Species
Anatomy and pretreatment
Optimal preservation methods
Black-footed ferret (Mustela nigripes)
Highly compacted seminiferous tubules
1 h enzymatic digestion
Slow freezing in straws
Cheetah (Acinonyx jubatus)
Seminiferous tubules can be easily separated
30 min enzymatic digestion
Rapid freezing in straws
Clouded leopard (Neofelis nebulosa)
Fishing cat (Prionailurus viverrinus)
Siberian tiger (Panthera tigris altaica)
Dama gazelle (Nanger dama)
Highly compacted seminiferous tubules
1 h enzymatic digestion
Slow freezing in cryovials
Based on success with cryopreservation of reproductive tissues from mice and non-human primates used as model species over the past 10 years (Comizzoli et al. 2010), encouraging progress is being made towards producing viable antral ovarian follicles, especially for the cat and the dog, a topic addressed in detail by Songsasen et al. (2011, 2012).Working with partners at other zoological institutions, ovaries are being shipped at 4 °C to our core laboratory, processed by cutting them into 1–2 mm3 pieces, equilibrated in cryoprotectant and then preserved by comparing of methods. So far, we have clearly demonstrated the value of vitrification (e.g., in 15 % ethylene glycol + 15 % DMSO + 0.5 M sucrose) compared to slow freezing methods for primordial follicles enclosed in the ovarian cortex from prepubertal and adult felids and several ungulate species (Comizzoli et al. 2010, 2012). Optimal techniques now are being used to routinely bank ovarian tissue from various species, including the black-footed ferret, cheetah (Acinonyx jubatus), clouded leopard (Neofelis nebulosa), Eld’s deer (Rucervus eldii), scimitar-horned oryx (Oryx dammah), tufted deer (Elaphodus cephalophus) and Przewalski horse (Comizzoli et al. 2010, 2012). As demonstrated for testicular tissue, preservation of small ovarian biopsies is preferred over the whole gonad to increase the number of frozen samples available from a given individual. The post-warming viability is assessed using different criteria after 2 days of in vitro culture (retention of tissue structure and cell communications, >50 % of the cell viability, proliferation of the granulosa as well as the stroma cells, <10 % apoptosis). As mentioned for male gonads, tissue structure characteristics are influencing the pre-treatment and the cryopreservation method (Table 14.2) but no differences between prepubertal (high follicle density) and adult ovarian tissues can usually be observed. We have consistently observed that germ cells tend to be more cryoresistant than the somatic/stroma cells. Also, the viability and the proliferation of the stroma cells surrounding the early follicles are considered as a critical viability indicator for the subsequent follicular growth. High survival of slow frozen-thawed ovarian tissue from some felids or marsupial species (wombat; Vombatus ursinus) also has been demonstrated on the basis of cell integrity after culture and grafting success (Jewgenow et al. 2011; Wiedemann et al. 2013; Paris et al. 2004; Cleary et al. 2004). Of course, the ability to grow these early stage follicles and their oocytes in vitro to achieve full maturation and fertilization would be more convenient but largely remain unknown, even for common livestock and laboratory species. This requires examining a host of micro-environmental factors from the hormonal support and oxygen concentration needed to the ability to eliminate wastes in follicular culture systems that will require up to 6 months to produce viable oocytes (Songsasen et al. 2011, 2012).
Table 14.2
Specificities in ovarian tissue anatomy and cryopreservation methods
Species | Anatomy | Optimal preservation methods |
---|---|---|
Black footed-ferret (Mustela nigripes) | Hard tissue | Rapid freezing of whole ovary in cryovial |
Cheetah (Acinonyx jubatus) | Soft tissue | Rapid freezing of 1–2 mm biopsies in cryovials |
Clouded leopard (Neofelis nebulosa) | ||
Florida panther (Puma concolor) | ||
Sumatran Tiger (Panthera tigris sumatrae) | ||
Maned wolf (Chrysocyon brachyurus) | Hard tissue | Rapid freezing of 1–2 mm biopsies in cryovials |
The preservation of gonadal tissue also is relevant for non-mammal species as indicated by a few studies. For species with eggs containing yolk, there has been interest in cryopreserving primordial germ cells as demonstrated in the rainbow trout (Oncorhynchus mykiss). These cells can be frozen in 1.8 M ethylene glycol, thawed and transplanted into the peritoneal cavity of allogenic trout hatchlings where they differentiated into mature spermatozoa and eggs having the genetic constitution of the original donor (Kobayashi et al. 2007). Production of donor-derived offspring also has been reported after transplantation of vitrified ovarian tissue in Japanese quail (Coturnix japonica) using the same vitrification solutions as in mammalian species (15 % ethylene glycol + 15 % DMSO + 0.5 M sucrose) (Liu et al. 2010). In that set of studies, vitrification again appears to have been more successful than slow-freezing for bird ovarian tissues. Transplantation of whole frozen ovaries as a means of capturing important genetic quality has been undertaken successfully with silkworms (Mochida et al. 2003; Banno et al. 2013). These authors estimated that there are about 2,000 strains of silkworms in Japan and that these are currently maintained as live cultures. The earlier report used a cryopreservation protocol, which was based on the use of 1.5 M DMSO and suspending the vials containing ovaries in liquid nitrogen vapor for 30 min, resulted in about 22 % of transplanted moths producing fertilized eggs. The more recent study used a slower cooling rate (1 °C/min to −80 °C followed by plunging in liquid nitrogen) and reported improved results (about 70 % of transplanted moths laid fertilized eggs).
5 Emerging Preservation Approaches to Address Current Limitations
5.1 Novel Sources of Germplasm
Stem cell technologies are promising as methods for producing gametes from embryonic stem cells, spermatogonial progenitors, or from differentiated cells. Characterization, isolation, and transfer of spermatogonial stem cells have been attempted in the cat and dog with mixed results (Travis et al. 2009). In brief, this has involved isolating the spermatogonial stem cells followed by transfer into a germ-cell depleted (via radiation) host. On occasion, it has been possible to recover ~20 % of mature sperm cells derived from the donor (Travis et al. 2009). Others have transplanted germ cells from a wild felid (ocelot) into the domestic cat to produce spermatozoa successfully from the donor (Silva et al. 2012). Recent studies on ovarian stem cells in various mammal species could also hold some promises for the production of gametes from endangered species (Dunlop et al. 2013).
The induced Pluripotent Stem (iPS) cell concept also is timely because of the possibility to optimize the use of Genome Resource Banks that include cultured somatic cells like fibroblasts (see Chap. 16 by Mastromonaco et al.). The iPS cells (in appropriate culture conditions) could provide a self-renewing, inexhaustible resource of material from wildlife species (Ben-Nun et al. 2011). Eventually, it will probably be more efficient to differentiate embryonic stem cells or iPS cells in vitro for this purpose, the latter being accomplished recently for the snow leopard (Panthera uncia) (Verma et al. 2012). The striking potential of these strategies also has been demonstrated in the mouse where in vitro-differentiated embryonic stem cells have given rise to sperm-like cells (Nayernia et al. 2006) or oocyte-like cells derived from newborn mouse skin (Dyce et al. 2011).
The limited success achieved with preserving fish oocytes and embryos has stimulated some novel approaches to the problem. One of the most interesting current strategies involves the vitrification of whole embryos at the 22–28 somite stage; this does not result in live post-thaw embryos but the primordial germ cells (PGC) survive the procedure. The PGCs, which are green fluorescent protein-labeled prior to vitrification, are dissected out of the embryos and transplanted into host blastulae from the same or a different species. When the host embryo develops the theoretical outcome is that it will produce gametes that are genetically derived from the original vitrified embryo (Fig. 14.1). One example of this procedure involved transferring common carp (Cyprinus carpio) PGCs into goldfish (Carassius auratus) embryos (Kawakami et al. 2012). A variant of this method involved isolating and vitrifying loach (Misgurnus anguillicaudatus) PGCs, labeling them with a fluorescent protein produced in zebrafish, and transplanting them into fresh embryos (Yasui et al. 2011; Inoue et al. 2012). The transplanted PGCs retained their ability to migrate within the embryo and colonize the genital ridge, an important outcome showing that this method has a realistic chance of resulting in normal sexual differentiation and gamete production. A different and equally ingenious approach to the problem of genome preservation in fish is based on the recovery of Type A spermatogonia (ASG) from slowly frozen rainbow trout (Oncorhynchus mykiss) testes (Lee et al. 2013) and their subsequent transfer into the peritoneal cavity of sterile triploid hatchlings of the same species. In this study nearly half of the triploid recipients produced functional eggs or spermatozoa derived from the frozen ASGs. Fertilization of these gametes resulted in the successful production of normal, frozen ASG-derived offspring. From the standpoint of genome conservation one of the most interesting aspects of this study was that the ASGs were derived from testes that had been kept frozen for up to 939 days without significant loss of ASG viability or lowered performance of the derived gametes. The authors state that the isolation and transfer of ASGs is very straightforward and practical; however, the ASGs in this study had been labeled with green fluorescent protein prior to isolation and identification of live ASGs was therefore relatively easy. If this method is to be useful for endangered fish species a new approach to the identification of ASGs will be required (Fig. 14.1).
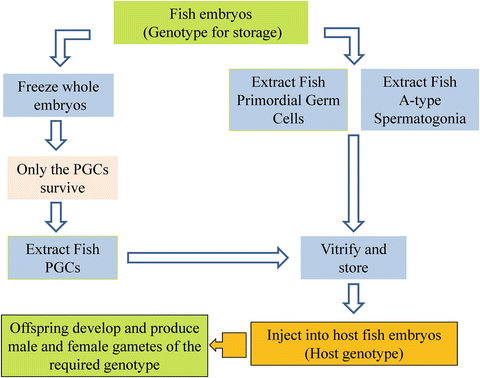
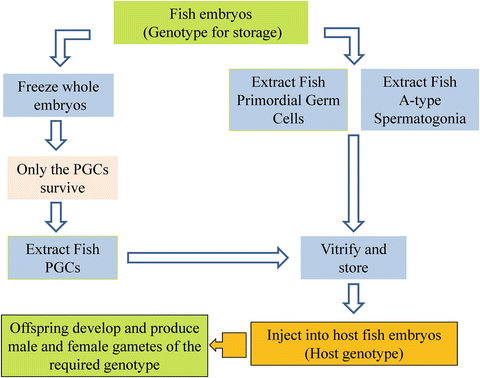
Fig. 14.1
Novel approaches in germplasm production and preservation in fish
The interest in PGC transplantation combined with the current topicality of research into epigenetics has raised awareness that freezing and thawing might, per se, induce epigenetic changes in the materials being preserved. In fact, evidence that this might be the case has recently been published by Riesco and Robles (2013) who studied the cryopreservation of zebrafish genital ridges; these are regarded as useful candidates for genome banking because they contain PGCs. The study detected the cryopreservation-induced downregulation of several zebrafish mRNA transcripts, and the upregulation of two heat shock proteins, Hsp70 and Hsp90. Epigenetic gene downregulation is normally attributed to hypermethylation of gene promoter regions, and the authors found that one specific promoter was indeed hypermethylated as a result of cryopreservation. This study confirmed what had been suspected for several years, namely that cryopreservation methods might have unforeseen epigenetic outcomes that could lead to altered phenotypes and disease. In fact, cryopreservation is not alone in being implicated in the induction of epigenetic changes. Recent concern has been expressed about the negative epigenetic impacts of culture media used for human embryos during assisted reproduction techniques (Nelissen et al. 2004). Similar effects, later attributed to the presence of growth factors in media containing bovine serum albumin (BSA) (Thompson et al. 1998) led to the birth of overweight calves following IVF and embryo transfer, but these effects were eliminated by the expedient of treating the BSA with charcoal. Subtle effects associated with assisted reproductive technologies can occur whenever gametes and embryos are being manipulated (for review, see Yamauchi et al. 2011) and it is clear that this will be an important research area in the future.
5.2 Alternative Preservation Methods at Low Temperatures
Directional freezing is based on a simple thermodynamic principle in which ice crystals are precisely controlled through the sample by regulating the velocity of the sample movement through the predetermined temperature gradient. Directional freezing permits a precise and uniform cooling rate in both small and large volume samples. Directional freezing has been used for slow and rapid freezing, as well as for vitrification of oocytes and embryos using the minimum drop size technique. Sperm samples from a wide range of domestic and wild animals have been successfully cryopreserved using the directional freezing method. The method also has enabled, for the first time, successful freezing of a whole ovary and freeze-drying of mammalian cells followed by thawing and transplantation and rehydration, respectively (Arav and Natan 2012).Post-thaw results showed that within the same sperm type in marine mammals, directional freezing was superior to conventional techniques for maintaining motility and in immediate post-thaw viabilities (O’Brien and Robeck 2006). Another comparative study was carried out on spermatozoa from killer whales, contrasting conventional freezing (using straws) and directional freezing (using hollow tubes) (Robeck et al. 2011). The post-thaw results showed that directional freezing also was superior to conventional freezing in all parameters of motility and viability in gazelle (Gazella gazelle) semen (Saragusty et al. 2006), European brown hare (Lepus europaeus, Hildebrandt et al. 2009), or rhinoceros (Ceratotherium simum simum, Hermes et al. 2009).
Ultra-rapid freezing and vitrification still are regarded by many as ‘novel’, despite Rall and Fahy’s pioneering report more than 25 years ago on its usefulness for preserving mouse embryos (Rall and Fahy 1985). We continue to be enthusiastic about vitrification because of its relative simplicity, low cost and ‘field-friendliness’ (i.e., the ability to vitrify biomaterials, even in harsh, remote environments using only a liquid nitrogen dry shipper). Interestingly, compared to studies of embryos or oocytes, there continues to be few reports on the efficacy of ultra-rapid freezing, or vitrification, of spermatozoa. However, encouraging results recently have been reported for human (Isachenko et al. 2011) and dog (Kim et al. 2012) spermatozoa. In the latter case, the gametes were exposed to 5 % glycerol and freezing vapors for <1 min before plunging into liquid nitrogen; >50 % of spermatozoa were motile after thawing. Despite its great potential, this approach has not yet been explored in wild species.
Besides the freezing methods, the addition of heat shock protein HSPA8 to cryoprotectant media can improve the survival of spermatozoa post-thawing as demonstrated in the brown bear (Ursus arctos) (Alvarez-Rodriguez, et al. 2013). There also is a trend towards freeze-preservation in lower cryoprotectant concentrations, which appears especially important for dog spermatozoa and cat oocytes that are susceptible to cryoprotectant toxicity (Comizzoli et al. 2012). Another alternative explored in our laboratory is the effectiveness of vitrification solutions that rely only on non-permeating (non-toxic) cryoprotectants, including natural sugars such as sucrose or trehalose. In the case of cat oocytes, we have observed >80 % survival after vitrification in saturated trehalose solutions (Comizzoli and Wildt 2012a).
5.3 Novel Preservation Methods at Supra-zero Temperatures
Although isolated cells and tissues can be successfully vitrified and warmed without detrimental formation of ice crystals, the challenge remains that low temperature storage can trigger injury to DNA, membranes, and cell junctions (Yavin and Arav 2007). Dehydration by air-, evaporative-, and vacuum-drying, followed by storage at room temperature is therefore an appealing option for preserving germplasm, because desiccation is similar to natural approaches used by certain small organisms to suspend their life cycle. For instance, tardigrades are protostomal animals well known for their capability of surviving extreme conditions by undergoing anhydrobiosis at ambient temperature for extended periods (Crowe et al. 2002). This phenomenon is possible due to an innate ability to accumulate natural sugars (including the disaccharide trehalose) intracellularly to preserve membrane lipid bilayers and proteins (Crowe et al. 2002, 2005). For this reason, our laboratory is exploring alternative opportunities for preserving carnivore spermatozoa via desiccation in trehalose and storage at supra-zero temperature (Comizzoli and Wildt 2012a). The advantages of both include much simpler sample processing and transport of genetic material and, most impressively, no need for liquid nitrogen. In theory, the latter could markedly reduce the costs and complexity of biomaterials storage. However, results also have revealed a significant loss in sperm motility and the potential of compromised centrosomal function post-rehydration. For example, we observed poor sperm aster formation after injecting dehydrated (at ambient temperature in trehalose) cat spermatozoa into conspecific oocytes (Comizzoli and Wildt 2012a). Centrosomal dysfunction post-freeze drying has been less apparent for nonhuman primate and bull spermatozoa, although rhesus monkey spermatozoa desiccated in trehalose are known to lose fertilizing capacity (Comizzoli and Wildt 2012b). Results from preliminary studies of freeze-drying canine spermatozoa have revealed pronuclear formation after injection into mouse oocytes (Watanabe et al. 2009). Also encouraging are the recent findings of Ringleb et al. (2011) who found early (albeit limited) embryo development after injecting freeze-dried cat spermatozoa into conspecific oocytes. Finally, it is worth noting that desiccation likely has excellent potential for preserving the maternal genome. For example, our laboratory has determined that GVs from cat oocytes that are artificially compacted (with histone deacetylation enhancers), air-dried, and then rehydrated, can resume meiosis after injection into a fresh (enucleated) cytoplast (Graves-Herring et al. 2013). Thus, it may be possible to use this GV rescue approach to salvage the maternal genome from individuals who die early or late in life before reproducing, or who are experiencing cytoplasmic deficits in the oocyte or follicular anomalies. It also has been determined that the chromatin of the cat GV withstands artificial compaction for subsequent injection to reconstruct a viable oocyte, all without encountering the need for the usual complex membrane electrofusion (Graves-Herring et al. 2013). This approach, never reported for other species, may well evolve into a simple, inexpensive, and biologically viable means of storing the female genome (without the cytoplasm) of carnivores as well as other taxa (Holt 2013).
Preservation in a liquid environment at supra-zero or ambient temperatures also is an emerging area in cell or tissue preservation. As an alternate to cat sperm storage in classical extenders at cold temperature (Pope et al. 2006), we have effectively preserved cooled (4 °C) cat spermatozoa for up to 2 weeks in a 2 M trehalose solution while retaining DNA integrity and centrosomal structure (presence of centrin) as well as function (sperm aster formation) (Comizzoli and Wildt, 2012b). Recently, encouraging results also have been obtained in porcine oocytes that were able to retain developmental competence after storage for several days at ambient temperature (Yang et al. 2010). We now are exploring the same strategy for gonadal tissues.
It appears that harmonization of protocols at supra-zero temperatures would be easier since there are no issues related to species-specific cryo-sensitivity. However, in the search for these new and simple preservation protocols, it is critical to thoroughly verify the integrity of the DNA sequence and the multiple epigenetic factors regulating the functionality of the genome. It is expected that newly available tools such as Next Generation Sequencing and other ‘omics’ in association with bioinformatics will help to accurately control the quality of the germplasm preserved with these new approaches.
5.4 Bridges with Human Fertility Preservation
More interactions between human and animal cryobiology are needed to optimize fertility preservation (Comizzoli et al. 2010). Indeed, the next steps in human and animal fertility preservation are dictated by similar needs for (1) more options in case of complex fertility issues (2) minimal cost, field-friendly methods when lack of resources and limited access to freezing equipment or liquid nitrogen and (3) customized/universal solutions since variations in cryo-sensitivity within animal populations are similar to humans. We argue that human reproductive specialists also could well take advantage of new fundamental knowledge on biological insights from studies of far-from-traditional animal species. Translational fertility preservation could be ensured by promoting more interaction among stakeholders in all areas—whether human, livestock, laboratory animal or wildlife-oriented. For example, there could be significant benefits from the establishment of a fertility preservation network, with benefits ranging from active communication for sharing critical (or simply interesting) information to opportunities for direct collaboration.
As already identified in human reproductive medicine, critical needs for the future regardless of our ability to find ‘more-forgiving’ technologies are to have wider application across taxonomic groups too. However, there will always be the need for basic, species specific data. For example, we still are going to need to know when an animal cycles, when it ovulates, how many sperm cells it produces, etc. So the shift may come in the timing of when the fundamental information is required. It may become less important before a sample is preserved—but it will likely still be crucial to when the sample is used to produce an offspring (from gamete reconstruction or micro-injection to in vitro culture to preparation of a female for AI or ET).
6 Conclusions
Most contemporary germplasm preservation research conducted has been concentrated on the cryopreservation of spermatozoa in diverse wild species. There are few studies on oocytes and gonadal tissues (Fig. 14.2). The overall goal needs to be creating the ability to preserve any germplasm from a valuable animal of any age or reproductive state using reasonably simple, cost-effective techniques. Clearly, there are still vast needs in basic cryobiological studies for diverse species, especially fundamental biophysical traits as well as comparative evaluations of permeating (e.g., DMSO, DMA, propylene glycol) versus non-permeating (e.g. raffinose, trehalose) cryoprotectants as well as freeze/thaw rates. In some cases, progress can be enhanced markedly by relying on data already available in domesticated counterparts (e.g., cattle for antelope, dog for wild canids and cat for wild felids). However, even this approach can fail to overcome within-family physiological variations among species (Wildt et al. 2010). And relying on a domesticated counterpart does not assist the thousands of absolutely unique species (e.g., elephant, killer whale, bats, monotremes, seahorses, Monarch butterfly, among hundreds of others) for which no closely related research model exists. Inter-species (mammals vs. non-mammals, carnivores vs. ungulates) as well as inter-individual variations or sometimes commonalities also can be remarkable. More cryobiological studies will also help to develop customized treatments for some individuals or lead to universal freezing methods valid for a vast array of species. Progress can only be made if we continue to explore basic gamete and gonad biology to identify appropriate quality criteria before and after freezing. There also is a need to be more expansive in our thinking about the priorities in cryobiology and reproductive science, and this certainly includes species that are not mammals. The future is exciting because such efforts will continue to demonstrate the amazing reproductive diversity that already exists as well as produce knowledge that actually will be practical—helping to sustain viable populations and even avoid extinctions. Unlike as little as 20 years ago, such statements are no longer hyperbole. The recovery of species as diverse as the whooping crane, black-footed ferret and giant panda owe at least partial credit to modern reproductive science and tools in fertility preservation and assisted breeding.