Turi K. Aarnes1 and Melissa A. Murdock2 1 Department of Veterinary Clinical Sciences, College of Veterinary Medicine, The Ohio State University, Columbus, OH, 43210, USA 2 BluePearl Specialty and Emergency Pet Hospital, Levittown, PA, 19056, USA Anesthetic drug selection, delivery, and monitoring techniques for pregnant dogs and cats are similar to those used for the nonpregnant animal. It is, however, critically important to account for changes in maternal physiology and concerns for fetal viability, which often influences drug selection, delivery, and anesthetic monitoring. When planning for cesarean‐section anesthesia, provisions should be made for neonatal resuscitation and postdelivery care. The emergent nature of many cesarean sections often results in limited availability of trained and knowledgeable staff. Puppy mortality is approximately two‐thirds greater during emergent than during planned cesarean sections [1]. Maternal mortality is also significantly greater in emergent versus planned cesareans [2]. For this reason, anesthesia and delivery must be well planned before anesthetic induction. Oxygen, clean absorbent towels, a suction apparatus, and resuscitation and antagonist drugs should be available and organized before surgery. Pregnancy creates major maternal physiologic and anatomic adaptations associated with the increased metabolic demand imposed by the growing fetal and uterine mass [3]. Many or most of these adaptations impact periparturient anesthetic management, particularly those management aspects related to cardiorespiratory, gastrointestinal (GI), and hematological systems (Table 18.1). During pregnancy, oxygen consumption increases to meet the increased metabolic demand associated with the growing fetus(es). Changes to account for this increased demand begin to occur early during pregnancy and peak during the last trimester. Cardiovascular function returns to prepartum values within 4–12 weeks postpartum in women [4]. Cardiac output increases 30–40% and gravid uterine blood flow is 20–40 times greater than nonpregnant flow [3]. Blood flow to the mammary glands and skin also increases. The increased cardiac output is associated with a decrease in vascular tone and arterial blood pressure (approximately 10%) and an increased heart rate (approximately 55%) and stroke volume [3]. Blood volume increases up to 35% in women [3] and 23% in dogs [5]. Accompanying this increased blood volume is an increase in red cell mass. However, plasma volume increases relatively more than red cell mass, resulting in a pregnancy‐associated anemia. The magnitude of this anemia correlates with increasing fetal numbers [6]. Blood loss during normal parturition is buffered by this increased blood volume and red cell mass. Pregnancy‐associated anemia will influence the use of packed cell volume as an index of dehydration. Table 18.1 Maternal physiology changes associated with pregnancy (see text for details). Uterine and placental blood flow is not autoregulated (maintained constant over a wide range of perfusion pressure); therefore, anything that inhibits uterine arterial flow will result in decreased fetal oxygen and nutrient delivery. This includes anesthetic drug‐ and hemorrhage‐induced hypotension. Anything causing vasoconstriction can also result in decreased uterine perfusion, including vasoactive drugs (i.e., alpha‐2‐adrenergic receptor agonists, norepinephrine, etc.), excitement‐induced or iatrogenic hyperventilation leading to respiratory alkalosis, and sympathetic stimulation such as pain. In supine women, uterine blood flow is compromised because of accompanying aortocaval compression. This can lead to a decrease in venous return and cardiac output with a potential for reduced fetal oxygenation. This condition may be treated with a lateral tilt position, placing a wedge or towels under the right hip to alleviate aortocaval compression by the gravid uterus. Canine studies indicate that dorsal recumbency is not associated with a decrease in systemic blood pressure or altered arterial blood gas values [7]. These studies did not, however, investigate changes in uterine blood flow. In pentobarbital‐thiamylal‐anesthetized pregnant dogs, ligation of the inferior vena cava caudal to the renal veins did not result in diminished venous return with radiographic evidence of ample collateral venous return [8]. Thus, dorsal recumbency during anesthesia does not result in hypotension in the hemodynamically normal pregnant dog. While research suggests that pregnant dogs do not experience significant hemodynamic effects of uterine aortocaval compression, it is important to closely monitor the cardiovascular status of the pregnant patient and note any changes or indicators of hemodynamic instability when placed in dorsal recumbency. Vasopressor and chronotropic drugs are less effective in the parturient, possibly related to a downregulation of alpha‐ and beta‐adrenergic receptors and possibly increased vasodilatory prostaglandins [3]. For this reason, parturients with dehydration or cardiovascular compromise should receive appropriate fluid therapy before anesthetic induction because anesthetic drugs are typically associated with varying degrees of cardiovascular depression. Blood pressure should be monitored, and hypotension treated with crystalloids or colloids. Hemorrhage decreases arterial blood pressure in pregnant dogs relatively more than in nonpregnant dogs [5]. Therefore, hemorrhage and dehydration should be aggressively treated before anesthesia. Ephedrine improves blood pressure in pregnant ewes without decreasing uterine blood flow, while dopamine and dobutamine effectively improve maternal blood pressure but decrease uterine blood flow in nonanesthetized pregnant ewes [9]. The decrease in uterine blood flow is dose‐dependent and relatively greater for dopamine than dobutamine. Consequently, ephedrine is commonly used in parturient hypotensive women. Ephedrine can be used in dogs and cats as a bolus of 0.03–0.1 mg kg−1intravenous (IV) and dobutamine at 2–4 μg kg−1 min−1 IV. Tidal volume, respiratory rate, and minute ventilation increase by approximately 70% in women at term [3], despite the enlarged abdomen and anterior movement of the diaphragm. However, functional residual capacity (FRC) decreases, leading to decreased oxygen reserve within the lung. The decreased FRC and the increased oxygen consumption associated with pregnancy mean that long periods of apnea can more readily result in hypoxemia and hemoglobin desaturation. Because of this, preoxygenation before anesthetic induction is advisable, and apnea should be aggressively avoided during anesthesia. Progesterone‐induced maternal hyperventilation results in mild respiratory alkalosis [10] and an accompanying mild increase in PaO2. The enlarged uterus increases intragastric pressure. Increased circulating progesterone decreases lower esophageal sphincter pressure and GI motility. The increased intragastric pressure and the decreased GI motility result in a greater risk for regurgitation and aspiration during anesthesia. Concentrations of the peptide hormone gastrin, which stimulates production of stomach acid, increase in pregnant dogs during anesthesia [11]. The decreased gastric pH and regurgitation can result in a greater incidence of aspiration pneumonia and possibly esophageal inflammation. Rapid intubation with a cuffed endotracheal tube will help prevent aspiration of stomach contents. It is currently not known if a preoperative proton‐pump inhibitor or histamine H2‐receptor antagonists will lessen these risks. Serum progesterone increases during pregnancy. This and possibly a pregnancy‐associated endorphin increase are thought to be responsible for a 16–40% decrease in minimum alveolar concentration (MAC) of inhalants [12]. This decreased requirement may not be noticed during cesarean‐section anesthesia, as preanesthetic tranquilizers and sedatives that reduce MAC are often limited or not used. Inhalational anesthetics depress fetal blood pressure and minute ventilation. Therefore, high inhalant concentrations should be avoided, and local anesthetic techniques and epidurals should be considered to reduce inhalant anesthetic requirement and fetal cardiopulmonary depression. Little is known regarding the extent of drug passage across the placenta of dogs and cats. Most information is extrapolated from laboratory animal studies [13]. Because of species differences in placentation, extrapolation of placental drug transfer in dogs and cats from other species can be misleading. The placenta has some ability to metabolize drugs and also some carrier proteins on the maternal and fetal sides of the placenta are capable of transferring drugs across the placenta. The extent of such placental metabolism and transport is unknown for dogs and cats. The safest approach is to assume that most drugs cross the placenta and affect the developing fetus(es). The developing fetus(es) are most vulnerable to teratogenic drug effects during the first trimester—the first 20 days of gestation in dogs and cats. Therefore, elective surgical or medical procedures requiring anesthesia should be avoided during these first 20 days of gestation. A fundamental principle of cesarean‐section anesthesia is to minimize fetal concentrations of anesthetic drugs with respiratory, cardiovascular, and CNS depressant properties. In addition, drugs that can be antagonized, such as opioids, are advantageous because their effects can be reversed using naloxone in puppies or kittens after delivery. Little is known concerning placental transfer of anesthetic drugs in dogs and cats and the respective maternal/fetal plasma concentration ratios. Studies on sheep confirm that medetomidine, propofol, ketamine, and etomidate rapidly cross the placenta to develop high fetal concentrations. Conversely, the placental barrier may be effective in decreasing fetal exposure to dexmedetomidine administered to the dam during elective cesarean sections [14]. No detectable dexmedetomidine was present in the amniotic fluid, while a detectable amount was found in the placentas. A majority of puppies were considered vigorous at birth, and no puppy required antagonism of the dexmedetomidine at birth or at hospital discharge [14]. Anesthetic drugs cross the placenta by simple diffusion according to Fick’s equation of diffusion (Eq. 18.1): [15] where Δq/Δt represents the rate of drug transfer, and K is a diffusion constant that relates to drug molecular weight, pKa, lipid solubility, degree of ionization, and protein binding. A is the surface area for diffusion, C2 − C1 is the drug concentration difference across the placenta, and d is the membrane thickness. Properties favoring rapid placental drug transfer include drugs with a molecular weight <600 Da which are nonionized, highly lipid‐soluble, and minimally protein‐bound. Most anesthetic drugs have molecular weights <300 Da and are relatively lipid soluble. This, coupled with the relatively large placental surface area, means that most anesthetic drugs readily cross the placenta. Exceptions are glycopyrrolate, a relatively large polar molecule, and neuromuscular blocking drugs. The relatively more acidic fetal blood favors ionic trapping within the fetus of weakly basic drugs when drug pKa is close to plasma pH (local anesthetics, ketamine, and opioids), possibly prolonging their action in the fetus. Acidic drugs whose pKa is close to plasma pH (thiobarbiturates) more readily transfer from fetal to maternal plasma. The ultrashort‐acting drugs propofol and etomidate are extremely lipid‐soluble and almost completely nonionized at plasma pH. Propofol, a weak acid with pKa = 11, rapidly and readily crosses the placenta in women achieving an umbilical vein/maternal vein ratio of 0.7–0.85 at fetal delivery [16]. The transfer of propofol in sheep is not as complete, and the fetal elimination half‐life is longer than the maternal half‐life in this species. This might relate to the thicker barrier for diffusion of the ovine placenta compared to that in women [17]. Regardless, propofol’s relatively rapid clearance from maternal and fetal plasma makes it a suitable anesthetic induction drug. Etomidate, a weak base with a pKa = 4.2, achieves an umbilical vein/maternal vein plasma ratio of between 1:2 and 1:24 (0.5 and 0.04, respectively) in women [18]. In sheep, the transfer is not as complete, but relatively high fetal concentrations do occur [19]. The effect of dexmedetomidine and propofol for induction on puppy survival, Apgar scores, maternal plasma drug concentrations, as well as placental and amniotic fluid drug concentrations has been evaluated. Both 2.0 μg kg−1 of dexmedetomidine and 2.5 mg kg−1 of propofol were found to have higher concentrations in the placenta than the dam’s blood, and neither drug was detectable in the amniotic fluid. Placental drug concentrations were not correlated to placental weights. The birth weight of puppies increased with increasing placental weights, which also correlated to better Apgar scores. Dexmedetomidine may be an acceptable option in healthy bitches undergoing elective cesarean section that require additional premedication/sedation. None of the puppies in this study required reversal of the dexmedetomidine with atipamezole [14]. Fetal hemodynamic changes associated with alfaxalone administration were evaluated in pregnant ewes and were compared to propofol and etomidate. Propofol induction had no effect on fetal heart rate; use of alfaxalone increased fetal heart rate, while etomidate lowered it. No difference was found between baseline values and after administration of clinically acceptable induction drug doses in blood flow, velocities, and volumes to the umbilical arteries [20]. Inhalation anesthetics are highly lipid‐soluble and readily cross the placenta. Despite achieving concentrations associated with anesthesia in the fetus(es), isoflurane, sevoflurane, and desflurane have relatively low blood:gas partition coefficients and are rapidly cleared by the newly delivered neonate if they are breathing at delivery. Excessive inhalant concentrations should be avoided to prevent neonatal apnea. Women undergoing cesarean section demonstrate no difference in neonatal viability between isoflurane, sevoflurane, and desflurane, and no difference was detected in neonatal viability in women receiving sevoflurane compared to those receiving a spinal anesthetic [21, 22]. In these studies, nitrous oxide was administered to minimize the inhalant anesthetic concentrations. Methoxyflurane was the only inhalant anesthetic associated with increased canine neonatal death in a survey of anesthesia for canine cesarean section and should be avoided for cesarean section [1]. Isoflurane was associated positively with puppy survival at 7 days after delivery. However, neonatal mortality was no different in puppies delivered during propofol/isoflurane anesthesia or epidural [23]. Sevoflurane or desflurane, because of relatively low blood:gas solubilities, are useful for cesarean‐section anesthesia [21, 22, 24]. Opioids include a variety of natural and synthetic products that produce analgesic effects because of their combination with distinct opioid receptors. The most potent opioid analgesics activate mu‐opioid receptors and include morphine, hydromorphone, oxymorphone, fentanyl, methadone, and meperidine. Buprenorphine is classified as a partial mu‐opioid receptor agonist. Butorphanol is a kappa‐opioid receptor agonist and mu‐opioid receptor antagonist. Maternal opioid administration will result in placental transfer of these drugs to the fetus according to the previously discussed Fick’s equation of diffusion (Equation 18.1). The extent of this transfer differs among opioids [25]. Placental transfer of buprenorphine tends to be low (<10% of placental buprenorphine reaches the fetus), while that of the highly lipid‐soluble fentanyl is quite high, and fentanyl persists in the fetus long after clearing the maternal circulation [25–27]. Significant differences in the sensitivity to opioid‐induced analgesia are observed in animals during maturation compared to adults. The source of these differences is unknown. CNS maturity and the blood–brain barrier, end‐organ sensitivity to opioids, and solubility of different opioids have been postulated as the causes of the differences between age‐related effects of mu‐opioid receptor agonists [28]. Studies have also indicated differences in side effects from adult patients, although these differences are seemingly related to those in drug metabolism and physiology between animals of different ages. The most notable example of these differences is the development of respiratory depression. Respiratory depression, although a concern in adults, is a much more important issue in neonates because even a small change in tidal volume or respiratory rate in a neonate can result in life‐threatening hypoxia. While in utero, the fetal lungs are mostly nonfunctional, and the fetus is dependent on maternal circulation for normoxia and normocarbia. However, after cesarean section, the newborn becomes dependent on its own respiratory system for gas exchange and exhaling the inhalant anesthetic; opioid‐induced respiratory depression may lead to increased neonatal mortality [29]. Nonsteroidal anti‐inflammatory drugs inhibit inflammation and produce analgesia by decreasing prostaglandin synthesis through inhibition of cyclooxygenase (COX) and, in some cases, 5‐lipoxygenase (LOX) enzymes [30]. Prostaglandins cause vasodilation, increase vascular permeability, and sensitize pain receptors to noxious stimuli. In addition, they are responsible for regulating renal blood flow, protecting GI mucosa, and aiding platelet aggregation. COX has two clinically relevant isoforms: COX‐1 and COX‐2. Both are constitutively expressed in select tissues, although COX‐2 is upregulated in the inflammatory cascade. Differences between NSAID effects, efficacy, and toxicity are related to COX enzymes and the inhibitory effects on preexisting COX activities in the patient. LOX products (leukotriene B, leukotriene C, and leukotriene D) are responsible for recruitment of immune mediators and alteration of vascular permeability [30]. NSAIDs have minimal effects on normal cardiovascular and respiratory systems. Side effects are typically the result of COX‐1 inhibition, are dose‐related, and typically include GI ulceration, renal papillary necrosis, and inhibition of platelet aggregation [30]. NSAIDs should be used with caution in pregnant and young animals because of their reduced clearance and immaturity of biotransformation pathways. Fetal exposure to NSAIDs could result in significantly prolonged clearance, and prolonged and adverse effects. These drugs could impact the development of organ maturation or cause significant damage to young organs [31]. Cimicoxib, a COX‐2 selective NSAID, remained below the detectable limit in plasma of most suckling puppies sampled after the dams had received one oral dose. This low level of transfer to the puppies occurred despite high concentrations of the cimicoxib detected in the milk [32]. The low level of transfer of cimicoxib in milk makes it a viable option for postoperative analgesia in the dam once the puppies have been surgically removed. Preanesthetic evaluation should include a thorough physical examination and blood work evaluation (packed cell volume and total protein in healthy animals). A complete blood cell count and biochemistry panel may be helpful to evaluate overall health and determine calcium and blood glucose levels in animals that have been in active labor [33]. In addition, animals undergoing sedation and/or general anesthesia should be held off food for planned cesarean sections. Pregnant animals have an increased risk of regurgitation and aspiration due to decreased lower esophageal sphincter tone and prolonged gastric‐emptying time [34, 35]. An IV catheter should be placed to provide IV access for the administration of anesthetic agents, access in the case of emergency, and for IV fluid administration. Pregnant animals should be preoxygenated when possible (Figure 18.1) [36]. Preoxygenation for 3 min increases the time to desaturation in healthy dogs in which propofol was administered for anesthetic induction [37]. Placental oxygen delivery is an important factor influencing fetal mortality [35]. Some animals may be resistant to the placement of a face mask; therefore, preoxygenation may not be possible. In either case, it is important to intubate pregnant animals as soon as possible after anesthetic induction. Most cesarean sections in dogs and cats are performed on unpremedicated animals because all commonly used anesthetic drugs will cross the placenta [38]. Sedatives and tranquilizers have cardiovascular and respiratory side effects for the dam and fetus that can result in increased fetal mortality. For quiet and relatively calm parturient dogs and cats, preanesthetic sedation can be avoided to minimize cardiorespiratory depression in the newborn. If it is possible to place an IV catheter without sedation, it would be preferable to do so. However, excitable and nervous animals will benefit from preanesthetic sedation. This may provide a smoother anesthetic induction and a decreased need for induction agent and inhalant anesthetic concentrations. Fetal exposure to relatively high inhalant anesthetic concentrations results in excessive neonate respiratory depression immediately after delivery. If a preanesthetic sedative or tranquilizer is required, it is important to carefully consider the effects on the mother and the fetus(es). Xylazine administration is associated with fetal death, but this may have been impacted by the concurrent administration of ketamine. Ketamine, xylazine, and the combination of ketamine and xylazine should be administered with caution or avoided when possible [1]
18
Cesarean Section and Pregnancy
Introduction
Maternal Physiology
Cardiorespiratory System
Cardiovascular changes
Increased oxygen consumption
Increased cardiac output
Decreased vascular tone
Decreased arterial blood pressure
Increased stroke volume
Increased heart rate
Increased blood volume
Increased red blood cell mass
Decreased packed cell volume
Respiratory changes
Increased tidal volume
Increased respiratory rate
Increased minute ventilation
Decreased functional residual capacity
Gastrointestinal changes
Increased intragastric pressure
Decreased lower esophageal sphincter pressure
Decreased GI motility
Increased gastrin production
Central nervous system changes
Increased endorphins
Gastrointestinal System
Central Nervous System (CNS)
Placental Drug Transfer
Opioid and Nonsteroidal Anti‐Inflammatory Drug Effects on Maternal and Fetal Physiology
Opioids
Nonsteroidal Anti‐Inflammatory Drugs (NSAIDs)
Patient Management for Cesarean Section
Preoperative Considerations
Stay updated, free articles. Join our Telegram channel

Full access? Get Clinical Tree
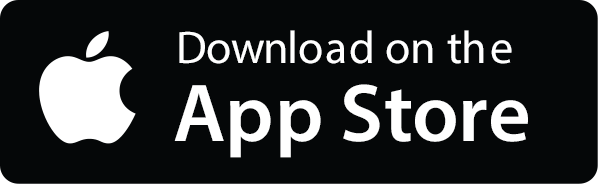
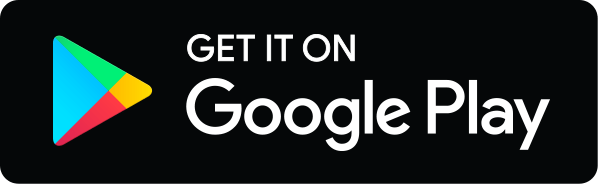