IV
5.1–10.0
Documented AKI
V
>10.0
Increasing severity of azotemia
Functional renal failure
Renal Disease and Surgery
IRIS stage
Creatinine (mg dl−1)
SDMA (μg dl−1)
Dog
Cat
Dog
Cat
1
<1.4
<1.6
<18
<18
2
1.4–2.8
1.6–2.8
18–35
18–35
3
2.9–5.0
2.9–5.0
36–54
26–38
4
>5.0
>5.0
>54
>38
Abnormalities commonly associated with chronic renal disease
↑ BUN
↑ Creatinine
↑ SDMA
↑ or ↓ K+
↑ Mg
Ca2+
↑ P
Metabolic acidosis
↑ Respiratory rate
Hypertension
Anemia
Uremic coagulopathy
Hypoalbuminemia
Dehydration
Nausea, vomiting
Renal Disease and Pharmacologic Agents
Pre‐anesthetic Agents
Phenothiazines
Alpha‐2‐Adrenergic Receptor Agonists
Benzodiazepines
Opioids
Nonsteroidal Anti‐Inflammatory Drugs (NSAIDs)
Induction Agents
Alfaxalone
Stay updated, free articles. Join our Telegram channel

Full access? Get Clinical Tree
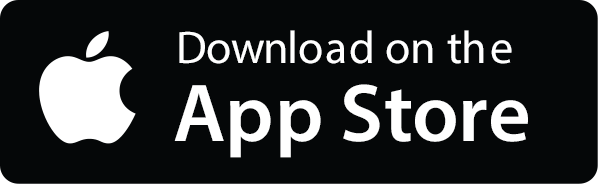
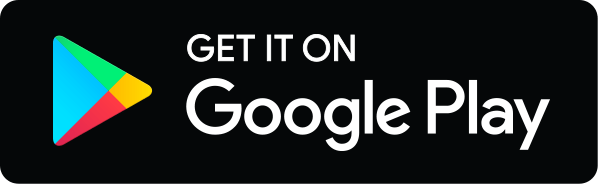